Abstract
Strain SR, a monensin-sensitive, ammonia-producing ruminal bacterium, grew rapidly on arginine and lysine, but only if sodium was present. Arginine transport could be driven by either an electrical potential or a chemical gradient of sodium. Arginine was converted to ornithine, and it appeared that ornithine efflux created a sodium gradient which in turn drove arginine transport. There was a linear decline in arginine transport as pH was decreased from 7.5 to 5.5, and the cells did not grow at a pH less than 6.0. The Eadie-Hofstee plot was biphasic, and arginine could also be taken by a high-capacity diffusion mechanism. Because arginine was a strong inhibitor of lysine transport and lysine was a weak inhibitor of arginine transport, it appeared that both lysine and arginine were taken up by an arginine-lysine carrier which had a preference for arginine. The rate of lysine fermentation was always proportional to the extracellular lysine concentration, and facilitated diffusion was the dominant mechanism of lysine transport. When SR was grown in continuous culture on arginine or lysine, the theoretical maximal growth yield was similar (13 g of cells per mol of ATP), but the apparent maintenance energy requirement for arginine was greater than lysine (9.4 versus 4.4 mmol of ATP per g of cells per h). On the basis of differences in yield and maintenance energy, it appeared that active arginine transport accounted for approximately 40% of the total ATP.
Full text
PDF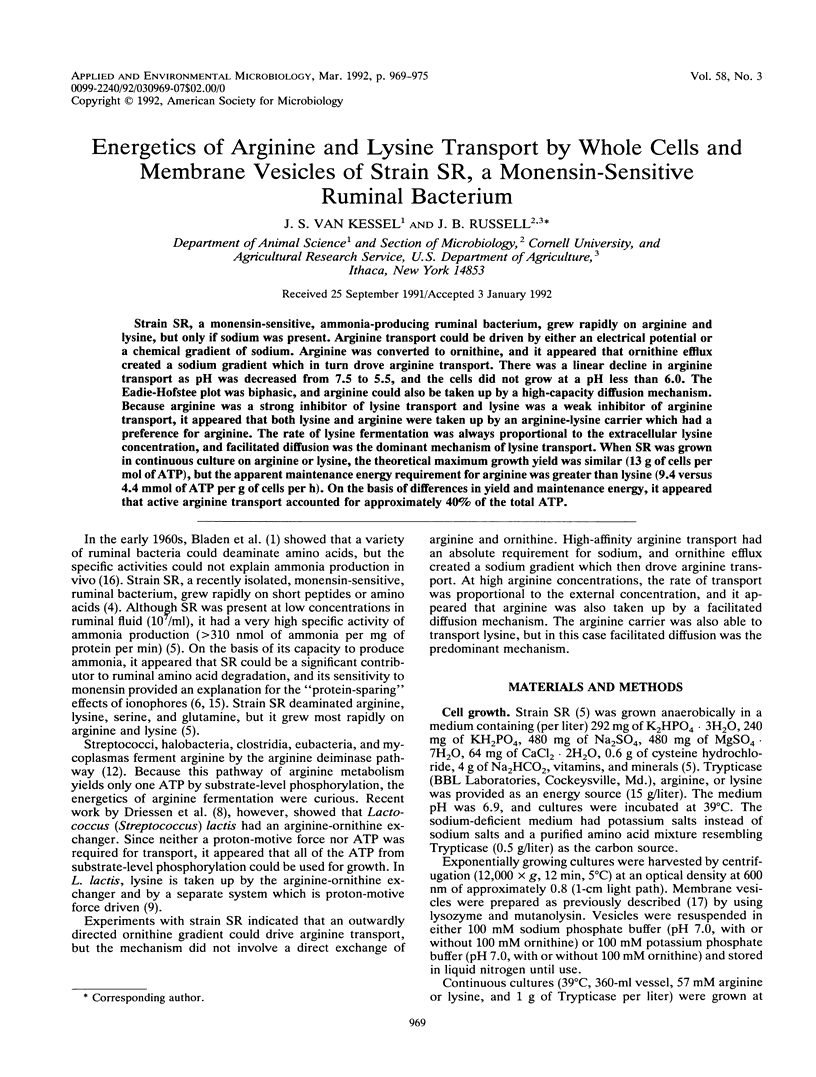
