Abstract
1. The effects of palmitoyl-DL-carnitine (0.01 to 1 mM) on whole cell voltage-activated calcium channel currents carried by calcium or barium and Ca(2+)-activated chloride currents were studied in cultured neurones from rat dorsal root ganglia. 2. Palmitoyl-DL-carnitine applied to the extracellular environment or intracellularly via the patch solution reduced Ca2+ currents activated over a wide voltage range from a holding potential of -90 mV. Inhibition of high voltage activated Ca2+ channel currents was dependent on intracellular Ca2+ buffering and was reduced by increasing the EGTA concentration from 2 to 10 mM in the patch solution. Barium currents were significantly less sensitive to palmitoyl-DL-carnitine than Ca2+ currents. 3. The amplitude of Ca(2+)-activated Cl- tail currents was reduced by palmitoyl-DL-carnitine. However, the duration of these Cl- currents was greatly prolonged by palmitoyl-DL-carnitine, suggesting slower removal of free Ca2+ from the cytoplasm following Ca2+ entry through voltage-activated channels. 4. Palmitoyl-DL-carnitine evoked Ca(2+)-dependent inward currents which could be promoted by activation of the residual voltage-activated Ca2+ currents and attenuated by intracellular application of EGTA. 5. We conclude that palmitoyl-DL-carnitine reduced the efficiency of intracellular Ca2+ handling in cultured dorsal root ganglion neurones and resulted in enhancement of Ca(2+)-dependent events including inactivation of voltage-activated Ca2+ currents. The activation of inward currents by palmitolyl-DL-carnitine may involve Ca(2+)-induced Ca2+ release from intracellular stores, or direct interaction of palmitoyl-DL-carnitine with Ca2+ stores.
