Abstract
Anoxic iron-rich sediment samples that had been stored in the light showed development of brown, rusty patches. Subcultures in defined mineral media with ferrous iron (10 mmol/liter, mostly precipitated as FeCO3) yielded enrichments of anoxygenic phototrophic bacteria which used ferrous iron as the sole electron donor for photosynthesis. Two different types of purple bacteria, represented by strains L7 and SW2, were isolated which oxidized colorless ferrous iron under anoxic conditions in the light to brown ferric iron. Strain L7 had rod-shaped, nonmotile cells (1.3 by 2 to 3 microns) which frequently formed gas vesicles. In addition to ferrous iron, strain L7 used H2 + CO2, acetate, pyruvate, and glucose as substrate for phototrophic growth. Strain SW2 had small rod-shaped, nonmotile cells (0.5 by 1 to 1.5 microns). Besides ferrous iron, strain SW2 utilized H2 + CO2, monocarboxylic acids, glucose, and fructose. Neither strain utilized free sulfide; however, both strains grew on black ferrous sulfide (FeS) which was converted to ferric iron and sulfate. Strains L7 and SW2 grown photoheterotrophically without ferrous iron were purple to brownish red and yellowish brown, respectively; absorption spectra revealed peaks characteristic of bacteriochlorophyll a. The closest phototrophic relatives of strains L7 and SW2 so far examined on the basis of 16S rRNA sequences were species of the