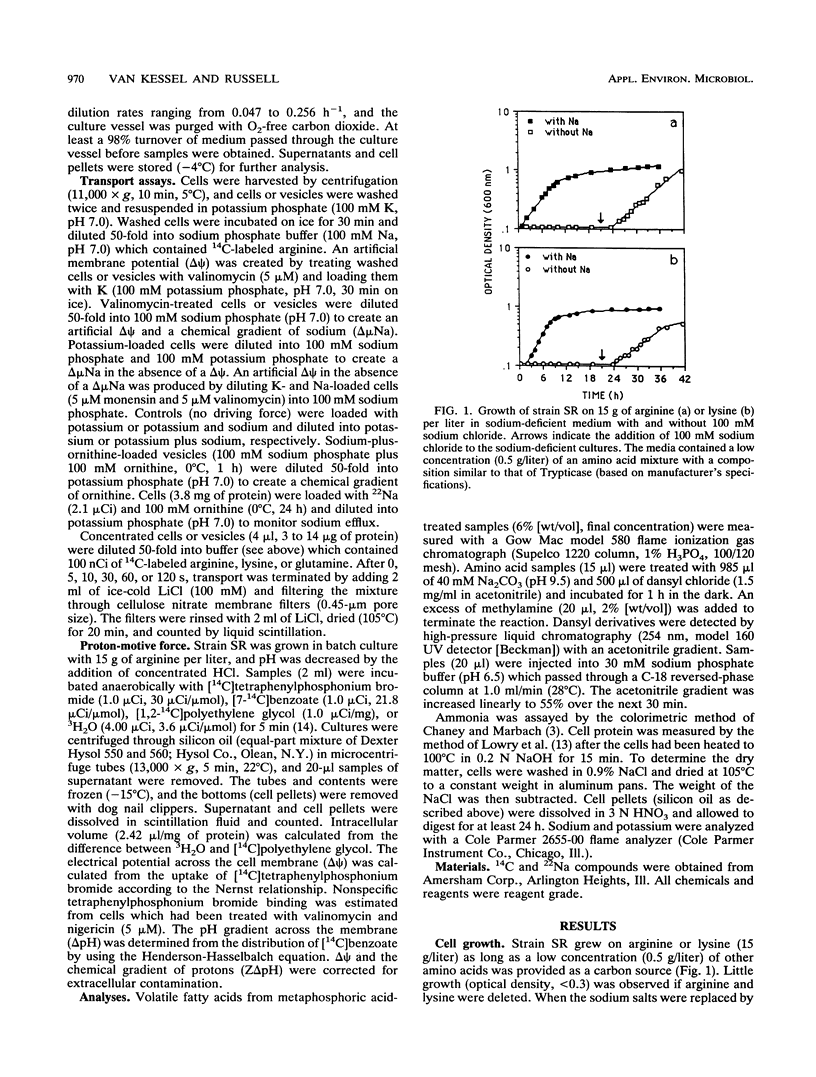
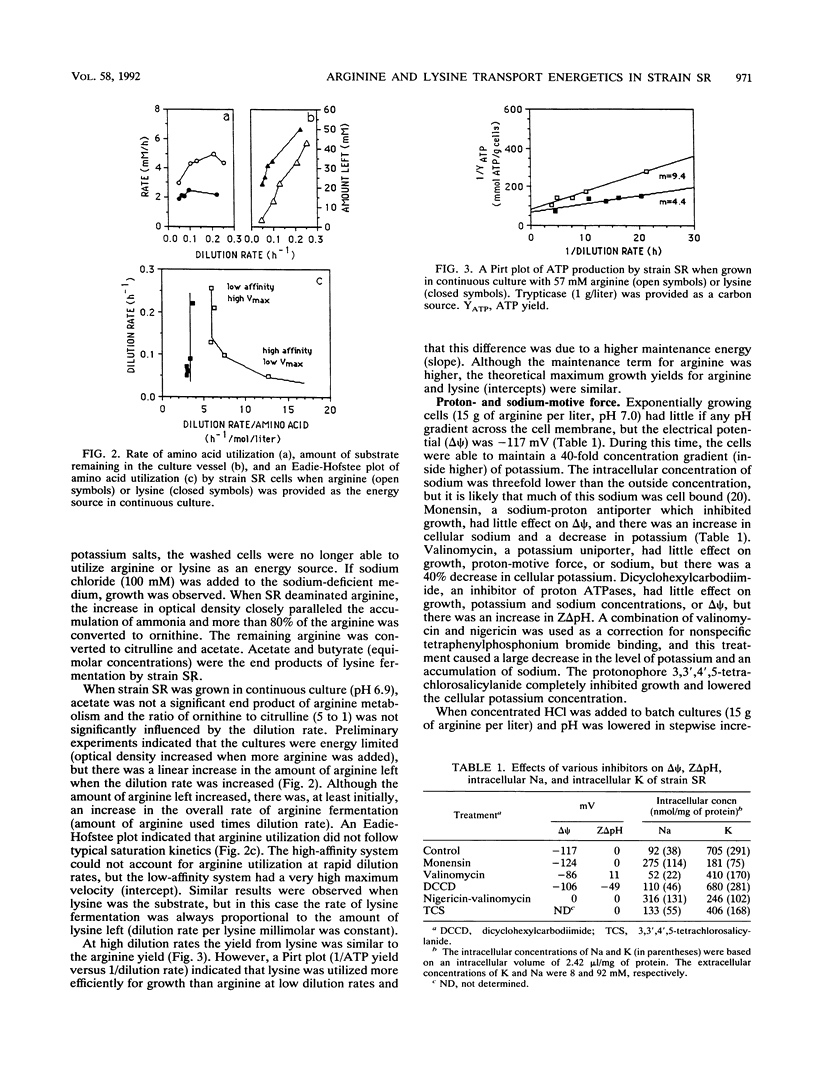
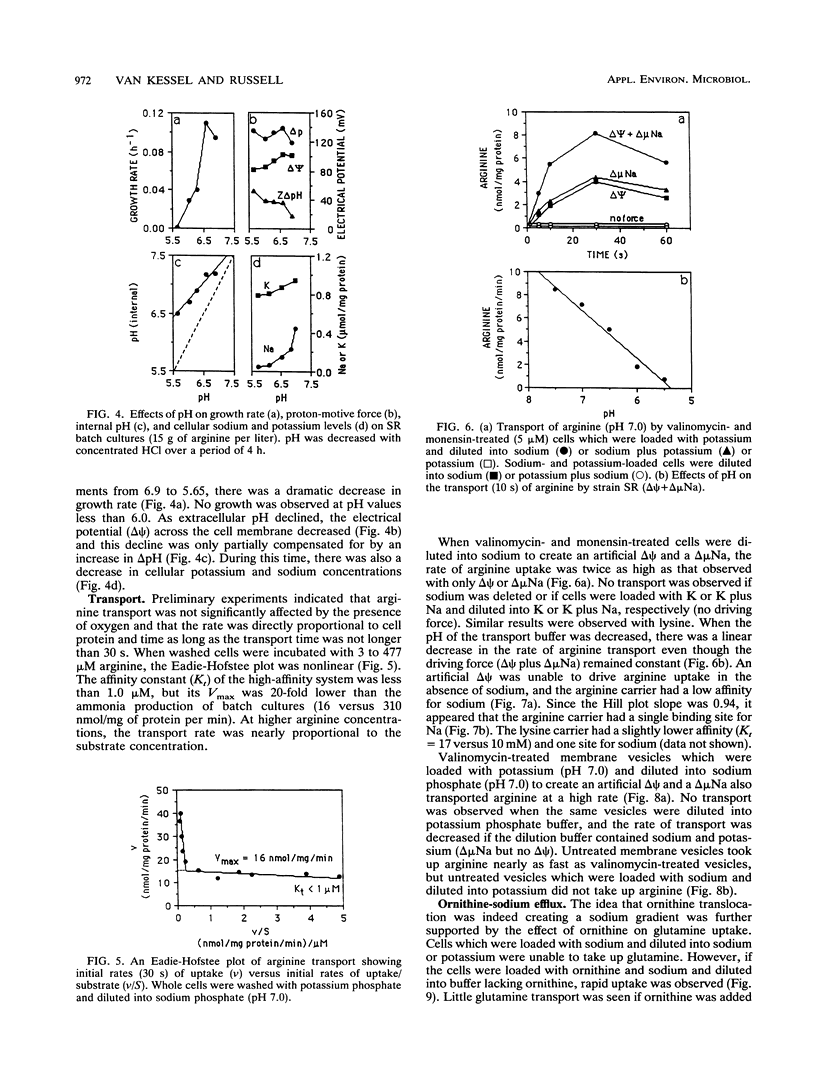
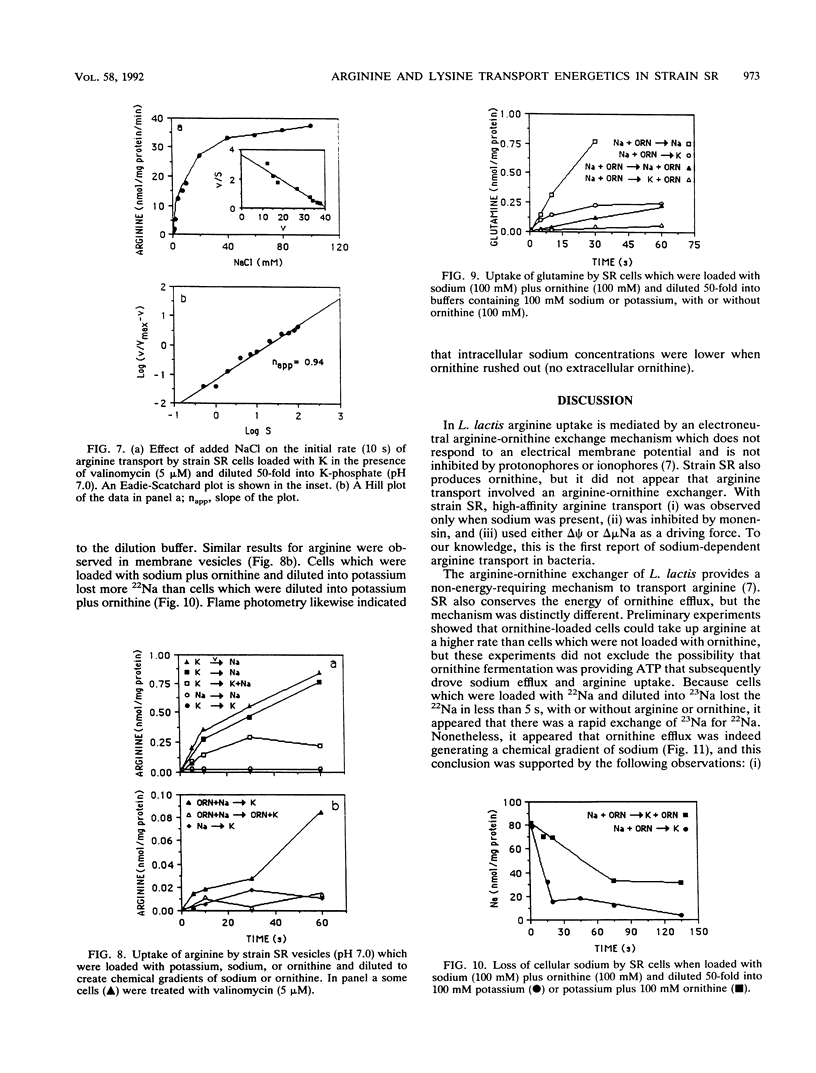
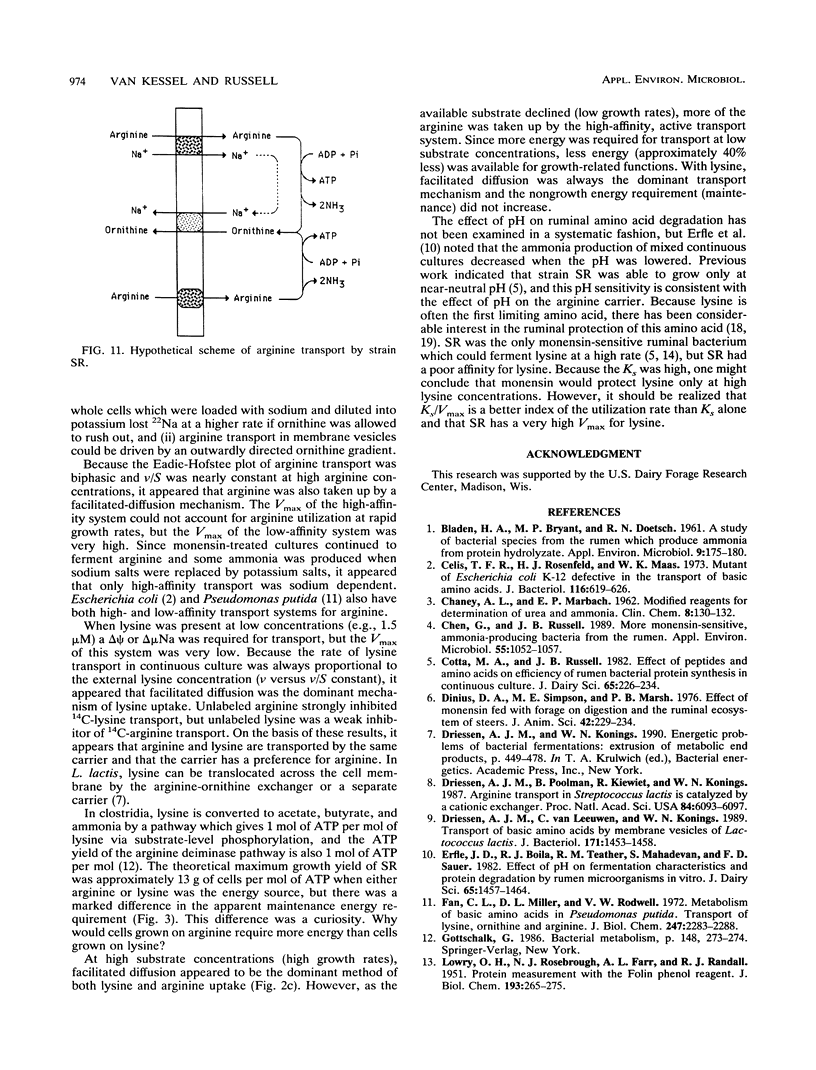
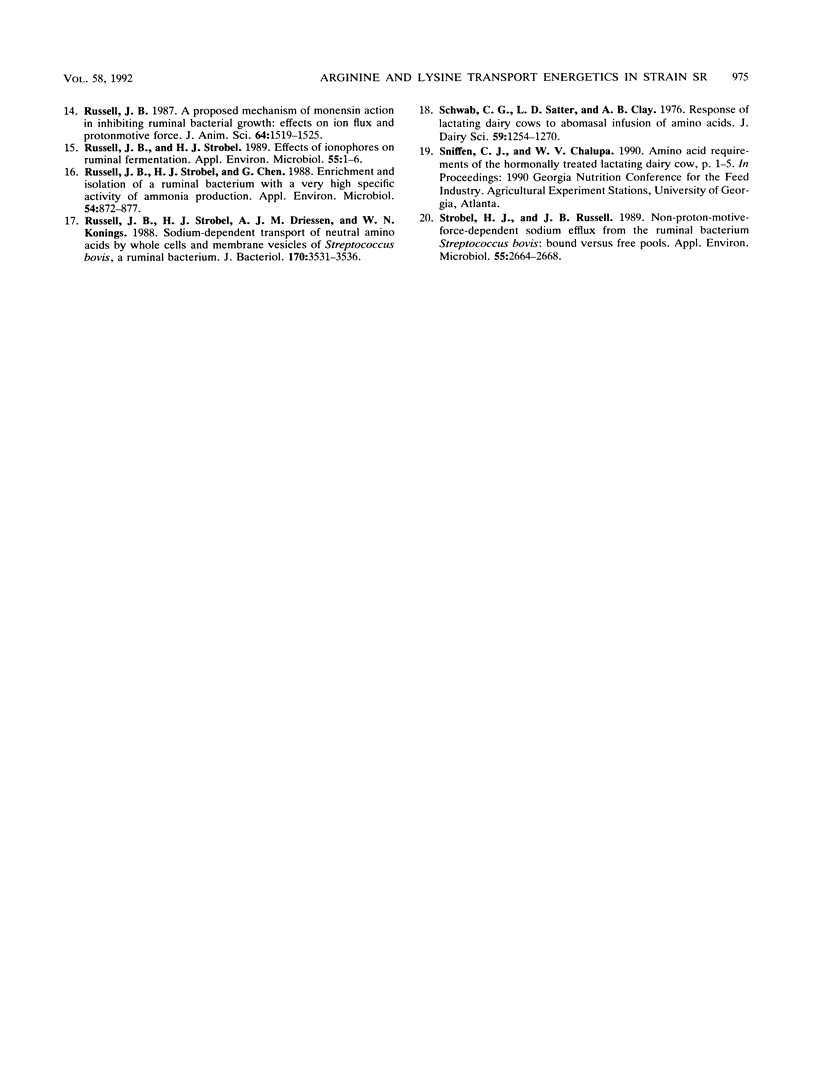
Selected References
These references are in PubMed. This may not be the complete list of references from this article.
