Abstract
A thymidine incorporation technique was used to determine the tolerance of a soil bacterial community to Cu, Cd, Zn, Ni, and Pb. An agricultural soil was artificially contaminated in our laboratory with individual metals at three different concentrations, and the results were compared with the results obtained by using the plate count technique. Thymidine incorporation was found to be a simple and rapid method for measuring tolerance. Data obtained by this technique were very reproducible. A linear relationship was found between changes in community tolerance levels obtained by the thymidine incorporation and plate count techniques (r = 0.732, P < 0.001). An increase in tolerance to the metal added to soil was observed for the bacterial community obtained from each polluted soil compared with the community obtained from unpolluted soil. The only exception was when Pb was added; no indication of Pb tolerance was found. An increase in the tolerance to metals other than the metal originally added to soil was also observed, indicating that there was multiple heavy metal tolerance at the community level. Thus, Cu pollution, in addition to increasing tolerance to Cu, also induced tolerance to Zn, Cd, and Ni. Zn and Cd pollution increased community tolerance to all five metals. Ni amendment increased tolerance to Ni the most but also increased community tolerance to Zn and, to lesser degrees, increased community tolerance to Pb and Cd. In soils polluted with Pb increased tolerance to other metals was found in the following order: Ni > Cd > Zn > Cu. We found significant positive