Abstract
We investigated the microbial community that developed at an iron seep where anoxic groundwater containing up to 250 μM Fe2+ flowed out of a rock wall and dense, mat-like aggregations of ferric hydroxides formed at the oxic-anoxic interface. In situ analysis with oxygen microelectrodes revealed that the oxygen concentrations in the mat were rarely more than 50% of air saturation and that the oxygen penetration depth was quite variable, ranging from <0.05 cm to several centimeters. The bulk pH of the mat ranged from 7.1 to 7.6. There appeared to be a correlation between the flow rates at different subsites of the mat and the morphotypes of the microorganisms and Fe oxides that developed. In subsites with low flow rates (<2 ml/s), the iron-encrusted sheaths of Leptothrix ochracea predominated. Miniature cores revealed that the top few millimeters of the mat consisted primarily of L. ochracea sheaths, only about 7% of which contained filaments of cells. Deeper in the mat, large particulate oxides developed, which were often heavily colonized by unicellular bacteria that were made visible by staining with acridine orange. Direct cell counts revealed that the number of bacteria increased from approximately 108 to 109 cells per cm3 and the total iron concentration increased from approximately 0.5 to 3 mmol/cm3 with depth in the mat. Primarily because of the growth of L. ochracea, the mat could accrete at rates of up to 3.1 mm/day at these subsites. The iron-encrusted stalks of Gallionella spp. prevailed in localized zones of the same low-flow-rate subsites, usually close to where the source water emanated from the wall. These latter