genera Chromatium (gamma subclass of proteobacteria) and Rhodobacter (alpha subclass), respectively. In mineral medium, the new isolates formed 7.6 g of cell dry mass per mol of Fe(II) oxidized, which is in good agreement with a photoautotrophic utilization of ferrous iron as electron donor for CO2 fixation. Dependence of ferrous iron oxidation on light and CO2 was also demonstrated in dense cell suspensions. In media containing both ferrous iron and an organic substrate (e.g., acetate, glucose), strain L7 utilized ferrous iron and the organic compound simultaneously; in contrast, strain SW2 started to oxidize ferrous iron only after consumption of the organic electron donor. Ferrous iron oxidation by anoxygenic phototrophs is understandable in terms of energetics. In contrast to the Fe3+/Fe2+ pair (E0 = +0.77 V) existing in acidic solutions, the relevant redox pair at pH 7 in bicarbonate-containing environments, Fe(OH)3 + HCO3-/FeCO3, has an E0' of +0.2 V. Ferrous iron at pH 7 can therefore donate electrons to the photosystem of anoxygenic phototrophs, which in purple bacteria has a midpoint potential around +0.45 V. The existence of ferrous iron-oxidizing anoxygenic phototrophs may offer an explanation for the deposition of early banded-iron formations in an assumed anoxic biosphere in Archean times.
Full text
PDF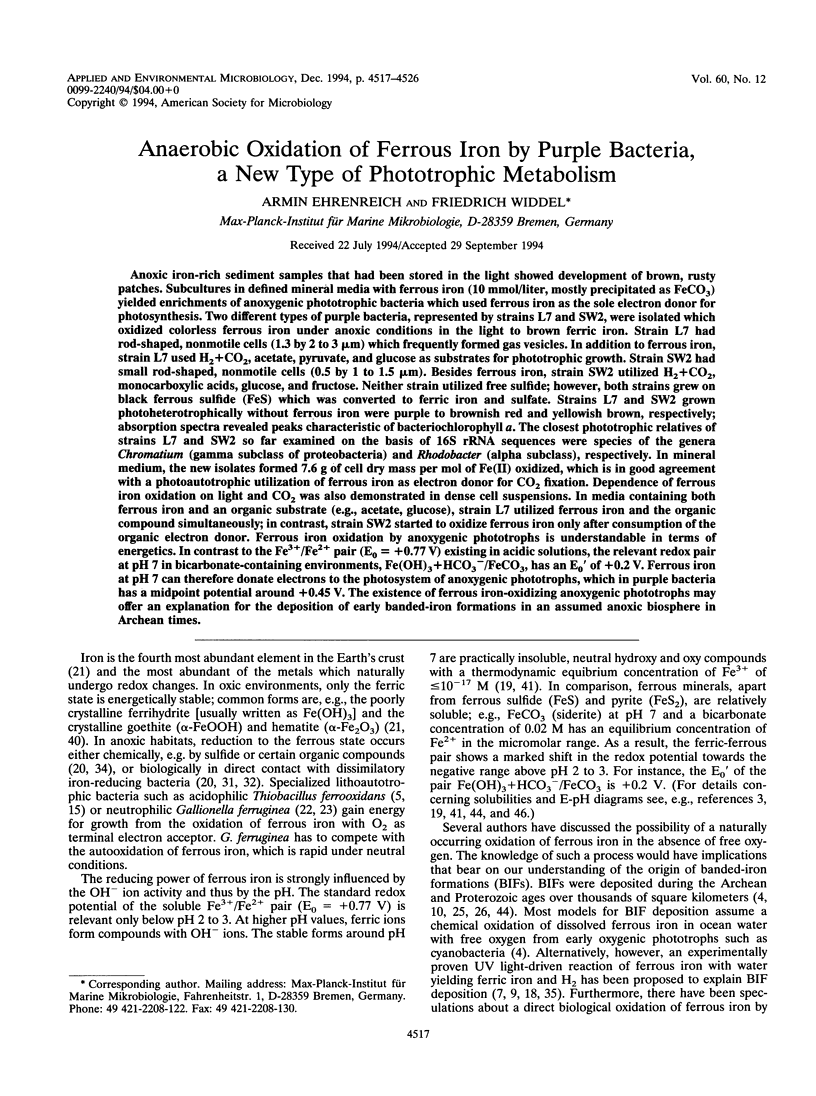
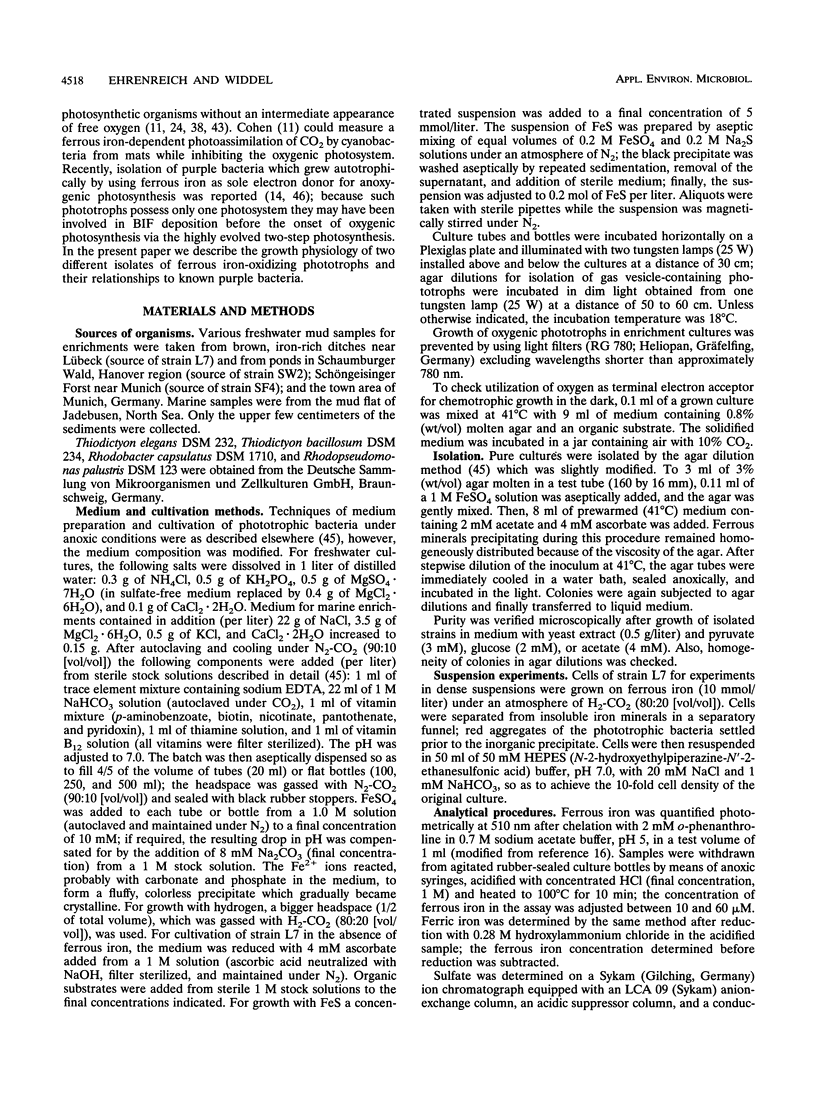
