Abstract
1. The present work was designed to examine the interference of L-3,4-dihydroxyphenylalanine (L-DOPA) on the cell inward transport of L-5-hydroxytryptophan (L-5-HTP) and on its decarboxylation by aromatic L-amino acid decarboxylase (AAAD) in rat isolated renal tubules. 2. The accumulation of both L-5-HTP and L-DOPA in renal tubules was found to occur through non-saturable and saturable mechanisms. The kinetics of the saturable component L-5-HTP and L-DOPA uptake in renal tubules were as follows: L-5-HTP, Vmax = 24.9 +/- 4.5 nmol mg-1 protein h-1 and Km = 121 (95% confidence limits: 75, 193) microM (n = 5); L-DOPA, Vmax = 58.0 +/- 4.3 nmol mg-1 protein h-1 and Km = 135 (97, 188) microM (n = 5). When the saturation curve of L-5-HTP tubular uptake was performed in the presence of L-DOPA (250 microM), the maximal rate of accumulation of L-5-HTP in renal tubules was found to be markedly (P < 0.01) reduced (Vmax = 10.5 +/- 1.7 nmol mg-1 protein h-1, n = 4); this was accompanied by a significant (P < 0.05) increase in Km values (325 [199, 531] microM, n = 4). 3. L-DOPA (50 to 2000 microM) was found to produce a concentration-dependent decrease (38% to 91% reduction) in the tubular uptake of 5-HTP; the Ki value (in microM) of L-DOPA for inhibition of L-5-HTP uptake was found to be 29.1 (13.8, 61.5) (n = 6). 4. At the highest concentration tested the organic anion inhibitor, probenecid (10 microM) produced no significant (P = 0.09) changes in L-5-HTP and L-DOPA uptake (18% and 22% reduction, respectively). The organic cation inhibitor, cyanine 863 (1-ethyl-2-[1,4-dimethyl-2-phenyl-6-pyrimidinylidene)methyl]-quino linium) produced a potent inhibitory effect on the tubular uptake of L-5-HTP (Ki = 212 [35, 1289] nM, n = 8), being slightly less effective against L-DOPA uptake (Ki = 903 [584, 1396] nM, n = 5). The cyanine derivatives 1,1-diethyl-2,4-cyanine (decynium 24) and 1,1-diethyl-2,2-cyanine (decynium 22) potently inhibited the tubular uptake of both L-5-HTP (Ki = 100 [49, 204] and 120 [26, 561] nM, n = 4-6, respectively) and L-DOPA (Ki = 100 [40, 290] and 415 [157, 1094] nM, n = 5, respectively). 5. The Vmax and Km values for AAAD using L-DOPA as the substrate (Vmax = 479.9 +/- 74.0 nmol mg-1 protein h-1; Km = 2380 [1630, 3476] microM; n = 4) were both found to be significantly (P < 0.01) higher than those observed when using L-5-HTP (Vmax = 81.4 +/- 5.2 nmol mg-1 protein h-1, Km = 97 [87, 107] microM, n = 10). The addition of 5 mM L-DOPA to the incubation medium reduced by 30% (P < 0.02) the maximal rate of decarboxylation of L-5-HTP (Vmax = 56.7 +/- 3.1 nmol mg-1 protein h-1, n = 10) and resulted in a significant (P < 0.05) increase in Km values (249 [228, 270] microM, n = 10). 6. The results presented suggest that L-5-HTP and L-DOPA are using the same transporter (most probably, the organic cation transporter) in order to be taken up into renal tubular cells; L-DOPA exerts a competitive type of inhibition upon the tubular uptake and decarboxylation of L-5-HTP. The decrease in the formation of 5-HT as induced by L-DOPA may also depend on a decrease in the rate of its decarboxylation by AAAD.