relationships between changes in Cd, Zn, and Pb tolerance and, to a lesser degree, between changes in Pb and Ni tolerance when all metals and amendment levels were compared. The magnitude of the increase in heavy metal tolerance was found to be linearly related to the logarithm of the metal concentration added to the soil. Threshold tolerance concentrations were estimated from these linear relationships, and changes in tolerance could be detected at levels of soil contamination similar to those reported previously to result in changes in the phospholipid fatty acid pattern (Å. Frostegård, A. Tunlid, and E. Bååth, Appl. Environ. Microbiol. 59: 3605-3617, 1993).
Full text
PDF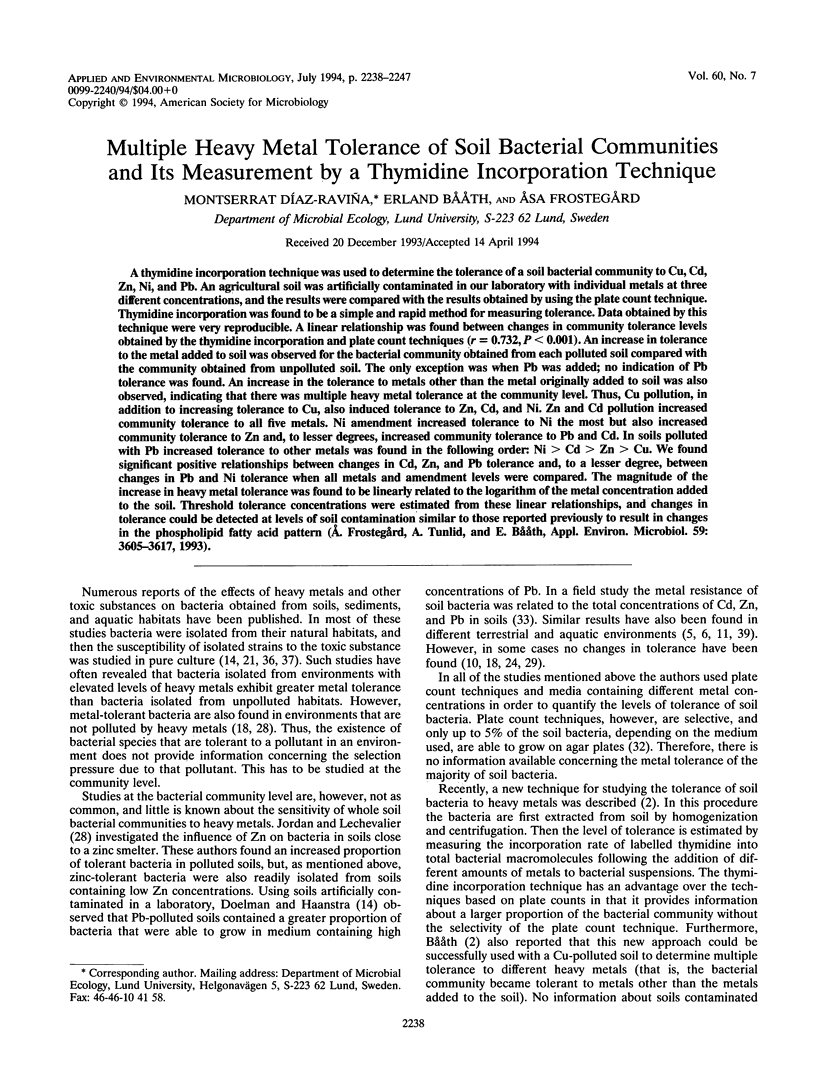
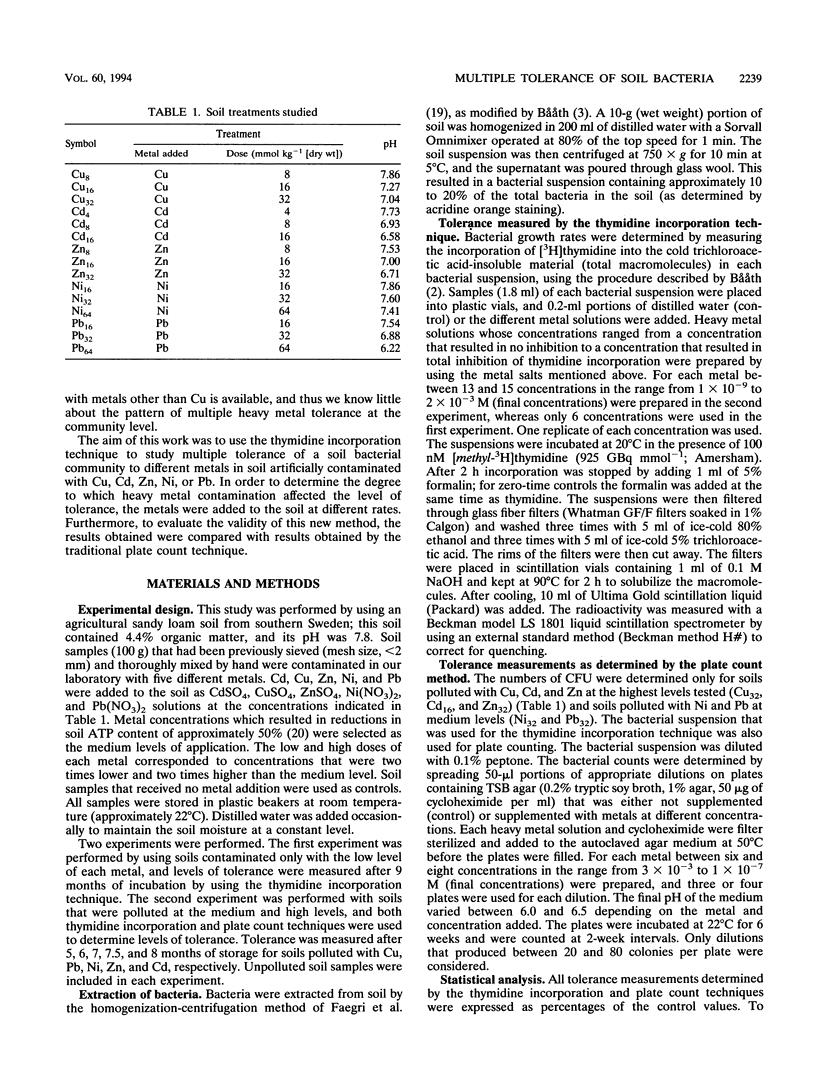
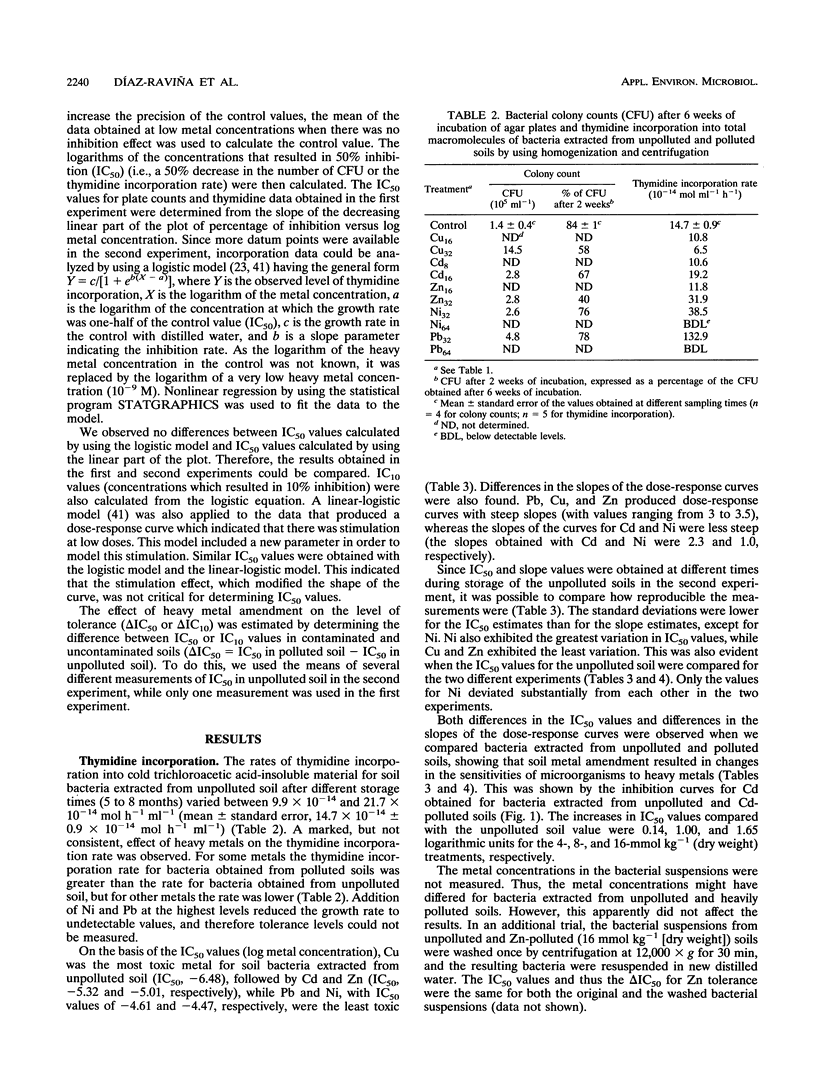