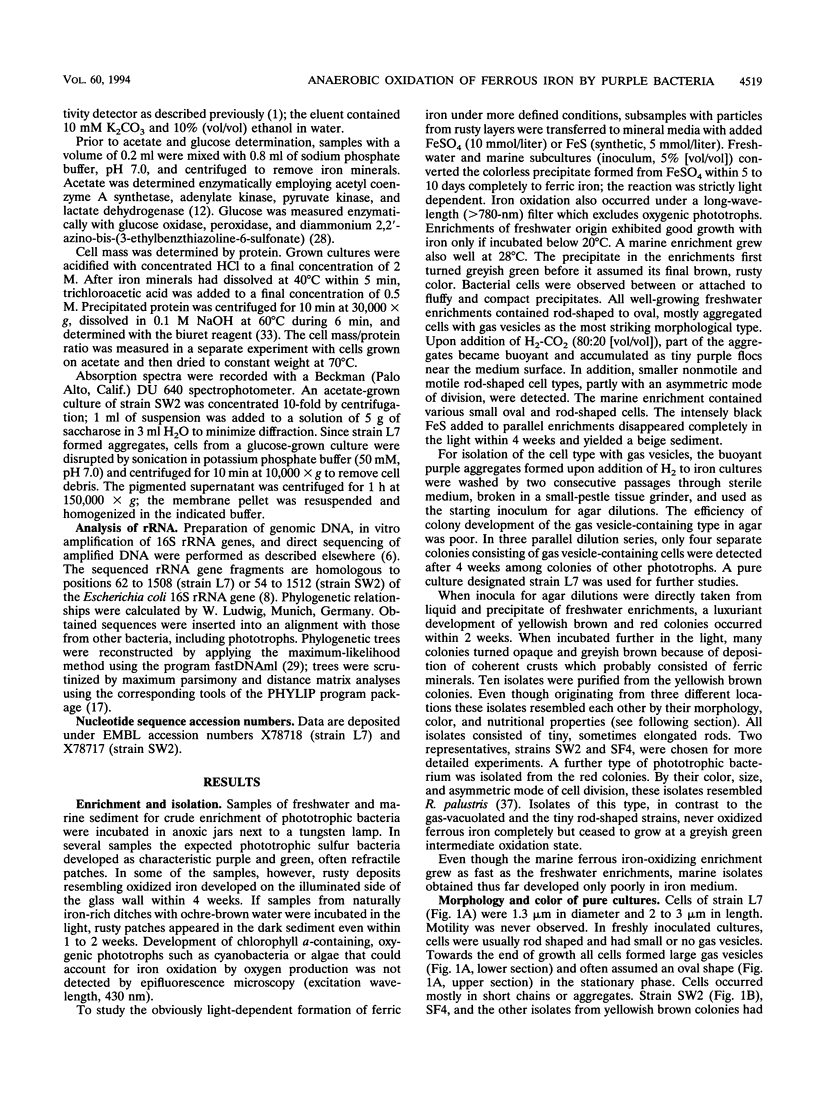
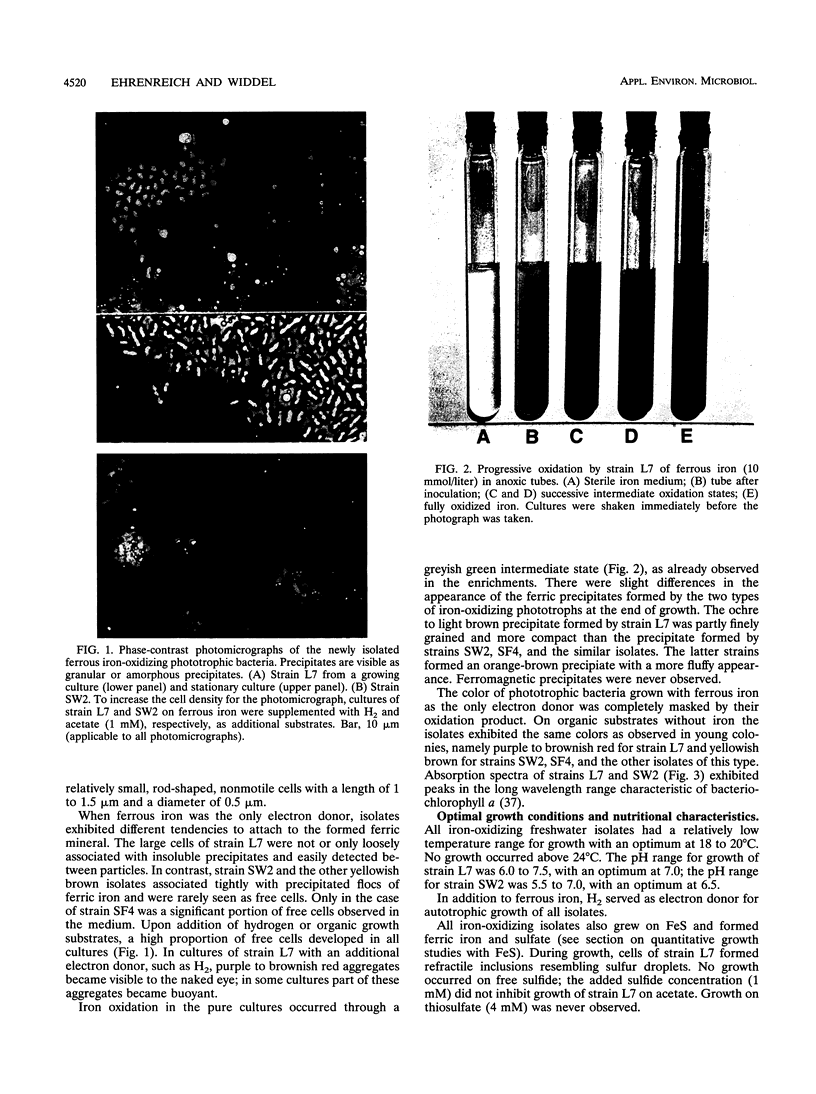
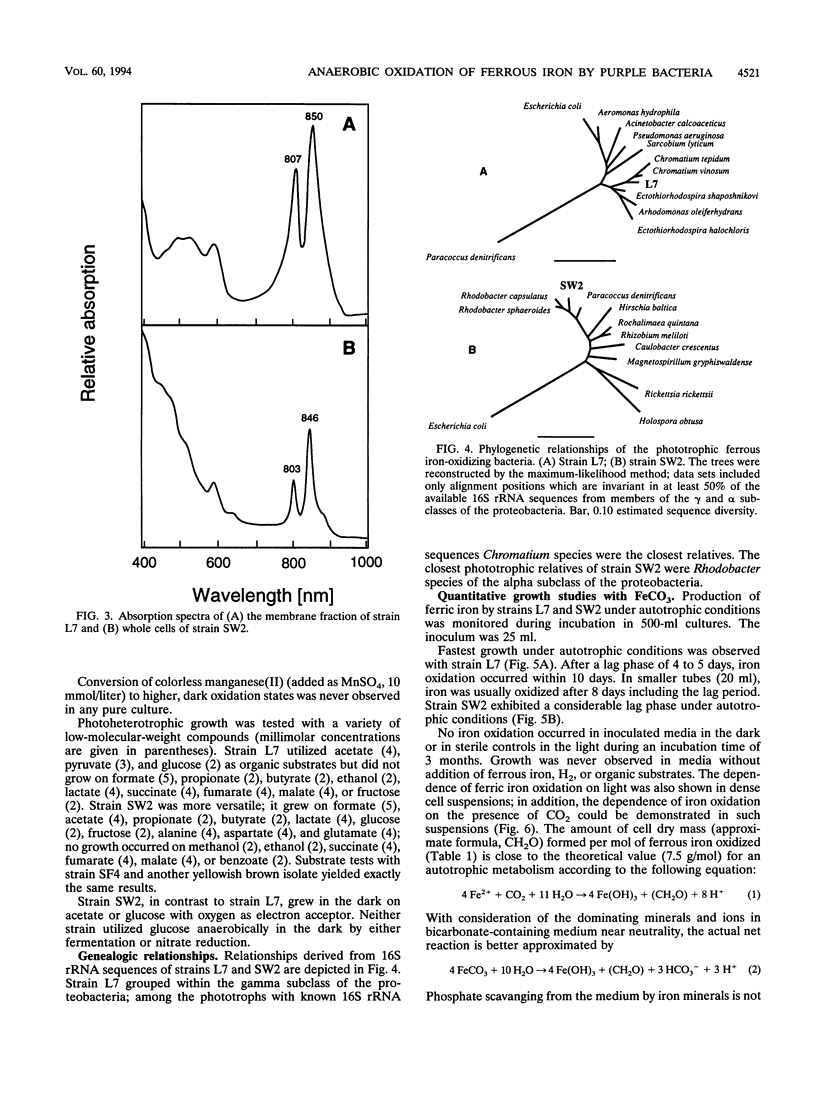
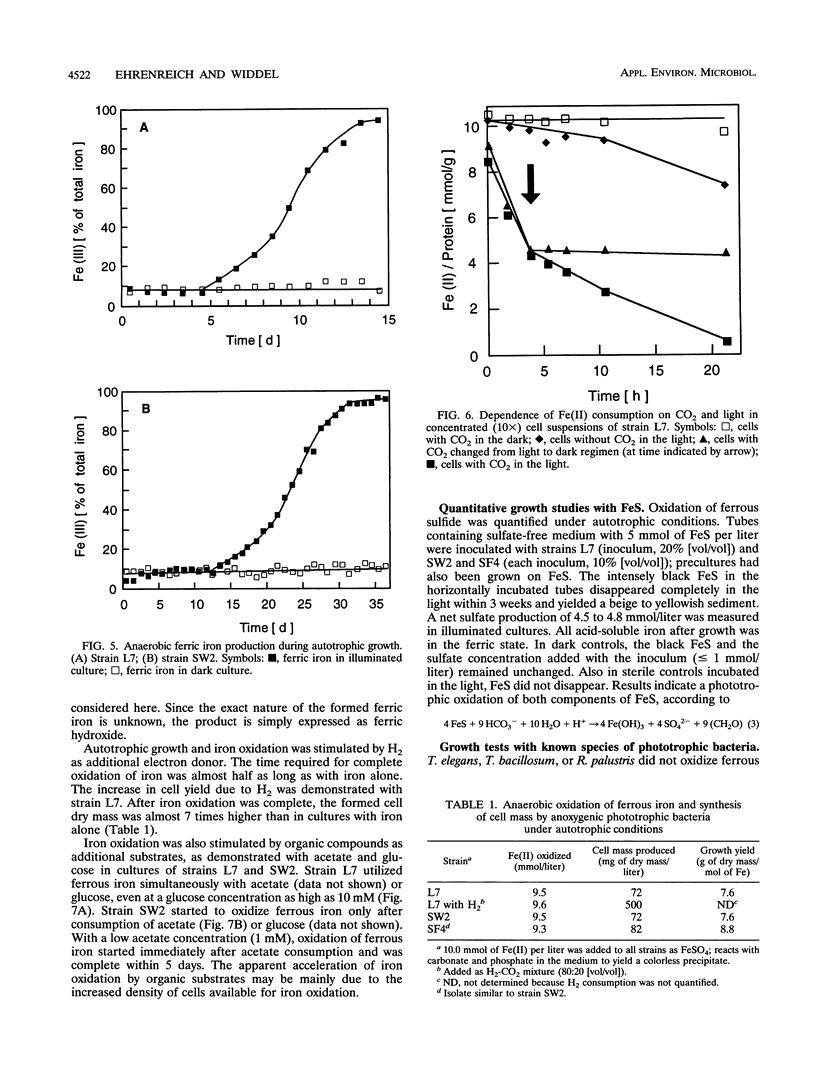
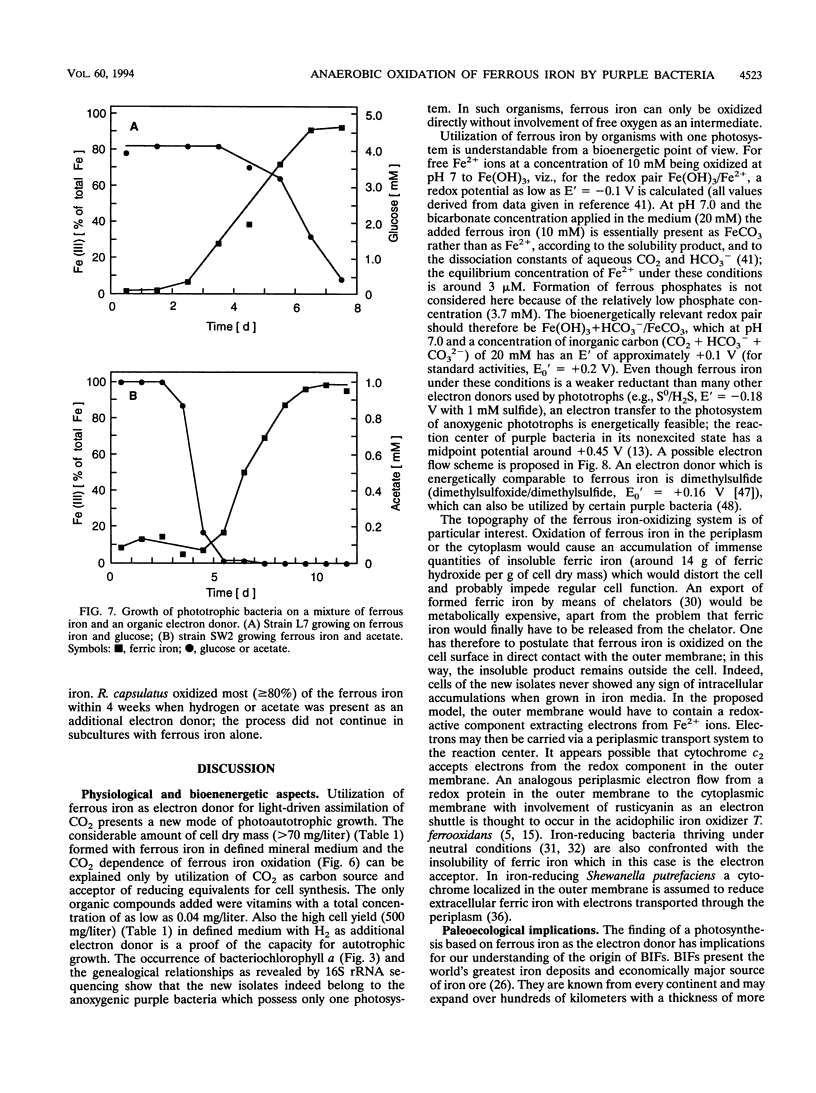
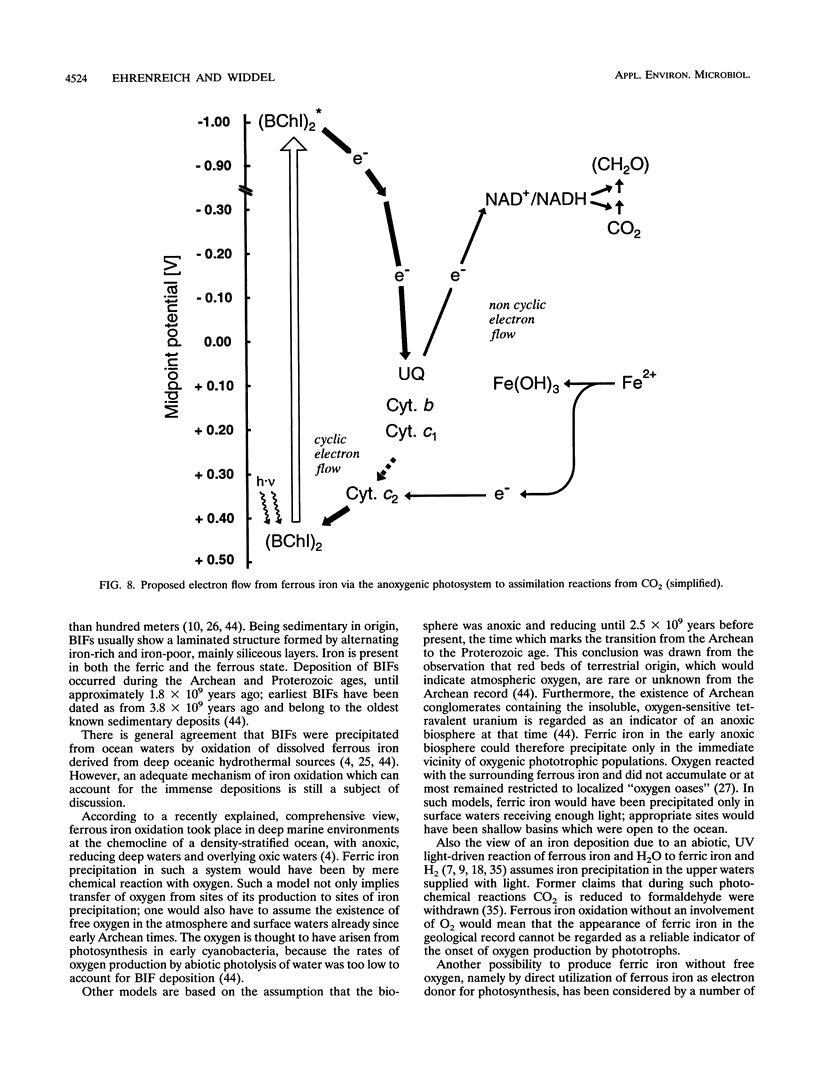
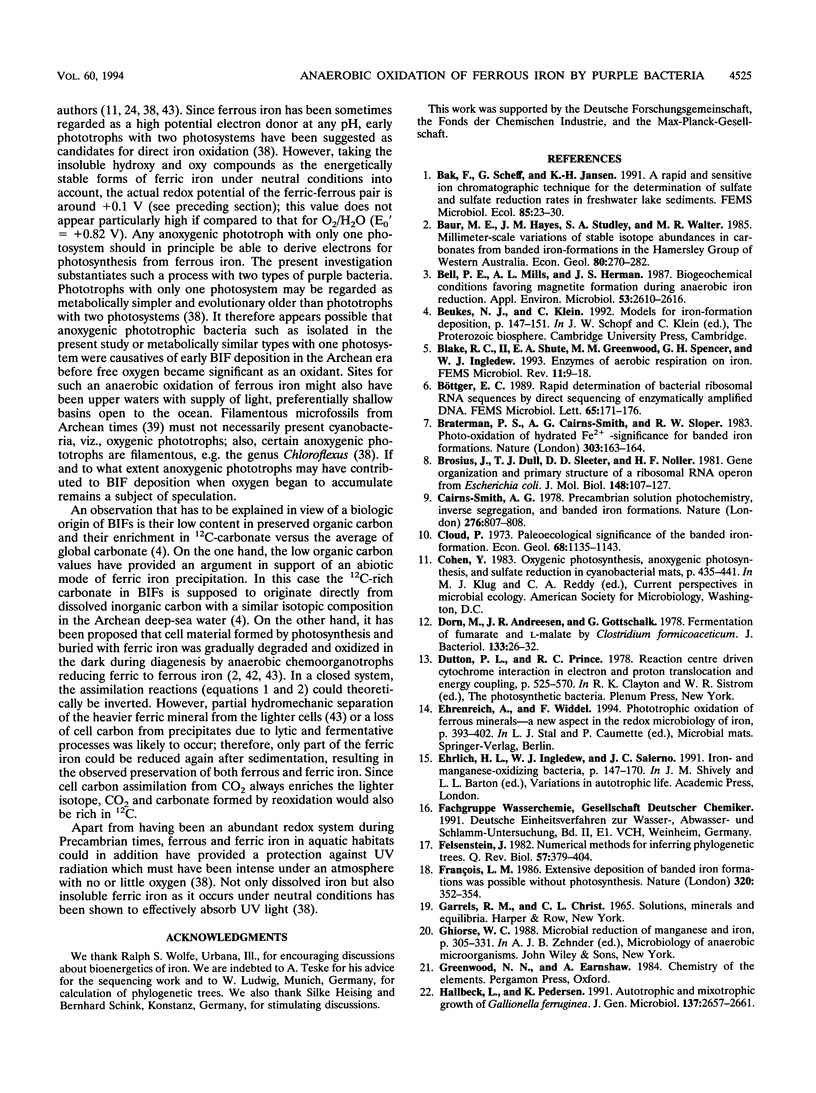
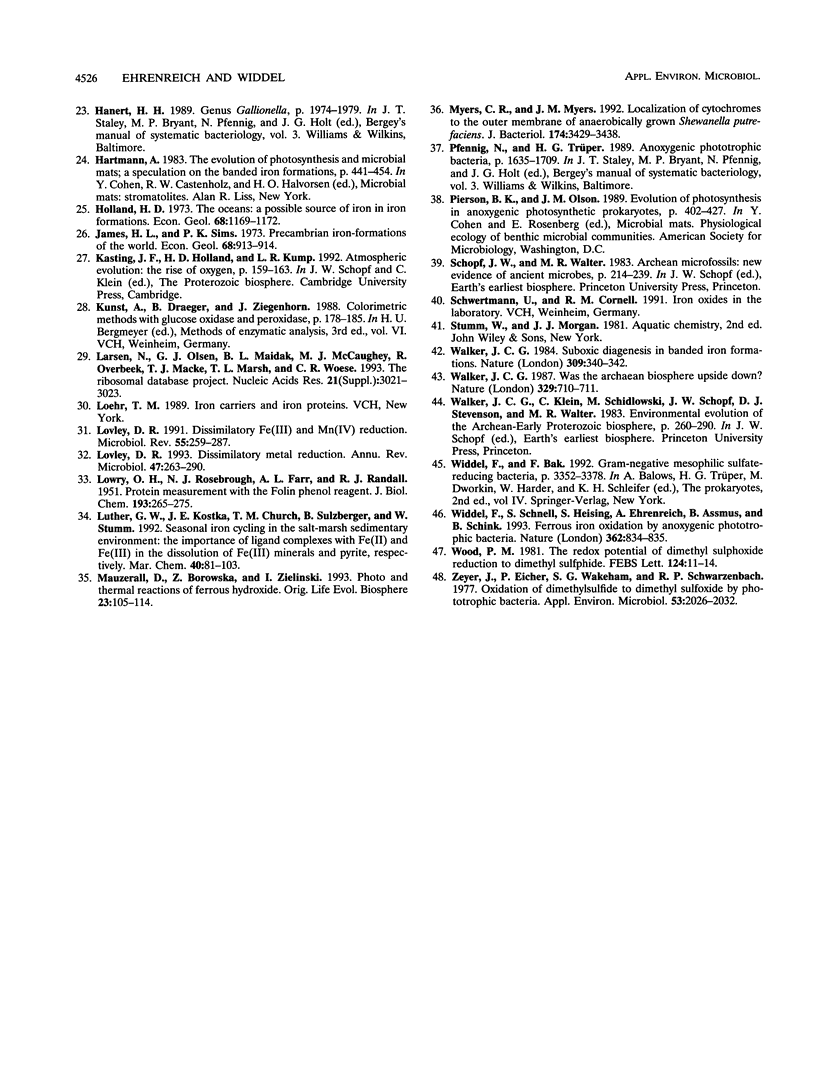
Images in this article
Selected References
These references are in PubMed. This may not be the complete list of references from this article.