- Bladen H. A., Bryant M. P., Doetsch R. N. A Study of Bacterial Species from the Rumen Which Produce Ammonia from Protein Hydrolyzate. Appl Microbiol. 1961 Mar;9(2):175–180. doi: 10.1128/am.9.2.175-180.1961. [DOI] [PMC free article] [PubMed] [Google Scholar]
- CHANEY A. L., MARBACH E. P. Modified reagents for determination of urea and ammonia. Clin Chem. 1962 Apr;8:130–132. [PubMed] [Google Scholar]
- Celis T. F., Rosenfeld H. J., Maas W. K. Mutant of Escherichia coli K-12 defective in the transport of basic amino acids. J Bacteriol. 1973 Nov;116(2):619–626. doi: 10.1128/jb.116.2.619-626.1973. [DOI] [PMC free article] [PubMed] [Google Scholar]
- Chen G., Russell J. B. More monensin-sensitive, ammonia-producing bacteria from the rumen. Appl Environ Microbiol. 1989 May;55(5):1052–1057. doi: 10.1128/aem.55.5.1052-1057.1989. [DOI] [PMC free article] [PubMed] [Google Scholar]
- Donius D. A., Simpson M. E., Marsh P. B. Effect of monensin fed with forage on digestion and the ruminal ecosystem of steers. J Anim Sci. 1976 Jan;42(1):229–234. doi: 10.2527/jas1976.421229x. [DOI] [PubMed] [Google Scholar]
- Driessen A. J., Poolman B., Kiewiet R., Konings W. Arginine transport in Streptococcus lactis is catalyzed by a cationic exchanger. Proc Natl Acad Sci U S A. 1987 Sep;84(17):6093–6097. doi: 10.1073/pnas.84.17.6093. [DOI] [PMC free article] [PubMed] [Google Scholar]
- Driessen A. J., van Leeuwen C., Konings W. N. Transport of basic amino acids by membrane vesicles of Lactococcus lactis. J Bacteriol. 1989 Mar;171(3):1453–1458. doi: 10.1128/jb.171.3.1453-1458.1989. [DOI] [PMC free article] [PubMed] [Google Scholar]
- Fan C. L., Miller D. L., Rodwell V. W. Metabolism of basic amino acids in Pseudomonas putida. Transport of lysine, ornithine, and arginine. J Biol Chem. 1972 Apr 25;247(8):2283–2288. [PubMed] [Google Scholar]
- LOWRY O. H., ROSEBROUGH N. J., FARR A. L., RANDALL R. J. Protein measurement with the Folin phenol reagent. J Biol Chem. 1951 Nov;193(1):265–275. [PubMed] [Google Scholar]
- Russell J. B. A proposed mechanism of monensin action in inhibiting ruminal bacterial growth: effects on ion flux and protonmotive force. J Anim Sci. 1987 May;64(5):1519–1525. doi: 10.2527/jas1987.6451519x. [DOI] [PubMed] [Google Scholar]
- Russell J. B., Strobel H. J., Chen G. J. Enrichment and isolation of a ruminal bacterium with a very high specific activity of ammonia production. Appl Environ Microbiol. 1988 Apr;54(4):872–877. doi: 10.1128/aem.54.4.872-877.1988. [DOI] [PMC free article] [PubMed] [Google Scholar]
- Russell J. B., Strobel H. J., Driessen A. J., Konings W. N. Sodium-dependent transport of neutral amino acids by whole cells and membrane vesicles of Streptococcus bovis, a ruminal bacterium. J Bacteriol. 1988 Aug;170(8):3531–3536. doi: 10.1128/jb.170.8.3531-3536.1988. [DOI] [PMC free article] [PubMed] [Google Scholar]
- Russell J. B., Strobel H. J. Effect of ionophores on ruminal fermentation. Appl Environ Microbiol. 1989 Jan;55(1):1–6. doi: 10.1128/aem.55.1.1-6.1989. [DOI] [PMC free article] [PubMed] [Google Scholar]
- Schwab C. G., Satter L. D., Clay B. Response to lactating dairy cows to abomasal infusion of amino acids. J Dairy Sci. 1976 Jul;59(7):1254–1270. doi: 10.3168/jds.s0022-0302(76)84354-8. [DOI] [PubMed] [Google Scholar]
- Strobel H. J., Russell J. B. Non-proton-motive-force-dependent sodium efflux from the ruminal bacterium Streptococcus bovis: bound versus free pools. Appl Environ Microbiol. 1989 Oct;55(10):2664–2668. doi: 10.1128/aem.55.10.2664-2668.1989. [DOI] [PMC free article] [PubMed] [Google Scholar]