Full text
PDF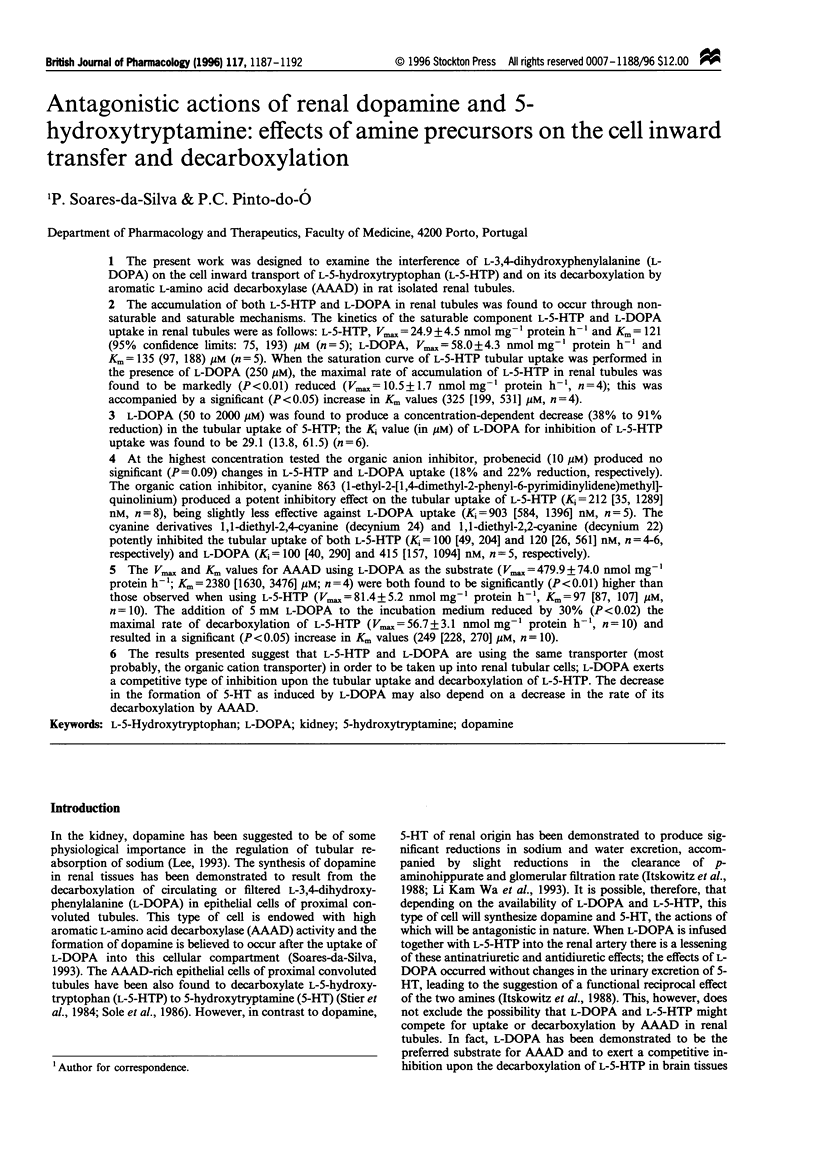
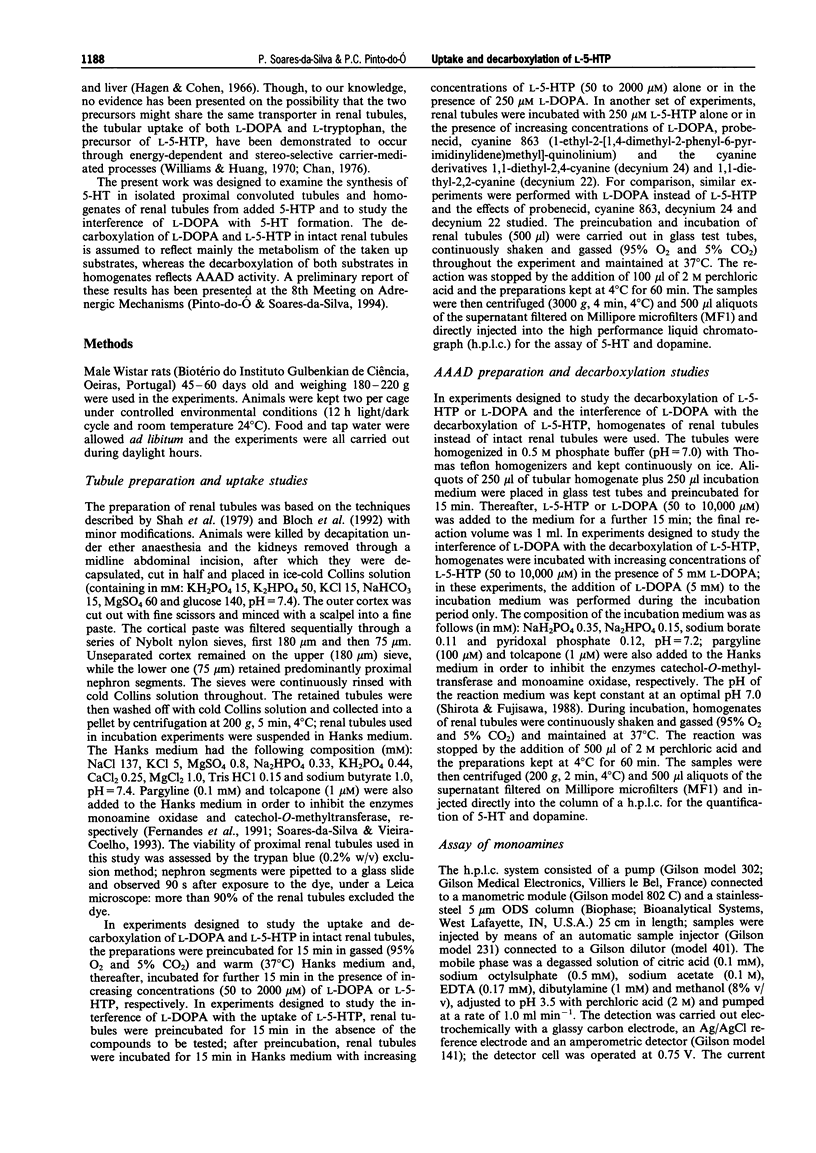