zones had the lowest O2 concentrations (<10% of the ambient concentration), confirming the microaerobic nature of Gallionella spp. In subsites with high flow rates (>6 ml/s) particulate Fe oxides were dominant; direct counts revealed that up to 109 cells of primarily unicellular bacteria per cm3 were associated with these particulate oxides. These zones exhibited little vertical stratification in either the number of cells or iron concentration. Finally, mat samples incubated anaerobically in the presence of acetate or succinate exhibited significant potential for iron reduction, suggesting the possibility that a localized iron cycle could occur within the mat community.
Full text
PDF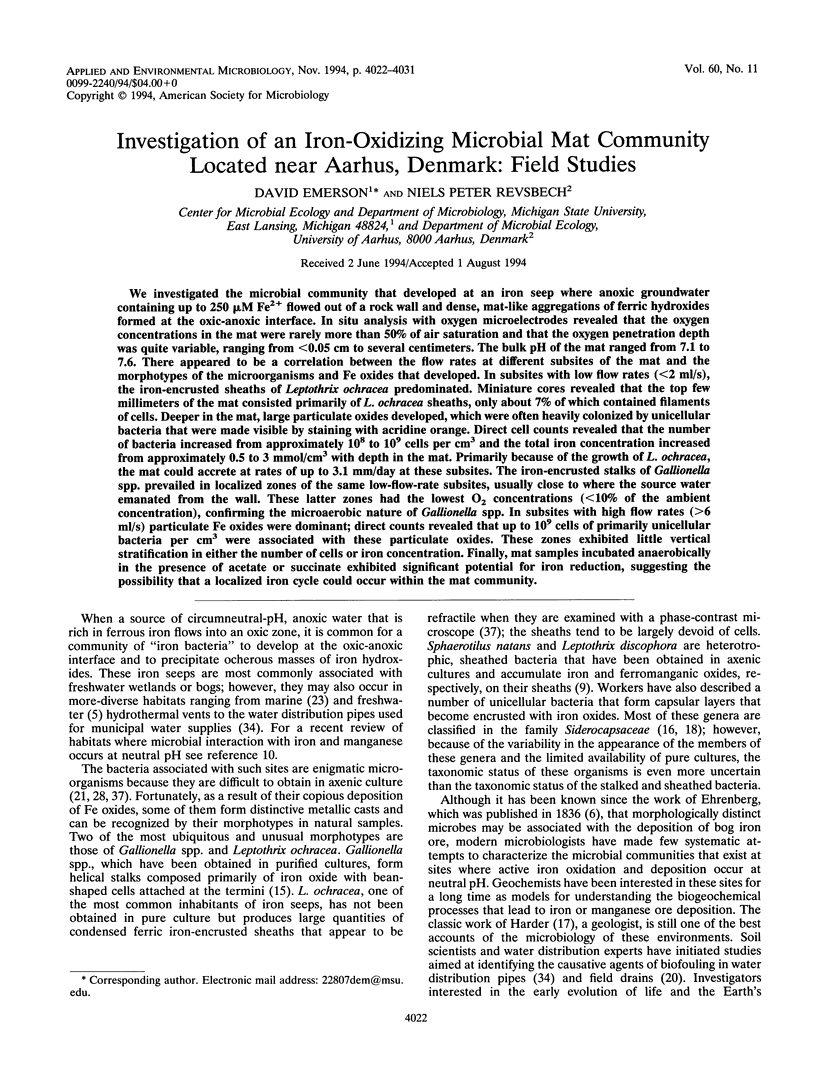
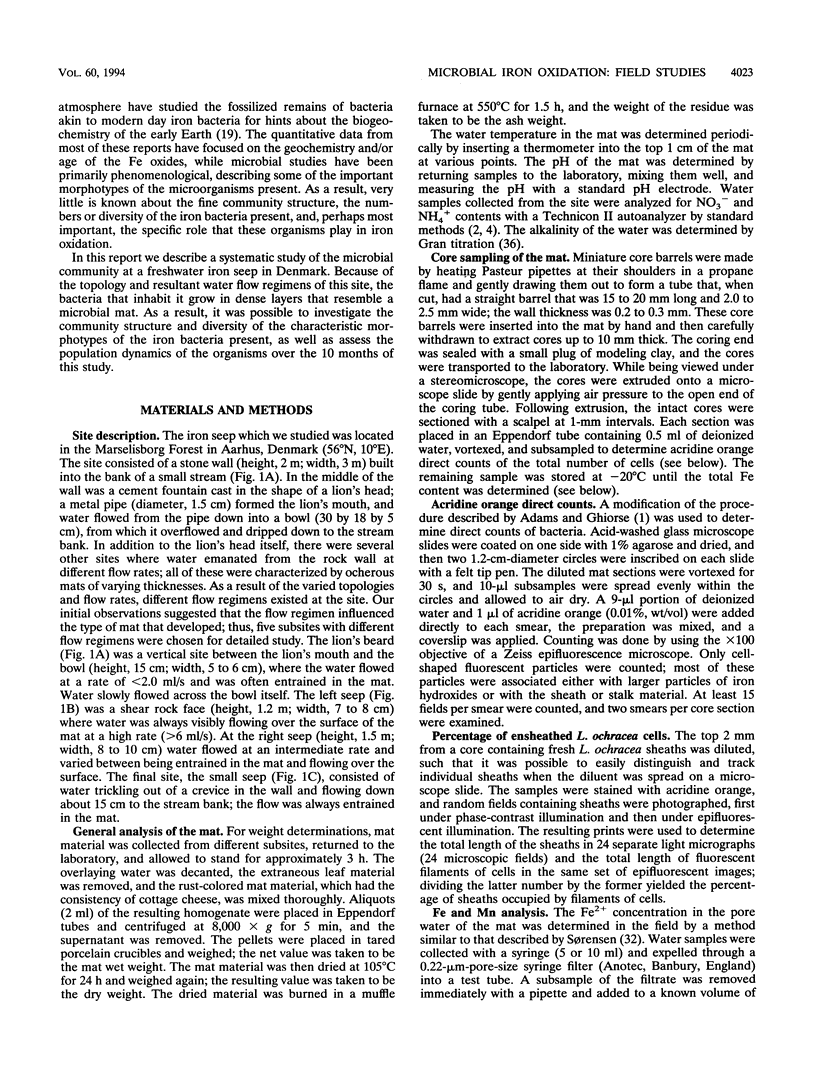
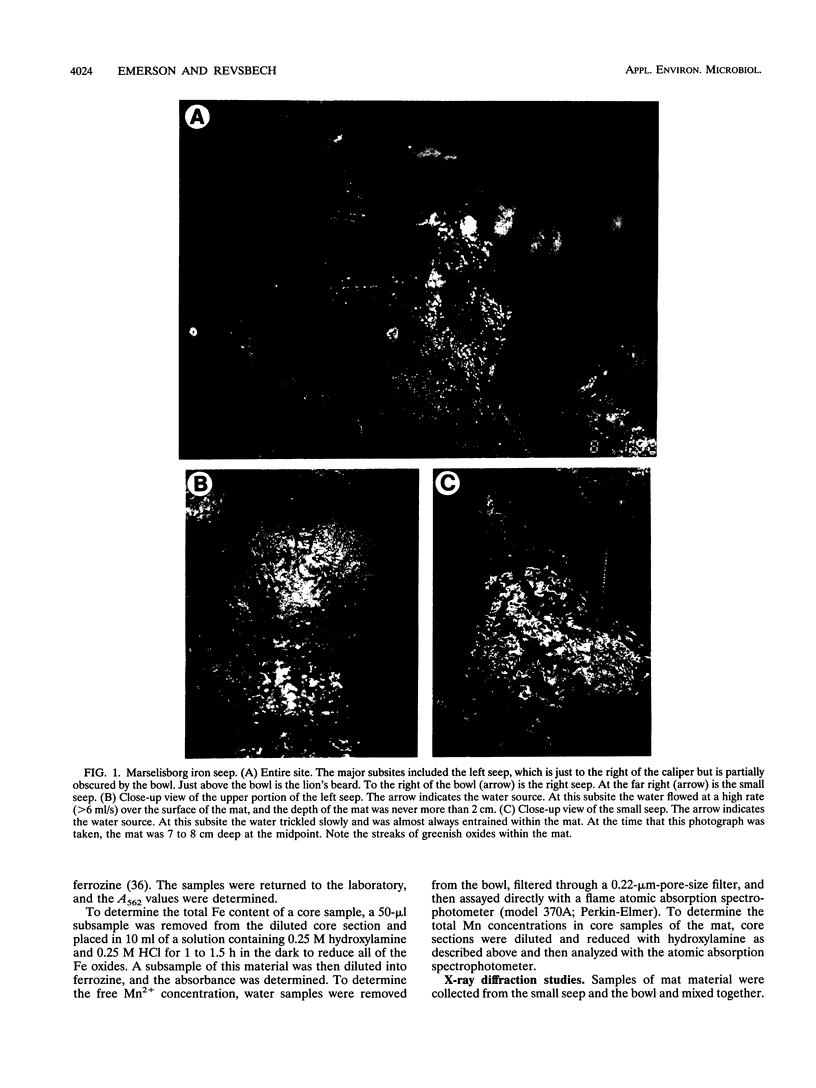