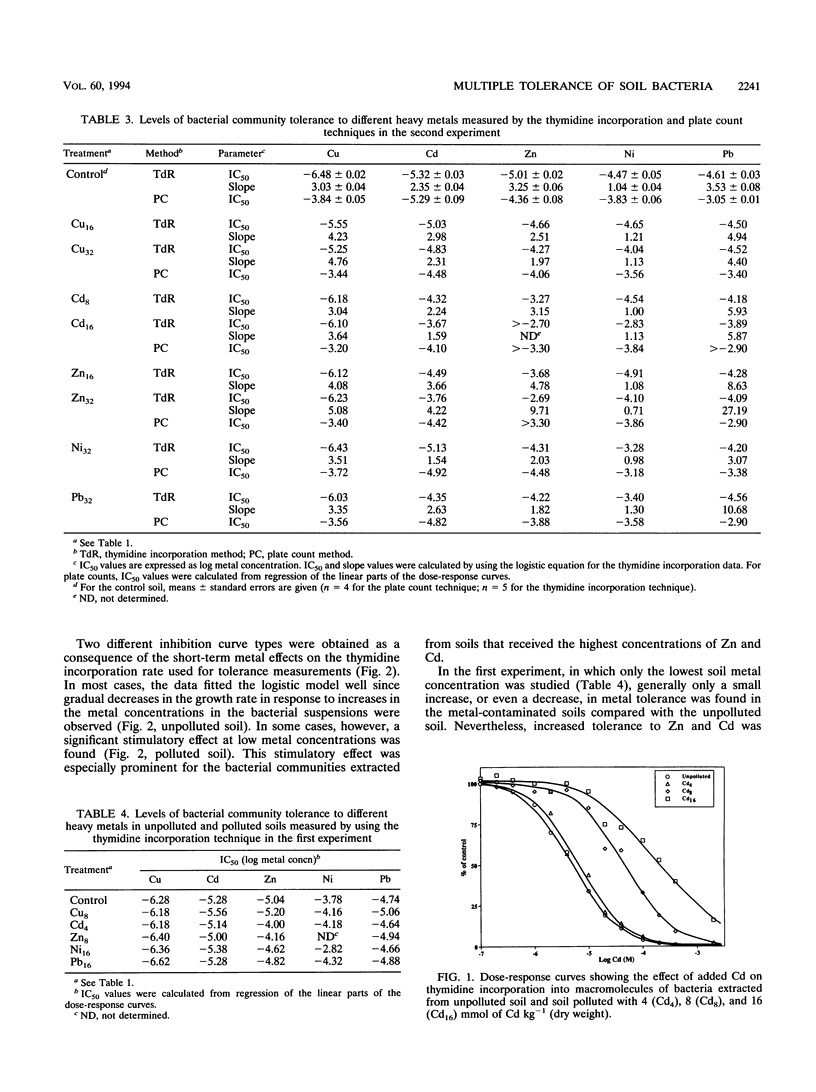
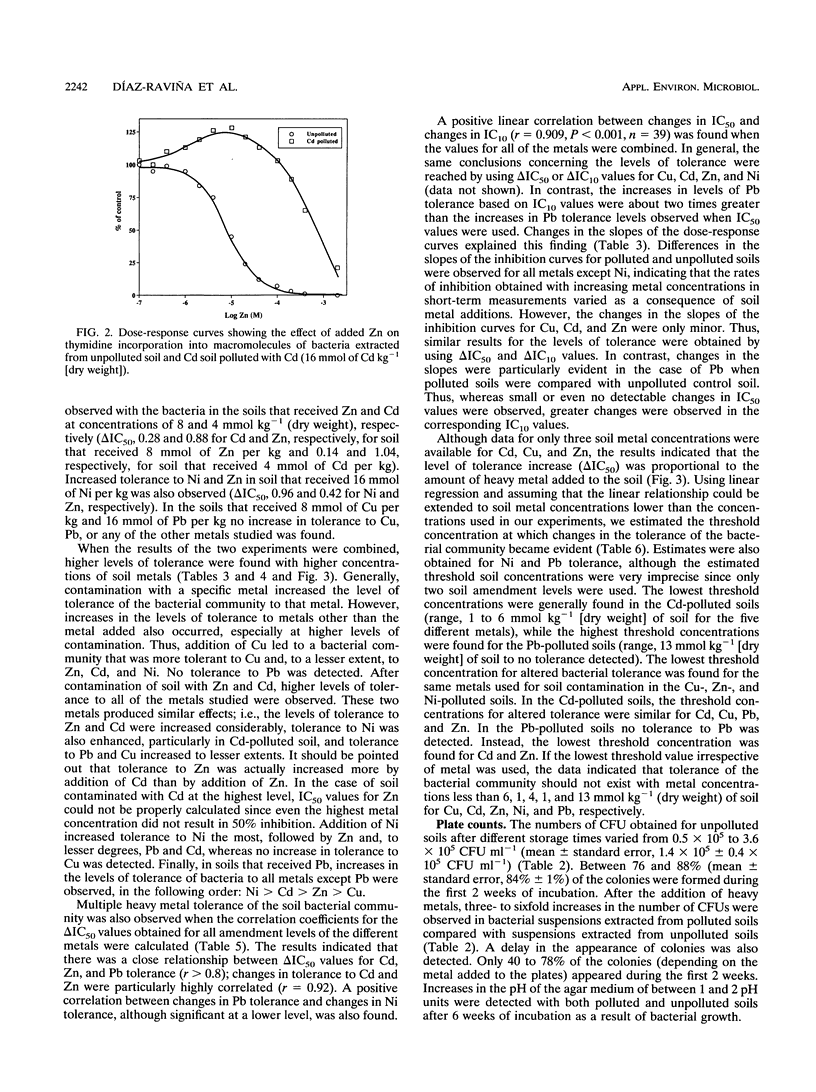
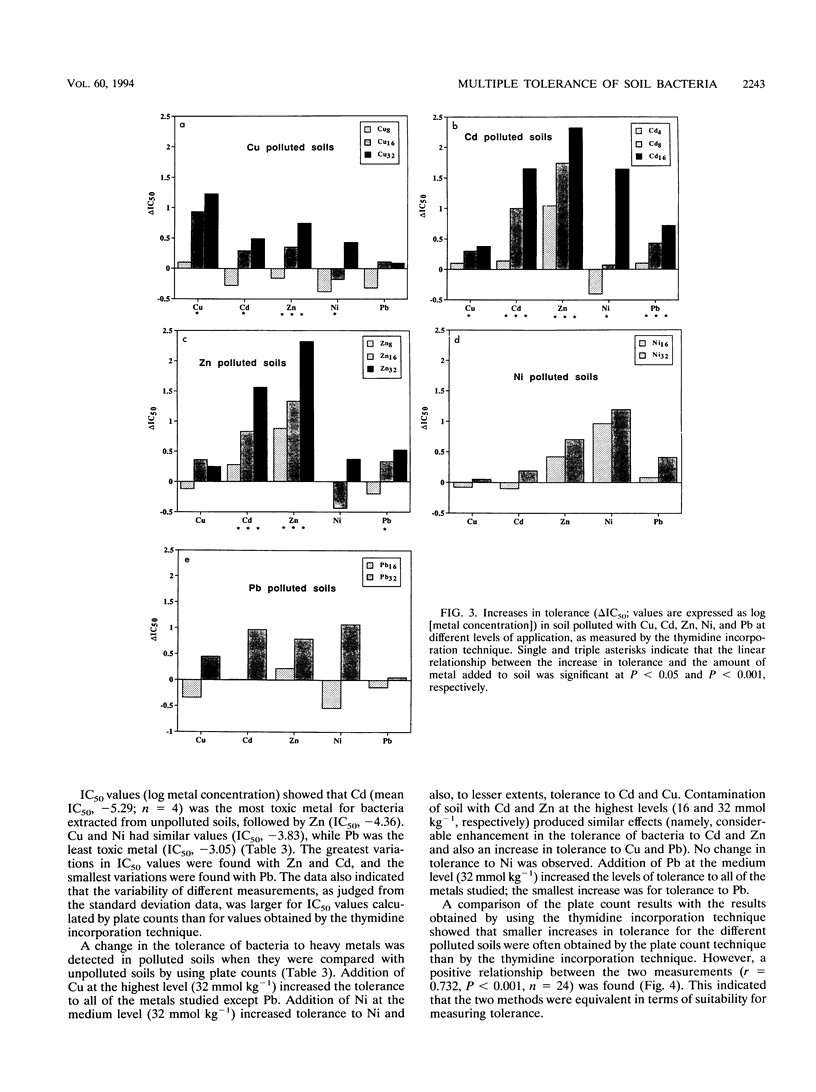
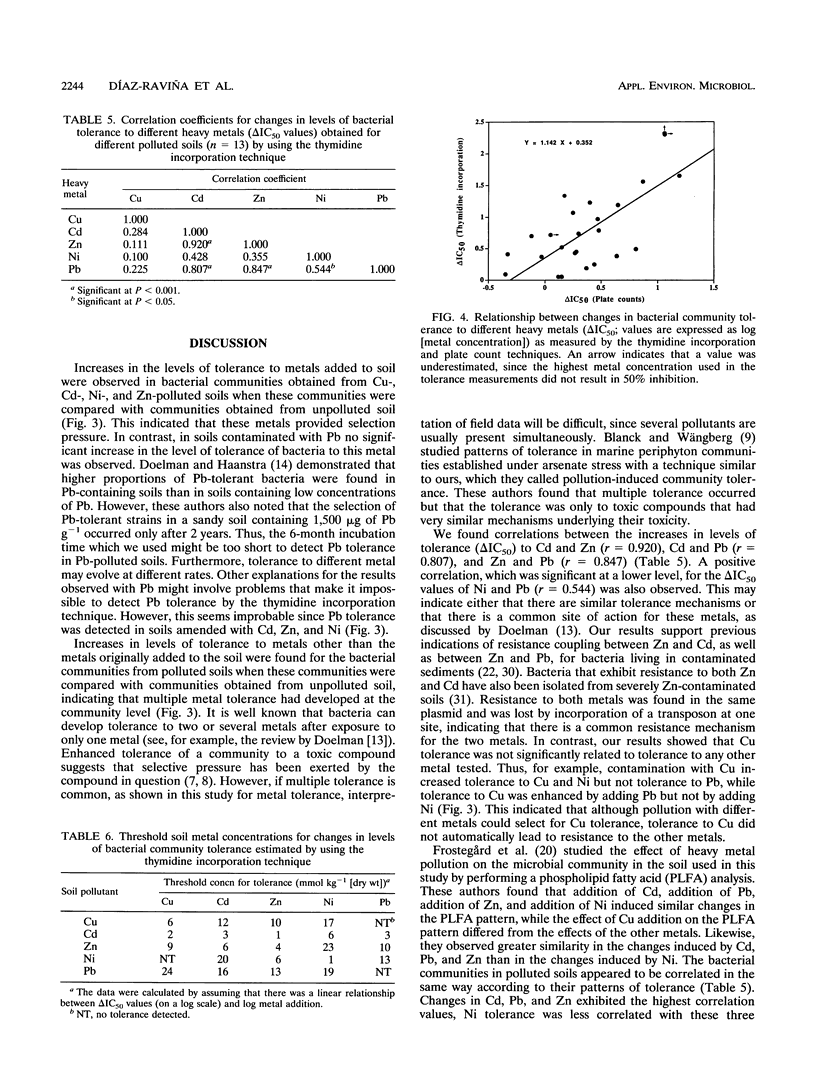
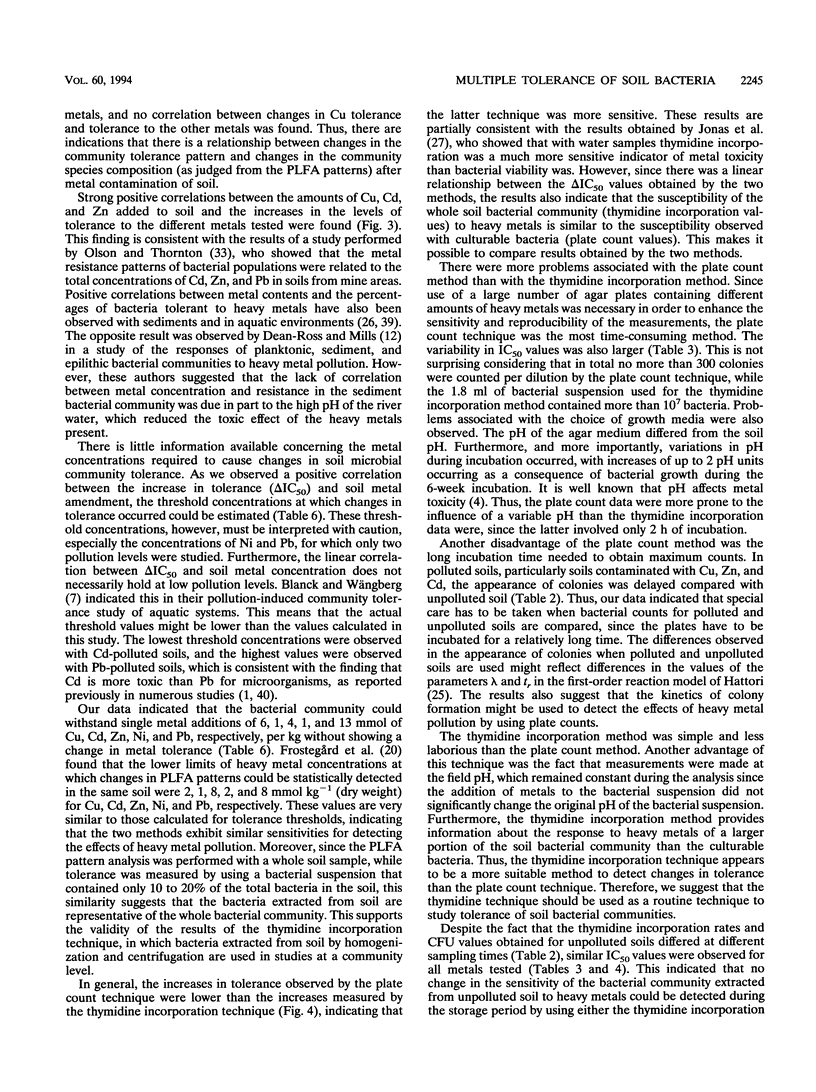
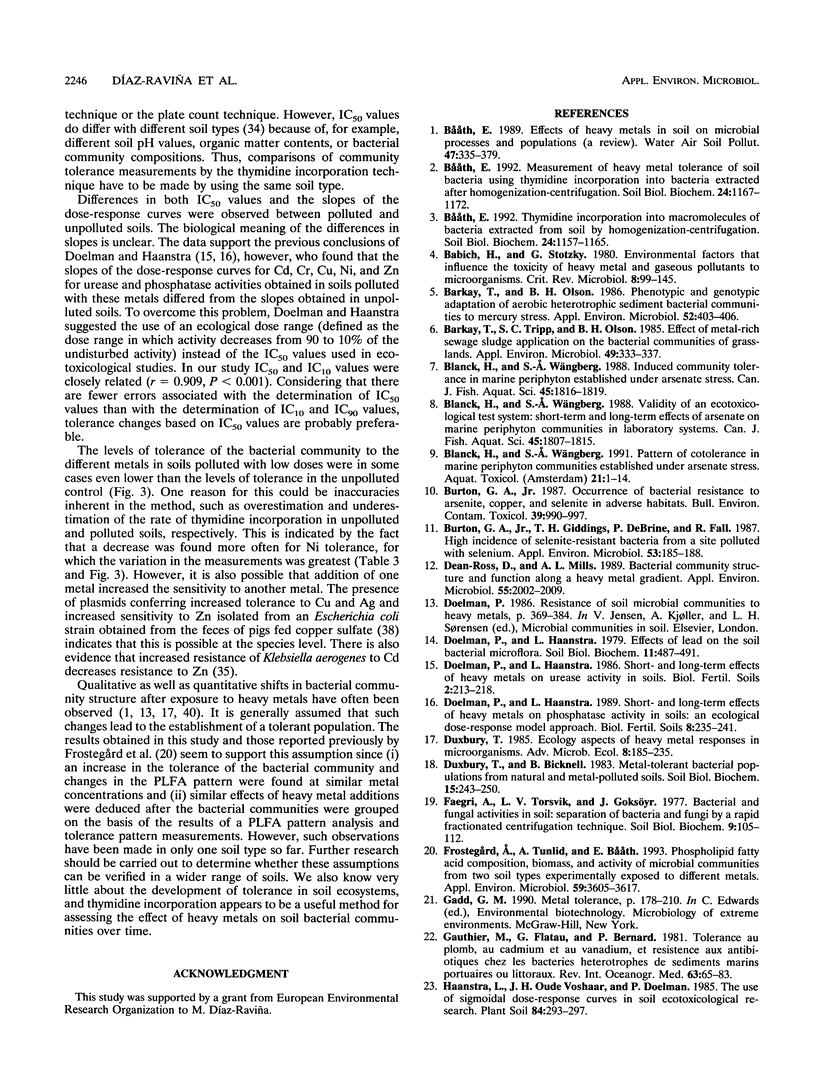
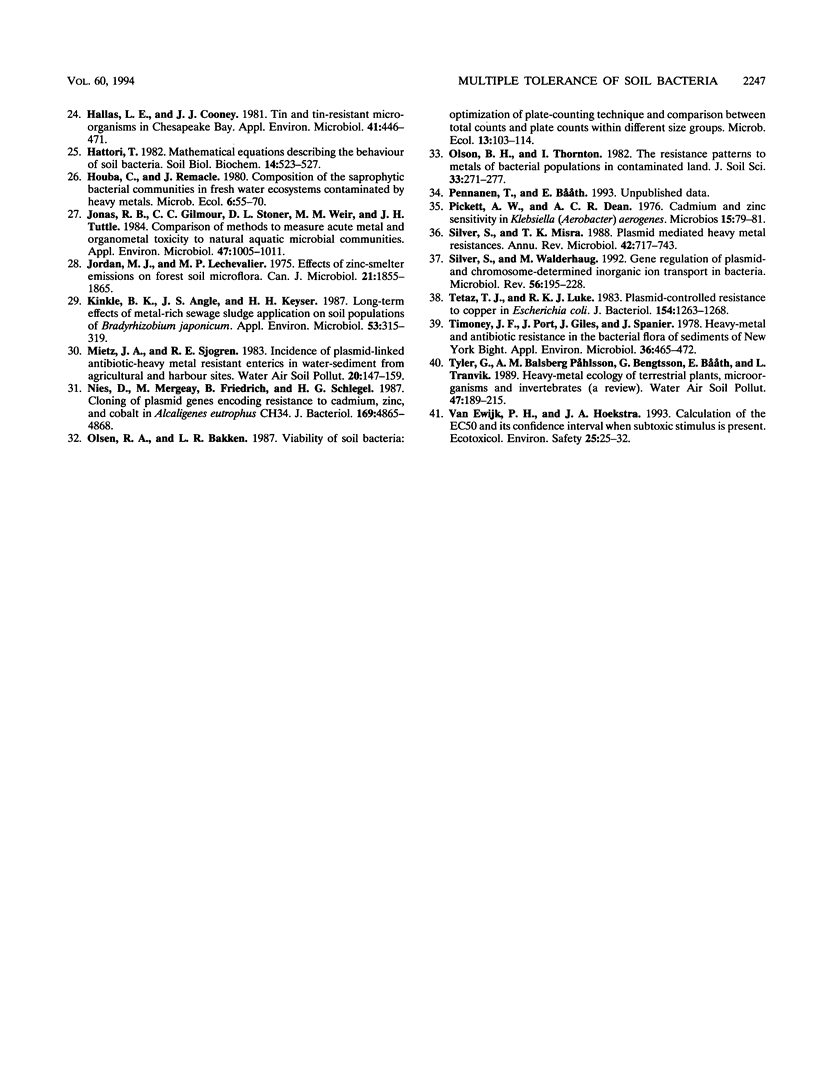
Selected References
These references are in PubMed. This may not be the complete list of references from this article.