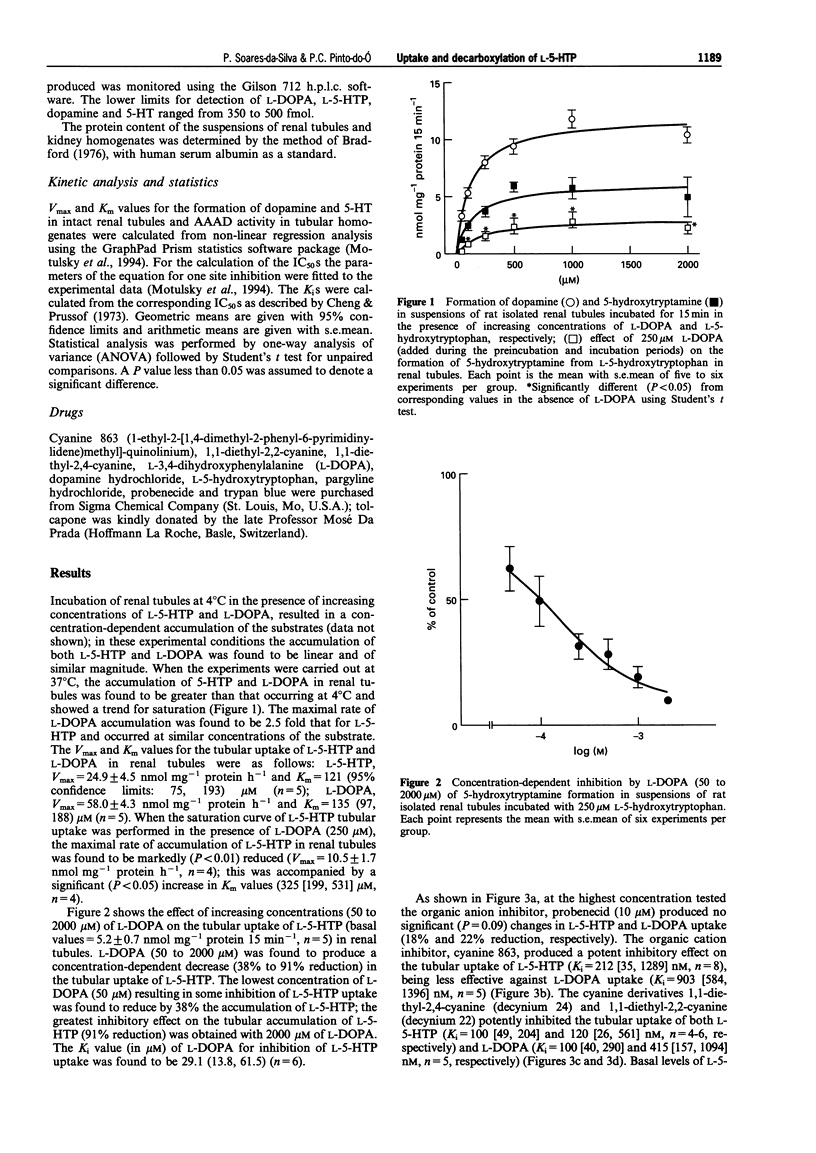
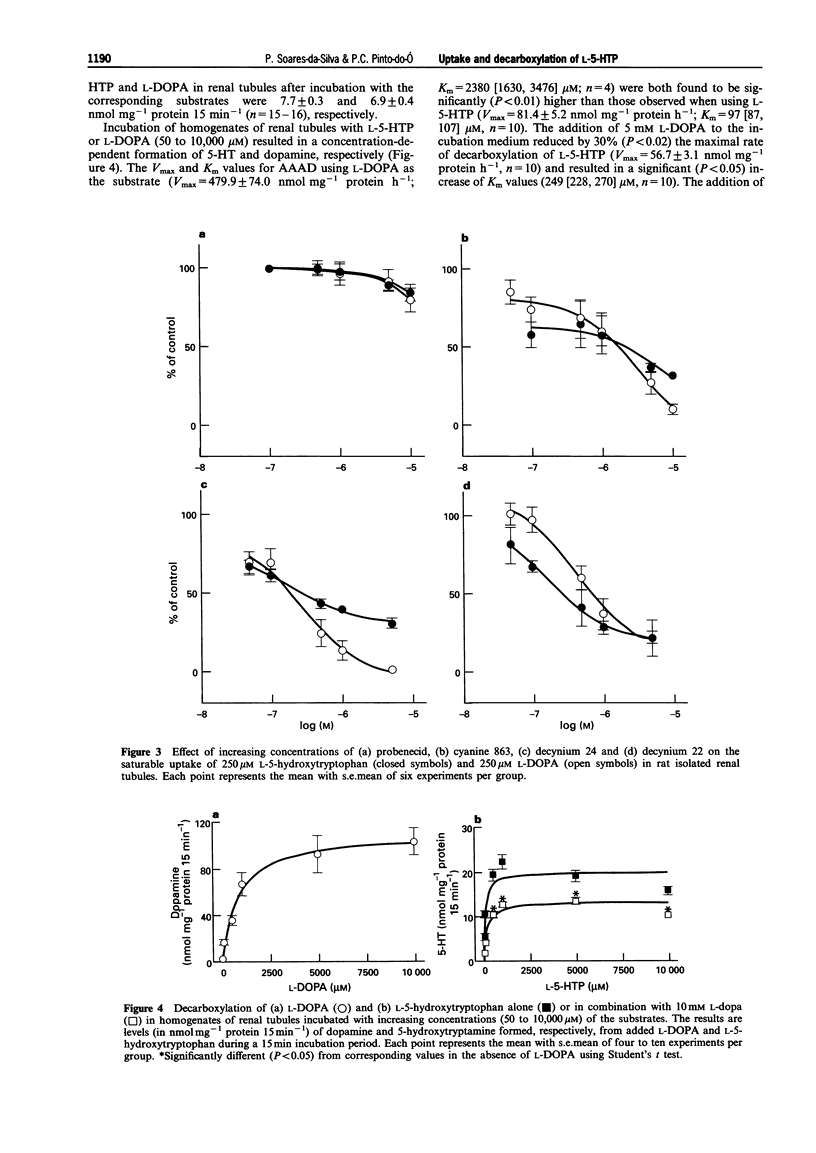