- Baur M. E., Hayes J. M., Studley S. A., Walter M. R. Millimeter-scale variations of stable isotope abundances in carbonates from banded iron-formations in the Hamersley Group of Western Australia. Econ Geol. 1985;80:270–282. doi: 10.2113/gsecongeo.80.2.270. [DOI] [PubMed] [Google Scholar]
- Bell P. E., Mills A. L., Herman J. S. Biogeochemical Conditions Favoring Magnetite Formation during Anaerobic Iron Reduction. Appl Environ Microbiol. 1987 Nov;53(11):2610–2616. doi: 10.1128/aem.53.11.2610-2616.1987. [DOI] [PMC free article] [PubMed] [Google Scholar]
- Blake R. C., 2nd, Shute E. A., Greenwood M. M., Spencer G. H., Ingledew W. J. Enzymes of aerobic respiration on iron. FEMS Microbiol Rev. 1993 Jul;11(1-3):9–18. doi: 10.1111/j.1574-6976.1993.tb00261.x. [DOI] [PubMed] [Google Scholar]
- Brosius J., Dull T. J., Sleeter D. D., Noller H. F. Gene organization and primary structure of a ribosomal RNA operon from Escherichia coli. J Mol Biol. 1981 May 15;148(2):107–127. doi: 10.1016/0022-2836(81)90508-8. [DOI] [PubMed] [Google Scholar]
- Böttger E. C. Rapid determination of bacterial ribosomal RNA sequences by direct sequencing of enzymatically amplified DNA. FEMS Microbiol Lett. 1989 Nov;53(1-2):171–176. doi: 10.1016/0378-1097(89)90386-8. [DOI] [PubMed] [Google Scholar]
- Dorn M., Andreesen J. R., Gottschalk G. Fermentation of fumarate and L-malate by Clostridium formicoaceticum. J Bacteriol. 1978 Jan;133(1):26–32. doi: 10.1128/jb.133.1.26-32.1978. [DOI] [PMC free article] [PubMed] [Google Scholar]
- LOWRY O. H., ROSEBROUGH N. J., FARR A. L., RANDALL R. J. Protein measurement with the Folin phenol reagent. J Biol Chem. 1951 Nov;193(1):265–275. [PubMed] [Google Scholar]
- Larsen N., Olsen G. J., Maidak B. L., McCaughey M. J., Overbeek R., Macke T. J., Marsh T. L., Woese C. R. The ribosomal database project. Nucleic Acids Res. 1993 Jul 1;21(13):3021–3023. doi: 10.1093/nar/21.13.3021. [DOI] [PMC free article] [PubMed] [Google Scholar]
- Lovley D. R. Dissimilatory Fe(III) and Mn(IV) reduction. Microbiol Rev. 1991 Jun;55(2):259–287. doi: 10.1128/mr.55.2.259-287.1991. [DOI] [PMC free article] [PubMed] [Google Scholar]
- Lovley D. R. Dissimilatory metal reduction. Annu Rev Microbiol. 1993;47:263–290. doi: 10.1146/annurev.mi.47.100193.001403. [DOI] [PubMed] [Google Scholar]
- Myers C. R., Myers J. M. Localization of cytochromes to the outer membrane of anaerobically grown Shewanella putrefaciens MR-1. J Bacteriol. 1992 Jun;174(11):3429–3438. doi: 10.1128/jb.174.11.3429-3438.1992. [DOI] [PMC free article] [PubMed] [Google Scholar]
- Walker J. C. Suboxic diagenesis in banded iron formations. Nature. 1984 May 24;309:340–342. doi: 10.1038/309340a0. [DOI] [PubMed] [Google Scholar]
- Walker J. C. Was the Archaean biosphere upside down? Nature. 1987 Oct 22;329:710–712. doi: 10.1038/329710a0. [DOI] [PubMed] [Google Scholar]
- Wood P. M. The redox potential for dimethyl sulphoxide reduction to dimethyl sulphide: evaluation and biochemical implications. FEBS Lett. 1981 Feb 9;124(1):11–14. doi: 10.1016/0014-5793(81)80042-7. [DOI] [PubMed] [Google Scholar]
- Zeyer J., Eicher P., Wakeham S. G., Schwarzenbach R. P. Oxidation of dimethyl sulfide to dimethyl sulfoxide by phototrophic purple bacteria. Appl Environ Microbiol. 1987 Sep;53(9):2026–2032. doi: 10.1128/aem.53.9.2026-2032.1987. [DOI] [PMC free article] [PubMed] [Google Scholar]