Full text
PDF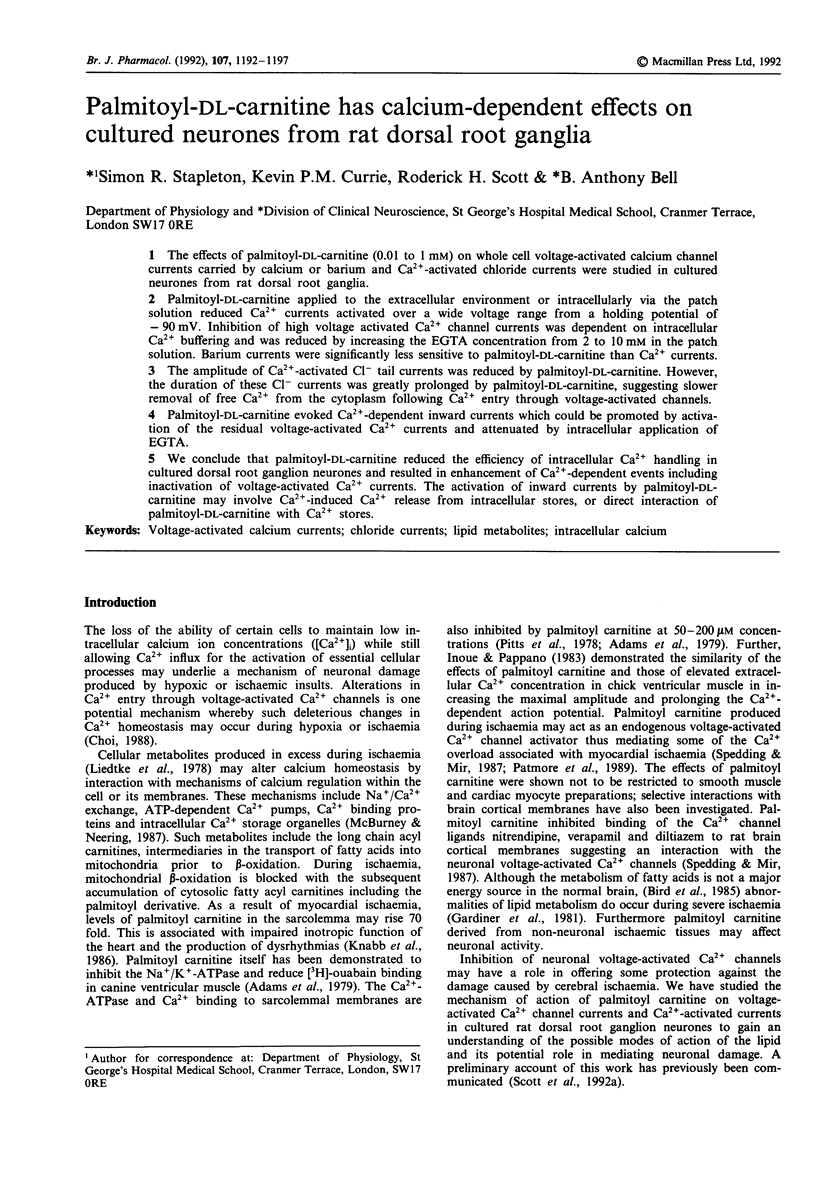
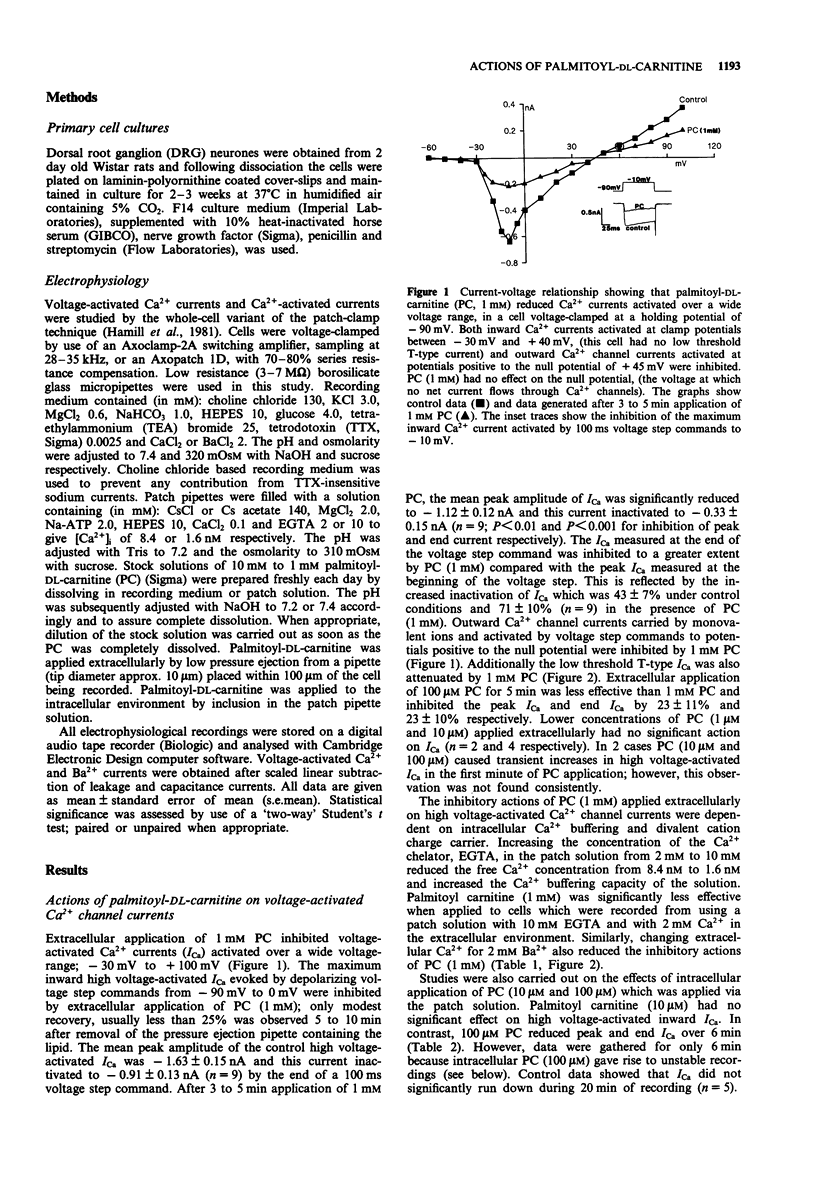
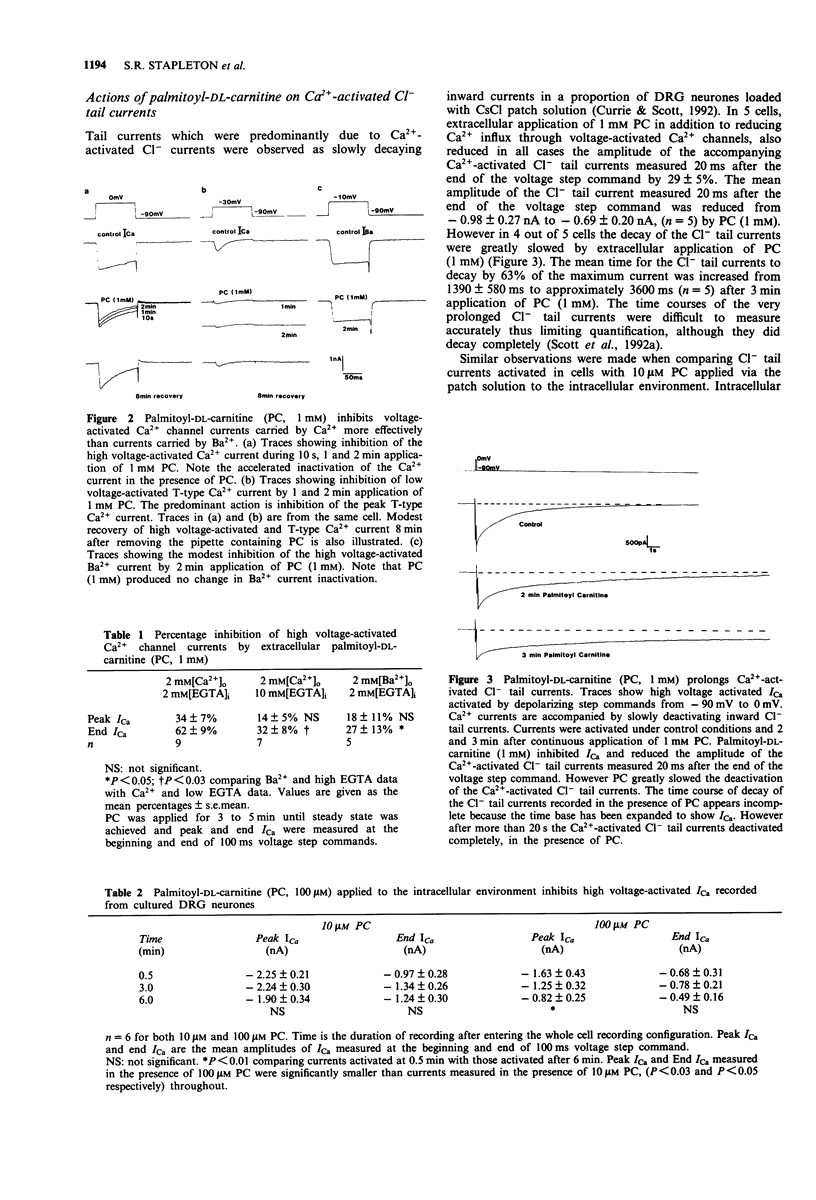
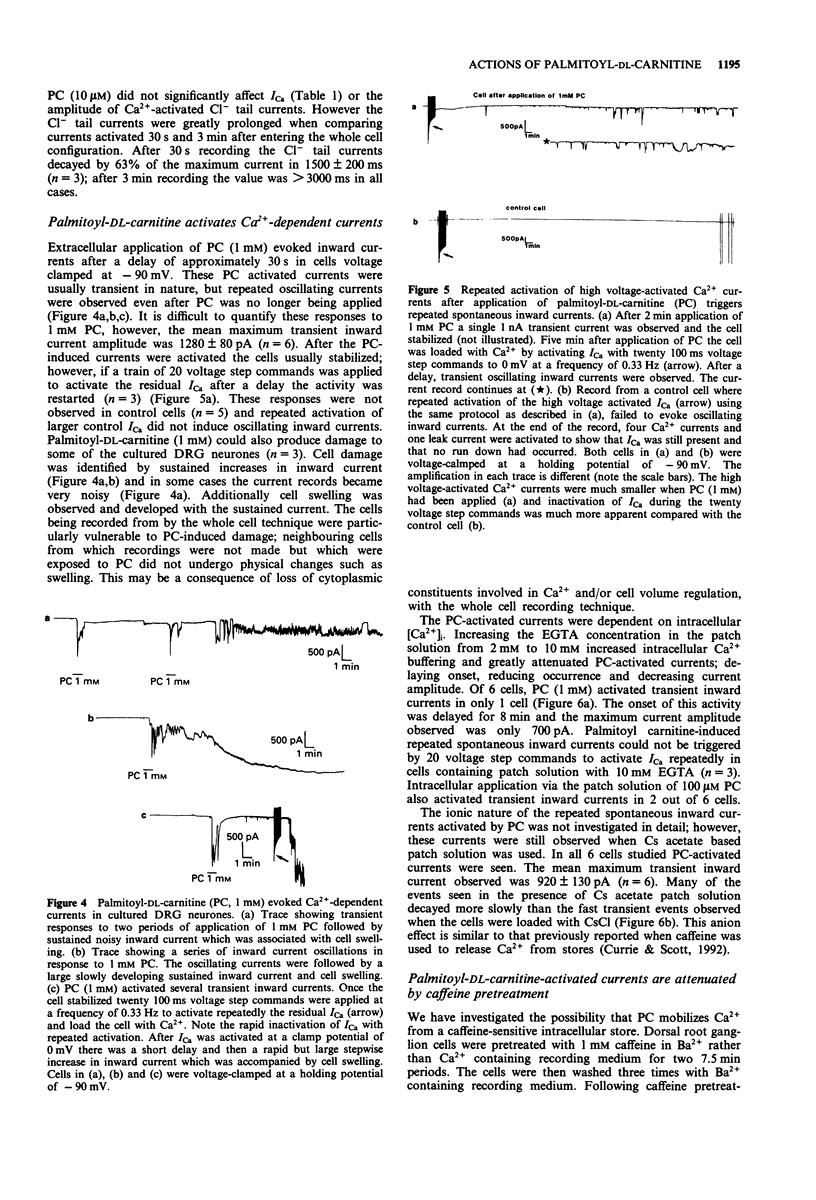
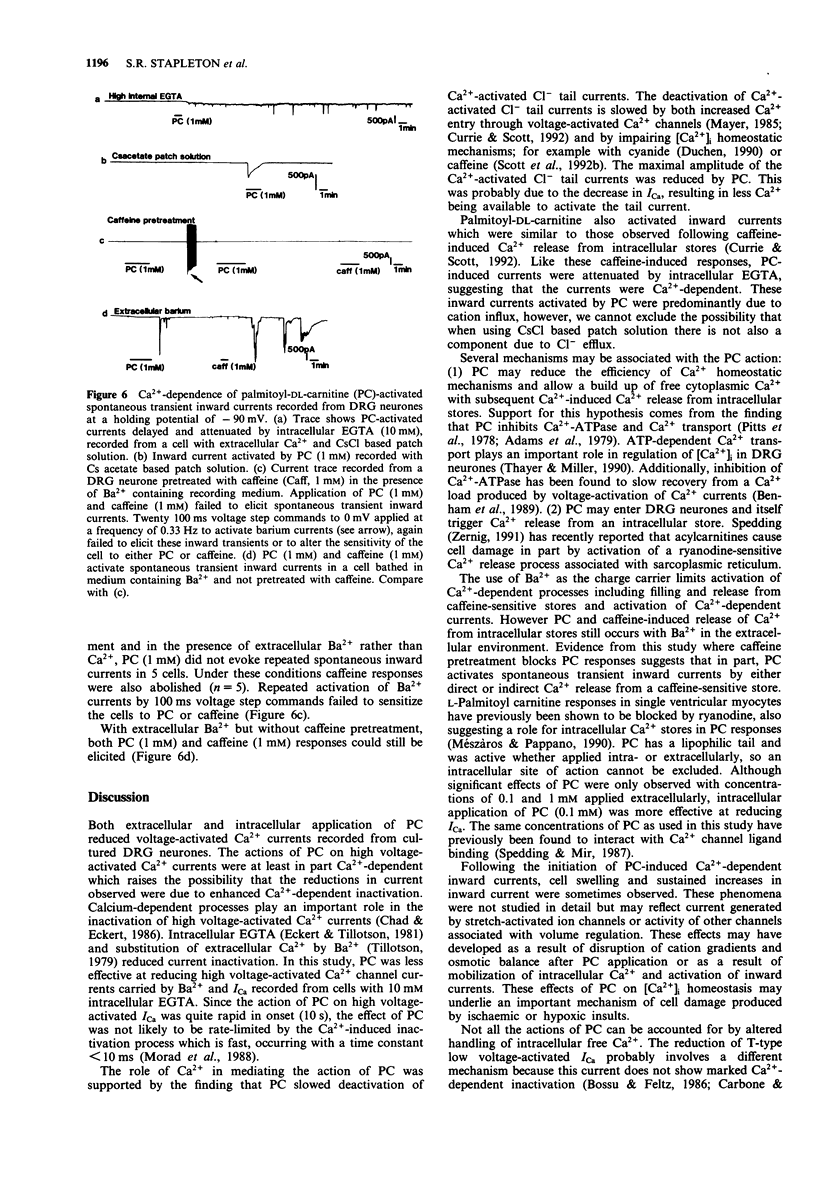
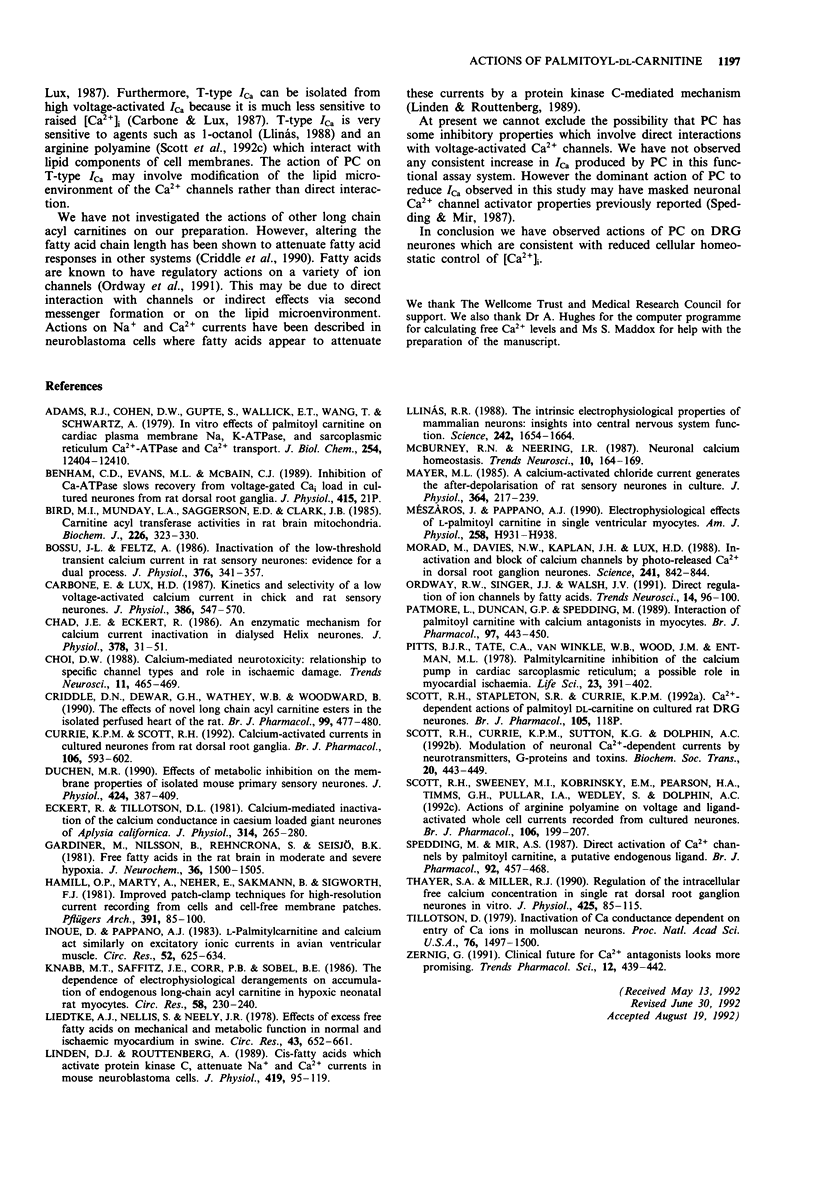
Selected References
These references are in PubMed. This may not be the complete list of references from this article.