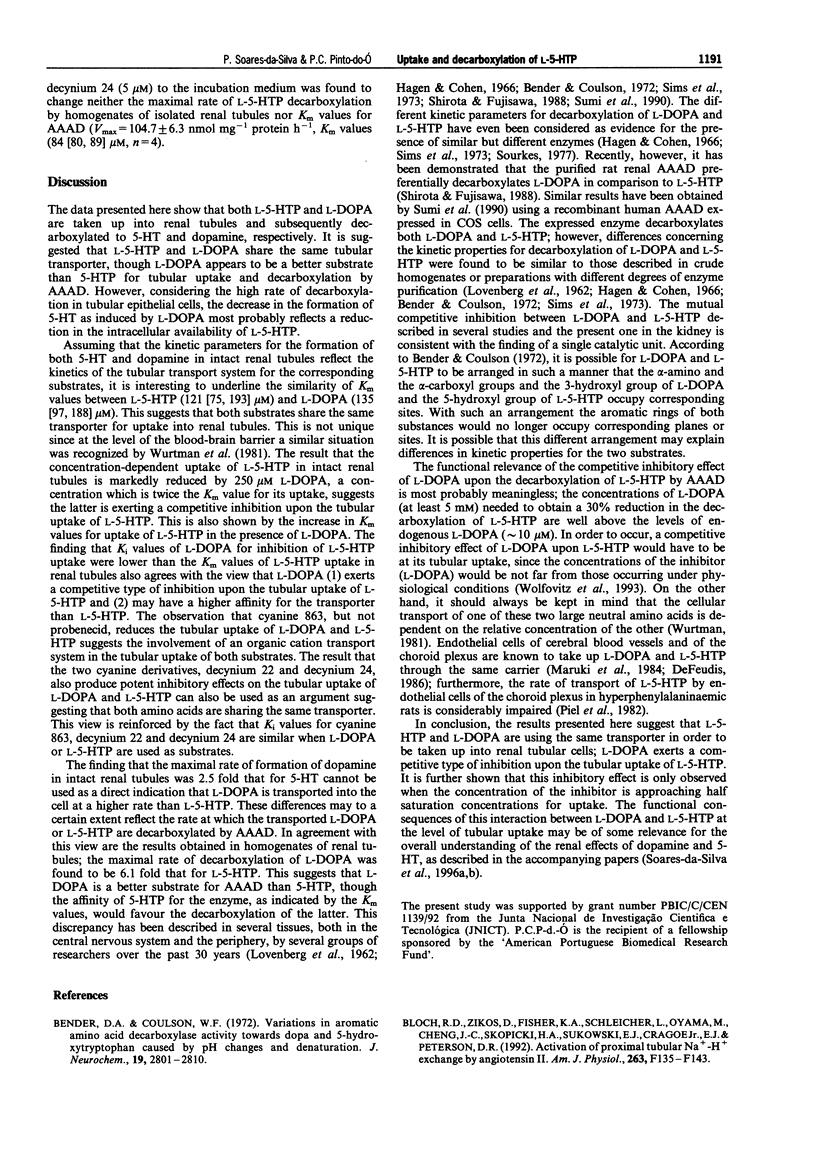
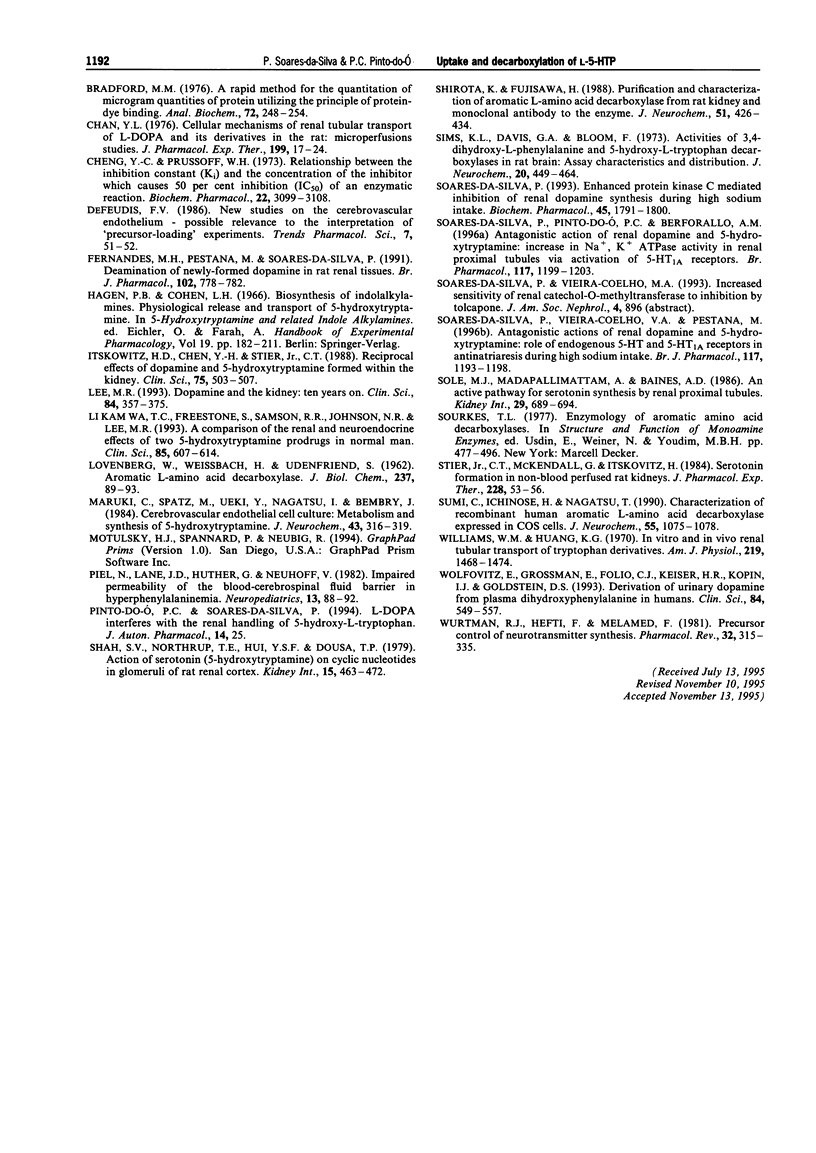
Selected References
These references are in PubMed. This may not be the complete list of references from this article.