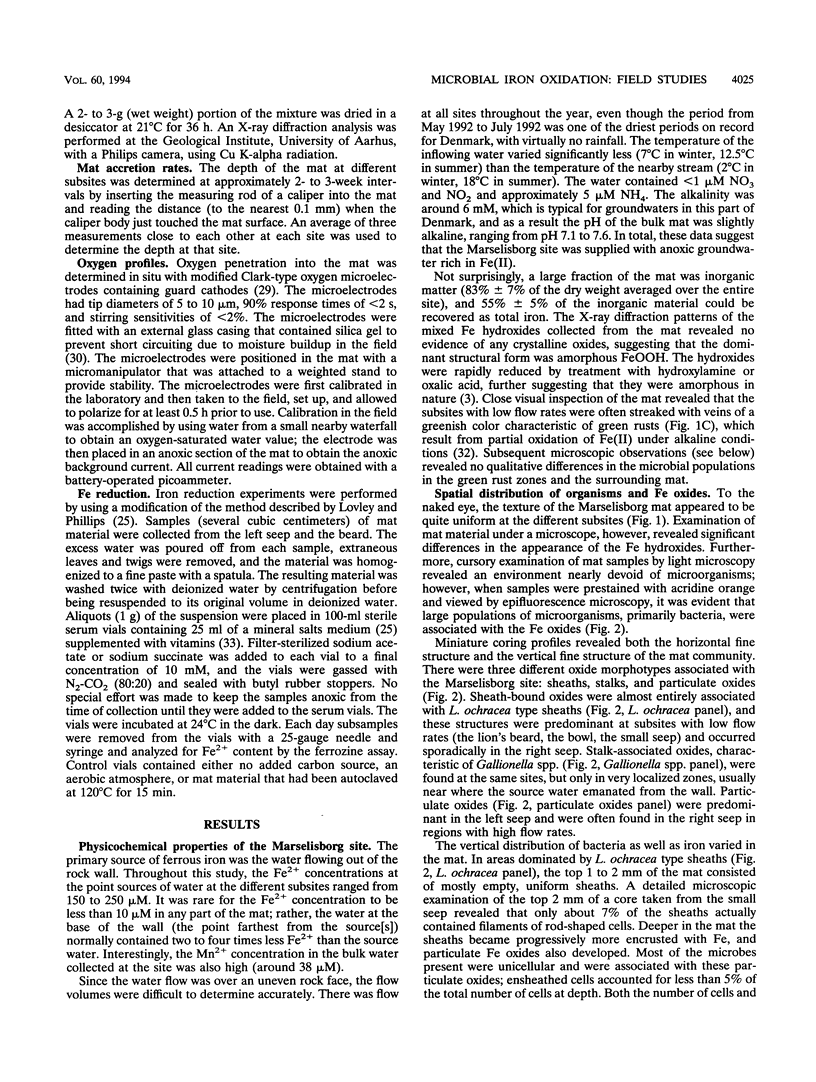
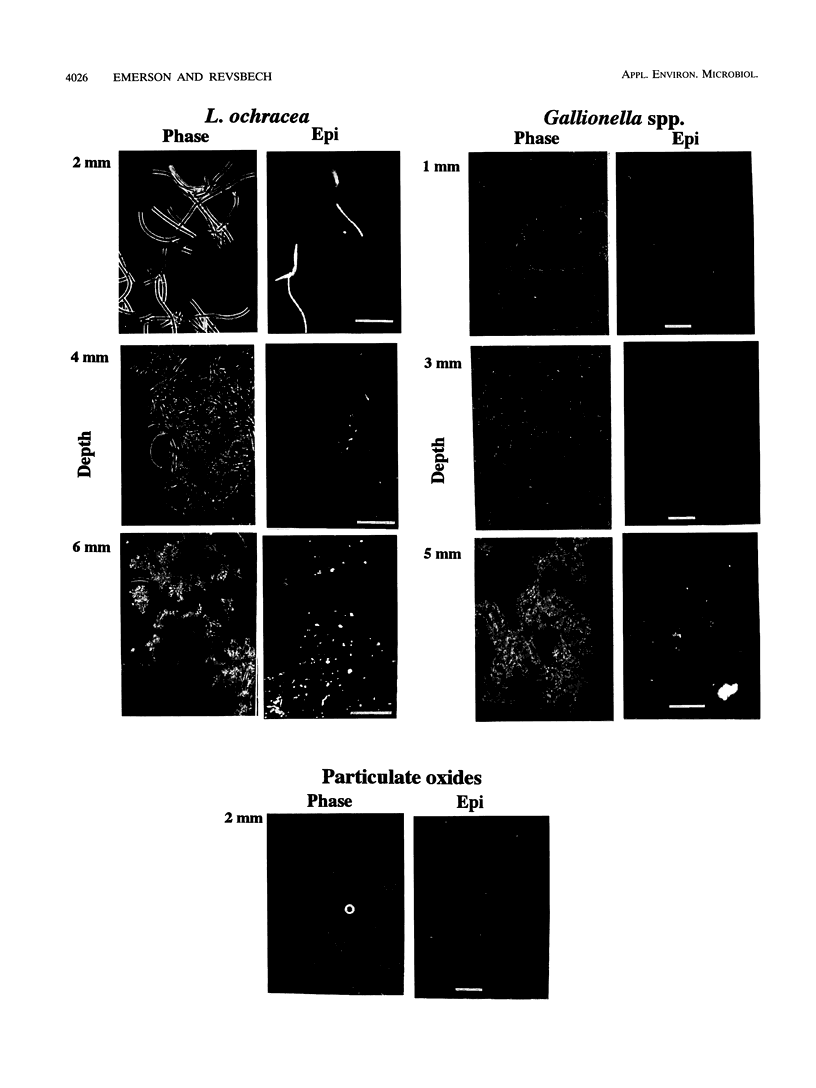
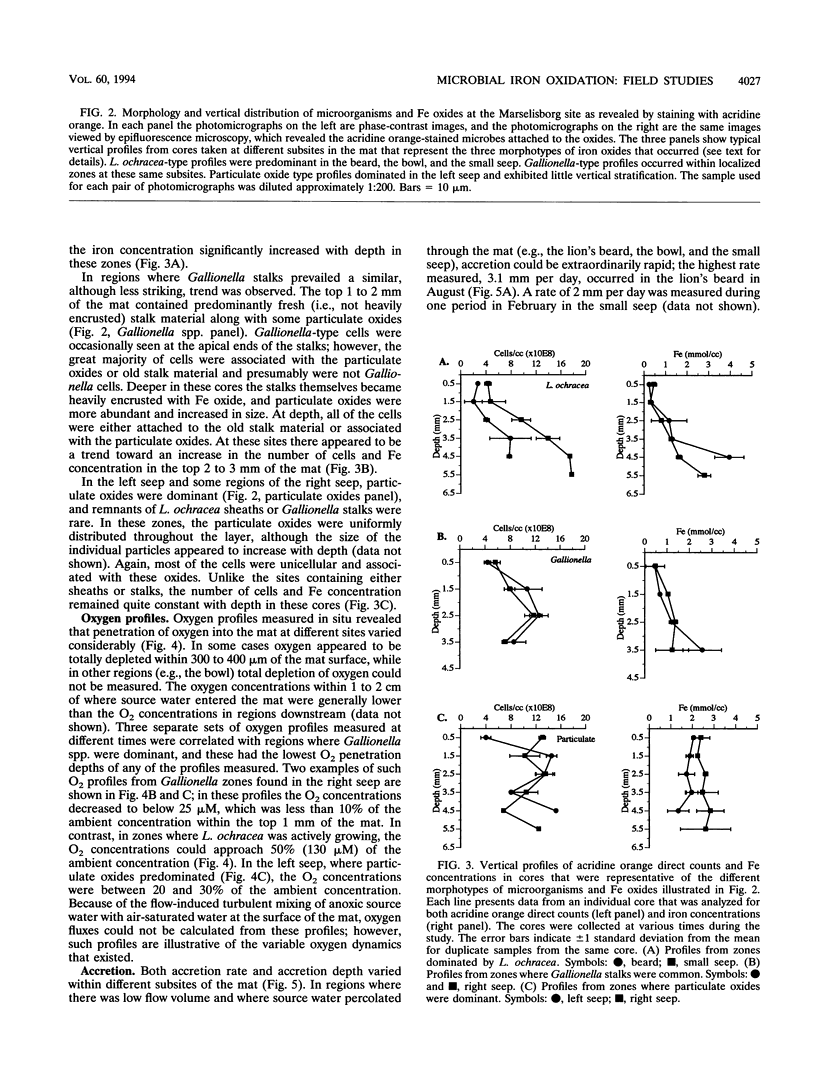
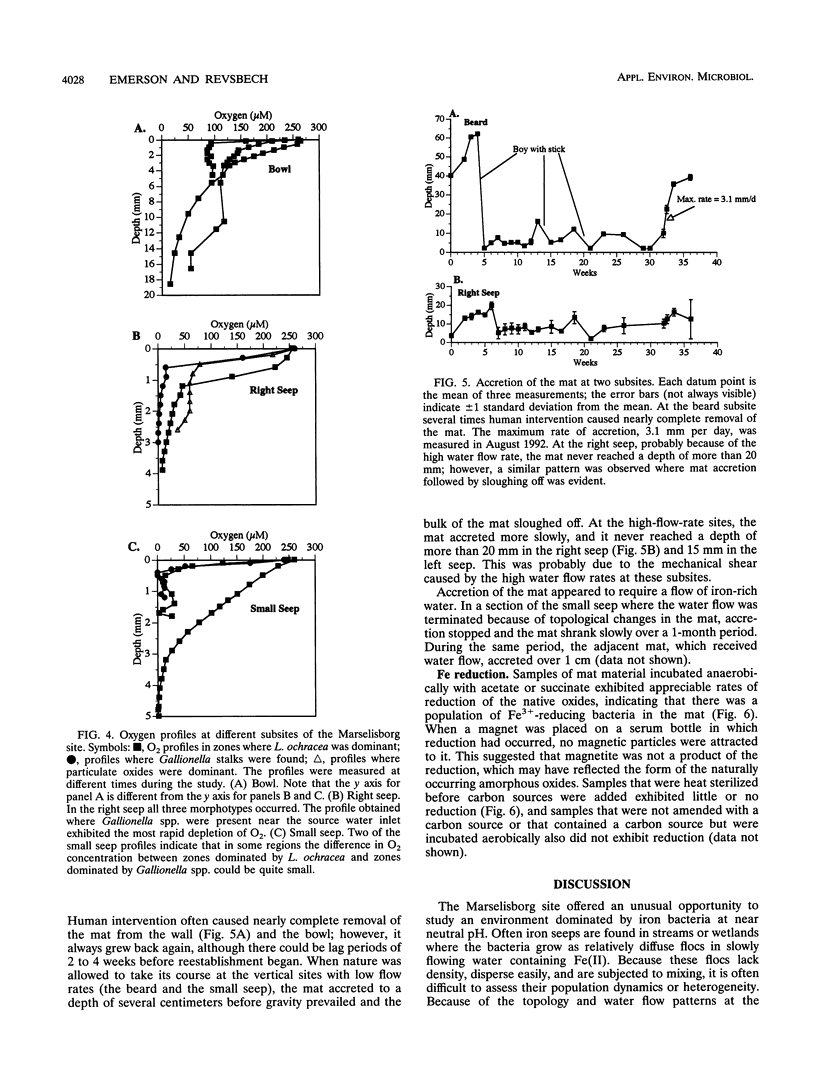
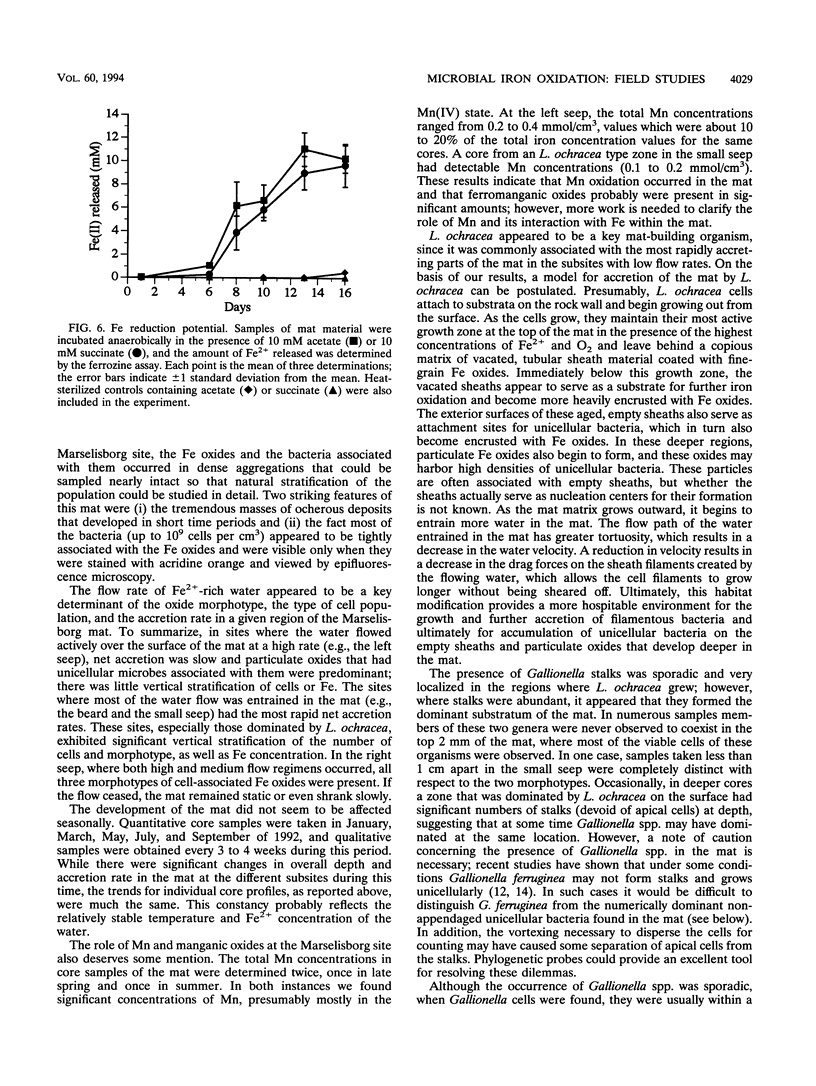
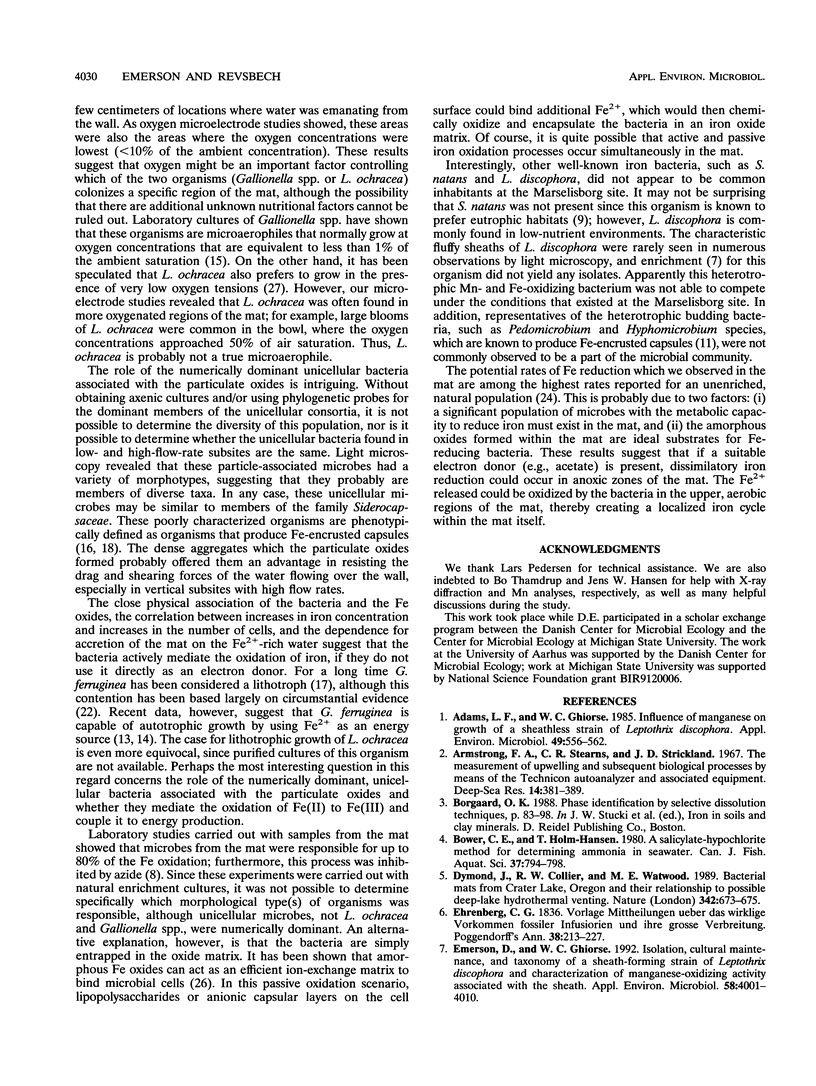
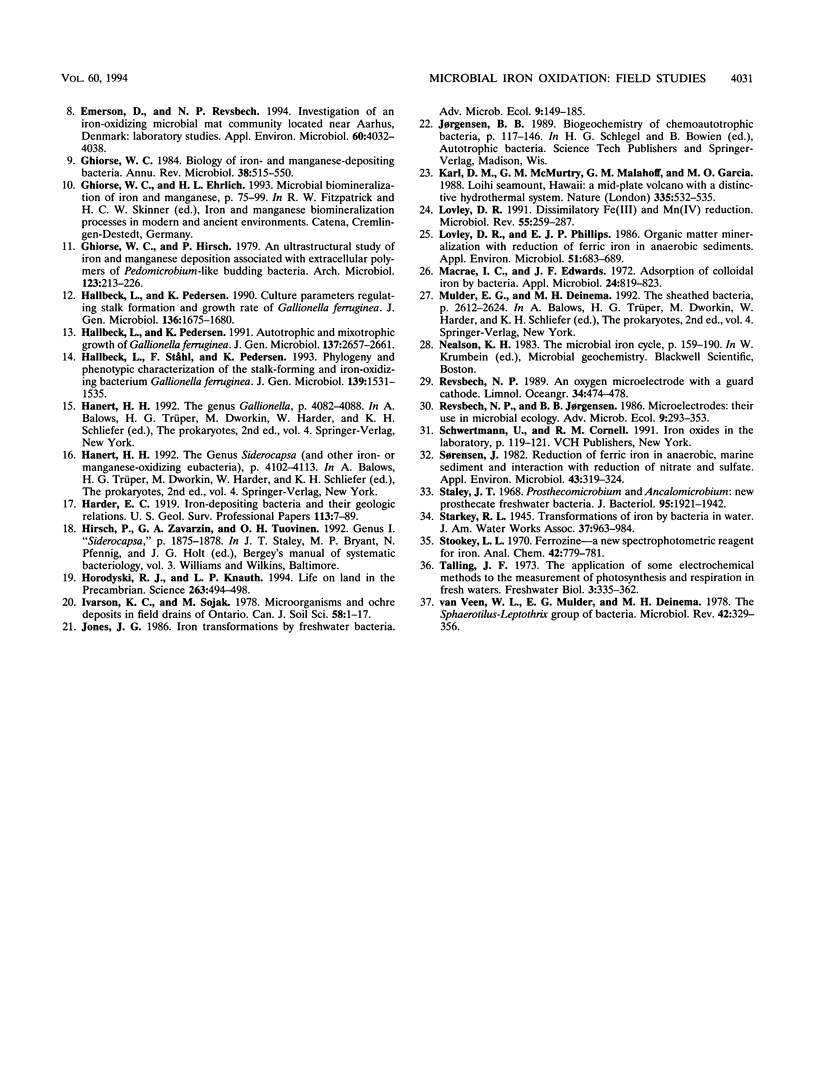
Images in this article
Selected References
These references are in PubMed. This may not be the complete list of references from this article.