- Adams R. J., Cohen D. W., Gupte S., Johnson J. D., Wallick E. T., Wang T., Schwartz A. In vitro effects of palmitylcarnitine on cardiac plasma membrane Na,K-ATPase, and sarcoplasmic reticulum Ca2+-ATPase and Ca2+ transport. J Biol Chem. 1979 Dec 25;254(24):12404–12410. [PubMed] [Google Scholar]
- Bird M. I., Munday L. A., Saggerson E. D., Clark J. B. Carnitine acyltransferase activities in rat brain mitochondria. Bimodal distribution, kinetic constants, regulation by malonyl-CoA and developmental pattern. Biochem J. 1985 Feb 15;226(1):323–330. doi: 10.1042/bj2260323. [DOI] [PMC free article] [PubMed] [Google Scholar]
- Bossu J. L., Feltz A. Inactivation of the low-threshold transient calcium current in rat sensory neurones: evidence for a dual process. J Physiol. 1986 Jul;376:341–357. doi: 10.1113/jphysiol.1986.sp016157. [DOI] [PMC free article] [PubMed] [Google Scholar]
- Carbone E., Lux H. D. Kinetics and selectivity of a low-voltage-activated calcium current in chick and rat sensory neurones. J Physiol. 1987 May;386:547–570. doi: 10.1113/jphysiol.1987.sp016551. [DOI] [PMC free article] [PubMed] [Google Scholar]
- Chad J. E., Eckert R. An enzymatic mechanism for calcium current inactivation in dialysed Helix neurones. J Physiol. 1986 Sep;378:31–51. doi: 10.1113/jphysiol.1986.sp016206. [DOI] [PMC free article] [PubMed] [Google Scholar]
- Choi D. W. Calcium-mediated neurotoxicity: relationship to specific channel types and role in ischemic damage. Trends Neurosci. 1988 Oct;11(10):465–469. doi: 10.1016/0166-2236(88)90200-7. [DOI] [PubMed] [Google Scholar]
- Criddle D. N., Dewar G. H., Wathey W. B., Woodward B. The effects of novel vasodilator long chain acyl carnitine esters in the isolated perfused heart of the rat. Br J Pharmacol. 1990 Mar;99(3):477–480. doi: 10.1111/j.1476-5381.1990.tb12953.x. [DOI] [PMC free article] [PubMed] [Google Scholar]
- Currie K. P., Scott R. H. Calcium-activated currents in cultured neurones from rat dorsal root ganglia. Br J Pharmacol. 1992 Jul;106(3):593–602. doi: 10.1111/j.1476-5381.1992.tb14381.x. [DOI] [PMC free article] [PubMed] [Google Scholar]
- Duchen M. R. Effects of metabolic inhibition on the membrane properties of isolated mouse primary sensory neurones. J Physiol. 1990 May;424:387–409. doi: 10.1113/jphysiol.1990.sp018073. [DOI] [PMC free article] [PubMed] [Google Scholar]
- Eckert R., Tillotson D. L. Calcium-mediated inactivation of the calcium conductance in caesium-loaded giant neurones of Aplysia californica. J Physiol. 1981 May;314:265–280. doi: 10.1113/jphysiol.1981.sp013706. [DOI] [PMC free article] [PubMed] [Google Scholar]
- Gardiner M., Nilsson B., Rehncrona S., Siesjö B. K. Free fatty acids in the rat brain in moderate and severe hypoxia. J Neurochem. 1981 Apr;36(4):1500–1505. doi: 10.1111/j.1471-4159.1981.tb00592.x. [DOI] [PubMed] [Google Scholar]
- Hamill O. P., Marty A., Neher E., Sakmann B., Sigworth F. J. Improved patch-clamp techniques for high-resolution current recording from cells and cell-free membrane patches. Pflugers Arch. 1981 Aug;391(2):85–100. doi: 10.1007/BF00656997. [DOI] [PubMed] [Google Scholar]
- Inoue D., Pappano A. J. L-palmitylcarnitine and calcium ions act similarly on excitatory ionic currents in avian ventricular muscle. Circ Res. 1983 Jun;52(6):625–634. doi: 10.1161/01.res.52.6.625. [DOI] [PubMed] [Google Scholar]
- Knabb M. T., Saffitz J. E., Corr P. B., Sobel B. E. The dependence of electrophysiological derangements on accumulation of endogenous long-chain acyl carnitine in hypoxic neonatal rat myocytes. Circ Res. 1986 Feb;58(2):230–240. doi: 10.1161/01.res.58.2.230. [DOI] [PubMed] [Google Scholar]