- Bender D. A., Coulson W. F. Variations in aromatic amino acid decarboxylase activity towards DOPA and 5-hydroxytryptophan caused by pH changes and denaturation. J Neurochem. 1972 Dec;19(12):2801–2810. doi: 10.1111/j.1471-4159.1972.tb03817.x. [DOI] [PubMed] [Google Scholar]
- Bloch R. D., Zikos D., Fisher K. A., Schleicher L., Oyama M., Cheng J. C., Skopicki H. A., Sukowski E. J., Cragoe E. J., Jr, Peterson D. R. Activation of proximal tubular Na(+)-H+ exchange by angiotensin II. Am J Physiol. 1992 Jul;263(1 Pt 2):F135–F143. doi: 10.1152/ajprenal.1992.263.1.F135. [DOI] [PubMed] [Google Scholar]
- Bradford M. M. A rapid and sensitive method for the quantitation of microgram quantities of protein utilizing the principle of protein-dye binding. Anal Biochem. 1976 May 7;72:248–254. doi: 10.1006/abio.1976.9999. [DOI] [PubMed] [Google Scholar]
- Chan Y. L. Cellular mechanisms of renal tubular transport of I-dopa and its derivatives in the rat: microperfusion studies. J Pharmacol Exp Ther. 1976 Oct;199(1):17–24. [PubMed] [Google Scholar]
- Cheng Y., Prusoff W. H. Relationship between the inhibition constant (K1) and the concentration of inhibitor which causes 50 per cent inhibition (I50) of an enzymatic reaction. Biochem Pharmacol. 1973 Dec 1;22(23):3099–3108. doi: 10.1016/0006-2952(73)90196-2. [DOI] [PubMed] [Google Scholar]
- Fernandes M. H., Pestana M., Soares-da-Silva P. Deamination of newly-formed dopamine in rat renal tissues. Br J Pharmacol. 1991 Mar;102(3):778–782. doi: 10.1111/j.1476-5381.1991.tb12250.x. [DOI] [PMC free article] [PubMed] [Google Scholar]
- Itskovitz H. D., Chen Y. H., Stier C. T., Jr Reciprocal renal effects of dopamine and 5-hydroxytryptamine formed within the rat kidney. Clin Sci (Lond) 1988 Nov;75(5):503–507. doi: 10.1042/cs0750503. [DOI] [PubMed] [Google Scholar]
- LOVENBERG W., WEISSBACH H., UDENFRIEND S. Aromatic L-amino acid decarboxylase. J Biol Chem. 1962 Jan;237:89–93. [PubMed] [Google Scholar]
- Lee M. R. Dopamine and the kidney: ten years on. Clin Sci (Lond) 1993 Apr;84(4):357–375. doi: 10.1042/cs0840357. [DOI] [PubMed] [Google Scholar]
- Li Kam Wa T. C., Freestone S., Samson R. R., Johnson N. R., Lee M. R. A comparison of the renal and neuroendocrine effects of two 5-hydroxytryptamine renal prodrugs in normal man. Clin Sci (Lond) 1993 Nov;85(5):607–614. doi: 10.1042/cs0850607. [DOI] [PubMed] [Google Scholar]
- Maruki C., Spatz M., Ueki Y., Nagatsu I., Bembry J. Cerebrovascular endothelial cell culture: metabolism and synthesis of 5-hydroxytryptamine. J Neurochem. 1984 Aug;43(2):316–319. doi: 10.1111/j.1471-4159.1984.tb00902.x. [DOI] [PubMed] [Google Scholar]
- Piel N., Lane J. D., Hüther G., Neuhoff V. Impaired permeability of the blood-cerebrospinal fluid barrier in hyperphenylalaninaemia. Neuropediatrics. 1982 May;13(2):88–92. doi: 10.1055/s-2008-1059603. [DOI] [PubMed] [Google Scholar]