- Babich H., Stotzky G. Environmental factors that influence the toxicity of heavy metal and gaseous pollutants to microorganisms. Crit Rev Microbiol. 1980;8(2):99–145. doi: 10.3109/10408418009081123. [DOI] [PubMed] [Google Scholar]
- Barkay T., Olson B. H. Phenotypic and genotypic adaptation of aerobic heterotrophic sediment bacterial communities to mercury stress. Appl Environ Microbiol. 1986 Aug;52(2):403–406. doi: 10.1128/aem.52.2.403-406.1986. [DOI] [PMC free article] [PubMed] [Google Scholar]
- Barkay T., Tripp S. C., Olson B. H. Effect of metal-rich sewage sludge application on the bacterial communities of grasslands. Appl Environ Microbiol. 1985 Feb;49(2):333–337. doi: 10.1128/aem.49.2.333-337.1985. [DOI] [PMC free article] [PubMed] [Google Scholar]
- Burton G. A., Jr, Giddings T. H., DeBrine P., Fall R. High incidence of selenite-resistant bacteria from a site polluted with selenium. Appl Environ Microbiol. 1987 Jan;53(1):185–188. doi: 10.1128/aem.53.1.185-188.1987. [DOI] [PMC free article] [PubMed] [Google Scholar]
- Burton G. A., Jr Occurrence of bacterial resistance to arsenite, copper, and selenite in adverse habitats. Bull Environ Contam Toxicol. 1987 Dec;39(6):990–997. doi: 10.1007/BF01689589. [DOI] [PubMed] [Google Scholar]
- Dean-Ross Deborah, Mills Aaron L. Bacterial Community Structure and Function along a Heavy Metal Gradient. Appl Environ Microbiol. 1989 Aug;55(8):2002–2009. doi: 10.1128/aem.55.8.2002-2009.1989. [DOI] [PMC free article] [PubMed] [Google Scholar]
- Frostegård A., Tunlid A., Båth E. Phospholipid Fatty Acid composition, biomass, and activity of microbial communities from two soil types experimentally exposed to different heavy metals. Appl Environ Microbiol. 1993 Nov;59(11):3605–3617. doi: 10.1128/aem.59.11.3605-3617.1993. [DOI] [PMC free article] [PubMed] [Google Scholar]
- Hallas L. E., Cooney J. J. Tin and tin-resistant microorganisms in chesapeake bay. Appl Environ Microbiol. 1981 Feb;41(2):466–471. doi: 10.1128/aem.41.2.466-471.1981. [DOI] [PMC free article] [PubMed] [Google Scholar]
- Jonas R. B., Gilmour C. C., Stoner D. L., Weir M. M., Tuttle J. H. Comparison of methods to measure acute metal and organometal toxicity to natural aquatic microbial communities. Appl Environ Microbiol. 1984 May;47(5):1005–1011. doi: 10.1128/aem.47.5.1005-1011.1984. [DOI] [PMC free article] [PubMed] [Google Scholar]
- Jordan M. J., Lechevalier M. P. Effects of zinc-smelter emissions on forest soil microflora. Can J Microbiol. 1975 Nov;21(11):1855–1865. doi: 10.1139/m75-269. [DOI] [PubMed] [Google Scholar]
- Kinkle B. K., Angle J. S., Keyser H. H. Long-Term Effects of Metal-Rich Sewage Sludge Application on Soil Populations of Bradyrhizobium japonicum. Appl Environ Microbiol. 1987 Feb;53(2):315–319. doi: 10.1128/aem.53.2.315-319.1987. [DOI] [PMC free article] [PubMed] [Google Scholar]
- Nies D., Mergeay M., Friedrich B., Schlegel H. G. Cloning of plasmid genes encoding resistance to cadmium, zinc, and cobalt in Alcaligenes eutrophus CH34. J Bacteriol. 1987 Oct;169(10):4865–4868. doi: 10.1128/jb.169.10.4865-4868.1987. [DOI] [PMC free article] [PubMed] [Google Scholar]
- Pickett A. W., Dean A. C. Cadmium and zinc sensitivity and tolerance in Klebsiella (Aerobacter) aerogenes. Microbios. 1976;15(60):79–91. [PubMed] [Google Scholar]
- Silver S., Misra T. K. Plasmid-mediated heavy metal resistances. Annu Rev Microbiol. 1988;42:717–743. doi: 10.1146/annurev.mi.42.100188.003441. [DOI] [PubMed] [Google Scholar]
- Silver S., Walderhaug M. Gene regulation of plasmid- and chromosome-determined inorganic ion transport in bacteria. Microbiol Rev. 1992 Mar;56(1):195–228. doi: 10.1128/mr.56.1.195-228.1992. [DOI] [PMC free article] [PubMed] [Google Scholar]
- Tetaz T. J., Luke R. K. Plasmid-controlled resistance to copper in Escherichia coli. J Bacteriol. 1983 Jun;154(3):1263–1268. doi: 10.1128/jb.154.3.1263-1268.1983. [DOI] [PMC free article] [PubMed] [Google Scholar]
- Timoney J. F., Port J., Giles J., Spanier J. Heavy-metal and antibiotic resistance in the bacterial flora of sediments of New York Bight. Appl Environ Microbiol. 1978 Sep;36(3):465–472. doi: 10.1128/aem.36.3.465-472.1978. [DOI] [PMC free article] [PubMed] [Google Scholar]
- Van Ewijk P. H., Hoekstra J. A. Calculation of the EC50 and its confidence interval when subtoxic stimulus is present. Ecotoxicol Environ Saf. 1993 Feb;25(1):25–32. doi: 10.1006/eesa.1993.1003. [DOI] [PubMed] [Google Scholar]