- Liedtke A. J., Nellis S., Neely J. R. Effects of excess free fatty acids on mechanical and metabolic function in normal and ischemic myocardium in swine. Circ Res. 1978 Oct;43(4):652–661. doi: 10.1161/01.res.43.4.652. [DOI] [PubMed] [Google Scholar]
- Linden D. J., Routtenberg A. cis-Fatty acids, which activate protein kinase C, attenuate Na+ and Ca2+ currents in mouse neuroblastoma cells. J Physiol. 1989 Dec;419:95–119. doi: 10.1113/jphysiol.1989.sp017863. [DOI] [PMC free article] [PubMed] [Google Scholar]
- Llinás R. R. The intrinsic electrophysiological properties of mammalian neurons: insights into central nervous system function. Science. 1988 Dec 23;242(4886):1654–1664. doi: 10.1126/science.3059497. [DOI] [PubMed] [Google Scholar]
- Mayer M. L. A calcium-activated chloride current generates the after-depolarization of rat sensory neurones in culture. J Physiol. 1985 Jul;364:217–239. doi: 10.1113/jphysiol.1985.sp015740. [DOI] [PMC free article] [PubMed] [Google Scholar]
- Morad M., Davies N. W., Kaplan J. H., Lux H. D. Inactivation and block of calcium channels by photo-released Ca2+ in dorsal root ganglion neurons. Science. 1988 Aug 12;241(4867):842–844. doi: 10.1126/science.2457253. [DOI] [PubMed] [Google Scholar]
- Mészàros J., Pappano A. J. Electrophysiological effects of L-palmitoylcarnitine in single ventricular myocytes. Am J Physiol. 1990 Apr;258(4 Pt 2):H931–H938. doi: 10.1152/ajpheart.1990.258.4.H931. [DOI] [PubMed] [Google Scholar]
- Ordway R. W., Singer J. J., Walsh J. V., Jr Direct regulation of ion channels by fatty acids. Trends Neurosci. 1991 Mar;14(3):96–100. doi: 10.1016/0166-2236(91)90069-7. [DOI] [PubMed] [Google Scholar]
- Patmore L., Duncan G. P., Spedding M. Interaction of palmitoyl carnitine with calcium antagonists in myocytes. Br J Pharmacol. 1989 Jun;97(2):443–450. doi: 10.1111/j.1476-5381.1989.tb11971.x. [DOI] [PMC free article] [PubMed] [Google Scholar]
- Pitts B. J., Tate C. A., Van Winkle W. B., Wood J. M., Entman M. L. Palmitylcarnitine inhibition of the calcium pump in cardiac sarcoplasmic reticulum: a possible role in myocardial ischemia. Life Sci. 1978 Jul 24;23(4):391–401. doi: 10.1016/0024-3205(78)90025-5. [DOI] [PubMed] [Google Scholar]
- Scott R. H., Currie K. P., Sutton K. G., Dolphin A. C. Modulation of neuronal Ca(2+)-dependent currents by neurotransmitters, G-proteins and toxins. Biochem Soc Trans. 1992 May;20(2):443–449. doi: 10.1042/bst0200443. [DOI] [PubMed] [Google Scholar]
- Scott R. H., Sweeney M. I., Kobrinsky E. M., Pearson H. A., Timms G. H., Pullar I. A., Wedley S., Dolphin A. C. Actions of arginine polyamine on voltage and ligand-activated whole cell currents recorded from cultured neurones. Br J Pharmacol. 1992 May;106(1):199–207. doi: 10.1111/j.1476-5381.1992.tb14315.x. [DOI] [PMC free article] [PubMed] [Google Scholar]
- Spedding M., Mir A. K. Direct activation of Ca2+ channels by palmitoyl carnitine, a putative endogenous ligand. Br J Pharmacol. 1987 Oct;92(2):457–468. doi: 10.1111/j.1476-5381.1987.tb11343.x. [DOI] [PMC free article] [PubMed] [Google Scholar]
- Thayer S. A., Miller R. J. Regulation of the intracellular free calcium concentration in single rat dorsal root ganglion neurones in vitro. J Physiol. 1990 Jun;425:85–115. doi: 10.1113/jphysiol.1990.sp018094. [DOI] [PMC free article] [PubMed] [Google Scholar]
- Tillotson D. Inactivation of Ca conductance dependent on entry of Ca ions in molluscan neurons. Proc Natl Acad Sci U S A. 1979 Mar;76(3):1497–1500. doi: 10.1073/pnas.76.3.1497. [DOI] [PMC free article] [PubMed] [Google Scholar]
- Zernig G. Clinical future for Ca2+ antagonists looks more promising. Trends Pharmacol Sci. 1991 Dec;12(12):439–442. doi: 10.1016/0165-6147(91)90628-6. [DOI] [PubMed] [Google Scholar]