- Adams L. F., Ghiorse W. C. Influence of Manganese on Growth of a Sheathless Strain of Leptothrix discophora. Appl Environ Microbiol. 1985 Mar;49(3):556–562. doi: 10.1128/aem.49.3.556-562.1985. [DOI] [PMC free article] [PubMed] [Google Scholar]
- Emerson D., Ghiorse W. C. Isolation, Cultural Maintenance, and Taxonomy of a Sheath-Forming Strain of Leptothrix discophora and Characterization of Manganese-Oxidizing Activity Associated with the Sheath. Appl Environ Microbiol. 1992 Dec;58(12):4001–4010. doi: 10.1128/aem.58.12.4001-4010.1992. [DOI] [PMC free article] [PubMed] [Google Scholar]
- Emerson D., Revsbech N. P. Investigation of an Iron-Oxidizing Microbial Mat Community Located near Aarhus, Denmark: Laboratory Studies. Appl Environ Microbiol. 1994 Nov;60(11):4032–4038. doi: 10.1128/aem.60.11.4032-4038.1994. [DOI] [PMC free article] [PubMed] [Google Scholar]
- Ghiorse W. C. Biology of iron- and manganese-depositing bacteria. Annu Rev Microbiol. 1984;38:515–550. doi: 10.1146/annurev.mi.38.100184.002503. [DOI] [PubMed] [Google Scholar]
- Hallbeck L., Ståhl F., Pedersen K. Phylogeny and phenotypic characterization of the stalk-forming and iron-oxidizing bacterium Gallionella ferruginea. J Gen Microbiol. 1993 Jul;139(7):1531–1535. doi: 10.1099/00221287-139-7-1531. [DOI] [PubMed] [Google Scholar]
- Horodyski R. J., Knauth L. P. Life on land in the precambrian. Science. 1994 Jan 28;263(5146):494–498. doi: 10.1126/science.263.5146.494. [DOI] [PubMed] [Google Scholar]
- Lovley D. R. Dissimilatory Fe(III) and Mn(IV) reduction. Microbiol Rev. 1991 Jun;55(2):259–287. doi: 10.1128/mr.55.2.259-287.1991. [DOI] [PMC free article] [PubMed] [Google Scholar]
- Lovley D. R., Phillips E. J. Organic matter mineralization with reduction of ferric iron in anaerobic sediments. Appl Environ Microbiol. 1986 Apr;51(4):683–689. doi: 10.1128/aem.51.4.683-689.1986. [DOI] [PMC free article] [PubMed] [Google Scholar]
- Macrae I. C., Edwards J. F. Adsorption of colloidal iron by bacteria. Appl Microbiol. 1972 Nov;24(5):819–823. doi: 10.1128/am.24.5.819-823.1972. [DOI] [PMC free article] [PubMed] [Google Scholar]
- Staley J. T. Prosthecomicrobium and Ancalomicrobium: new prosthecate freshwater bacteria. J Bacteriol. 1968 May;95(5):1921–1942. doi: 10.1128/jb.95.5.1921-1942.1968. [DOI] [PMC free article] [PubMed] [Google Scholar]
- Sørensen J. Reduction of ferric iron in anaerobic, marine sediment and interaction with reduction of nitrate and sulfate. Appl Environ Microbiol. 1982 Feb;43(2):319–324. doi: 10.1128/aem.43.2.319-324.1982. [DOI] [PMC free article] [PubMed] [Google Scholar]
- van Veen W. L., Mulder E. G., Deinema M. H. The Sphaerotilus-Leptothrix group of bacteria. Microbiol Rev. 1978 Jun;42(2):329–356. doi: 10.1128/mr.42.2.329-356.1978. [DOI] [PMC free article] [PubMed] [Google Scholar]