- Shah S. V., Northrup T. E., Hui Y. S., Dousa T. P. Action of serotonin (5-hydroxytryptamine) on cyclic nucleotides in glomeruli of rat renal cortex. Kidney Int. 1979 May;15(5):463–472. doi: 10.1038/ki.1979.62. [DOI] [PubMed] [Google Scholar]
- Shirota K., Fujisawa H. Purification and characterization of aromatic L-amino acid decarboxylase from rat kidney and monoclonal antibody to the enzyme. J Neurochem. 1988 Aug;51(2):426–434. doi: 10.1111/j.1471-4159.1988.tb01056.x. [DOI] [PubMed] [Google Scholar]
- Sims K. L., Davis G. A., Bloom F. E. Activities of 3,4-dihydroxy-L-phenylalanine and 5-hydroxy-L-tryptophan decarboxylases in rat brain: assay characteristics and distribution. J Neurochem. 1973 Feb;20(2):449–464. doi: 10.1111/j.1471-4159.1973.tb12144.x. [DOI] [PubMed] [Google Scholar]
- Soares-da-Silva P. Enhanced protein kinase C mediated inhibition of renal dopamine synthesis during high sodium intake. Biochem Pharmacol. 1993 May 5;45(9):1791–1800. doi: 10.1016/0006-2952(93)90435-y. [DOI] [PubMed] [Google Scholar]
- Soares-da-Silva P., Pinto-do-O P. C., Bertorello A. M. Antagonistic actions of renal dopamine and 5-hydroxytryptamine: increase in Na+, K(+)-ATPase activity in renal proximal tubules via activation of 5-HT1A receptors. Br J Pharmacol. 1996 Mar;117(6):1199–1203. doi: 10.1111/j.1476-5381.1996.tb16716.x. [DOI] [PMC free article] [PubMed] [Google Scholar]
- Soares-da-Silva P., Vieira-Coelho M. A., Pestana M. Antagonistic actions of renal dopamine and 5-hydroxytryptamine: endogenous 5-hydroxytryptamine, 5-HT1A receptors and antinatriuresis during high sodium intake. Br J Pharmacol. 1996 Mar;117(6):1193–1198. doi: 10.1111/j.1476-5381.1996.tb16715.x. [DOI] [PMC free article] [PubMed] [Google Scholar]
- Sole M. J., Madapallimattam A., Baines A. D. An active pathway for serotonin synthesis by renal proximal tubules. Kidney Int. 1986 Mar;29(3):689–694. doi: 10.1038/ki.1986.53. [DOI] [PubMed] [Google Scholar]
- Stier C. T., Jr, McKendall G., Itskovitz H. D. Serotonin formation in nonblood-perfused rat kidneys. J Pharmacol Exp Ther. 1984 Jan;228(1):53–56. [PubMed] [Google Scholar]
- Sumi C., Ichinose H., Nagatsu T. Characterization of recombinant human aromatic L-amino acid decarboxylase expressed in COS cells. J Neurochem. 1990 Sep;55(3):1075–1078. doi: 10.1111/j.1471-4159.1990.tb04601.x. [DOI] [PubMed] [Google Scholar]
- Williams W. M., Huang K. C. In vitro and in vivo renal tubular transport of tryptophan derivatives. Am J Physiol. 1970 Nov;219(5):1468–1474. doi: 10.1152/ajplegacy.1970.219.5.1468. [DOI] [PubMed] [Google Scholar]
- Wolfovitz E., Grossman E., Folio C. J., Keiser H. R., Kopin I. J., Goldstein D. S. Derivation of urinary dopamine from plasma dihydroxyphenylalanine in humans. Clin Sci (Lond) 1993 May;84(5):549–557. doi: 10.1042/cs0840549. [DOI] [PubMed] [Google Scholar]
- Wurtman R. J., Hefti F., Melamed E. Precursor control of neurotransmitter synthesis. Pharmacol Rev. 1980 Dec;32(4):315–335. [PubMed] [Google Scholar]