Abstract
Homolactic fermentation of glucose and heterolactic fermentation of maltose with Lactococcus lactis 65.1 were confirmed. When moles of glucose were compared, the uptake rates of the two carbon sources were similar. The intracellular concentration of fructose-1,6-diphosphate (FDP) in maltose-assimilating cells was half of that in glucose-assimilating cells. Similarly, formation of FDP and lactate from maltose by extracts of maltose-grown cells was half of that formed from glucose by extracts of glucose-grown cells, indicating a difference in the utilization of the two carbon sources for energy metabolism. Concentrations of adenine nucleotides were similar in both types of cells. Glucose-1-phosphate was found in extracts of maltose-grown cells given maltose and, in addition, an inducible and low β-specific phosphoglucomutase activity was observed. β-Glucose-1-phosphate was not metabolized by cell extracts to either FDP or lactate, suggesting an alternative metabolic route. The amount of [14C]maltose incorporated into the cell material of maltose-grown cells was four times greater than that of [14C]glucose incorporated into the cell material of glucose-grown cells. The intracellular concentration of UTP was lower in maltose-assimilating cells than in glucose-assimilating cells. Cells grown on maltose were more spherical and less fragile than cells grown on glucose.
Full text
PDF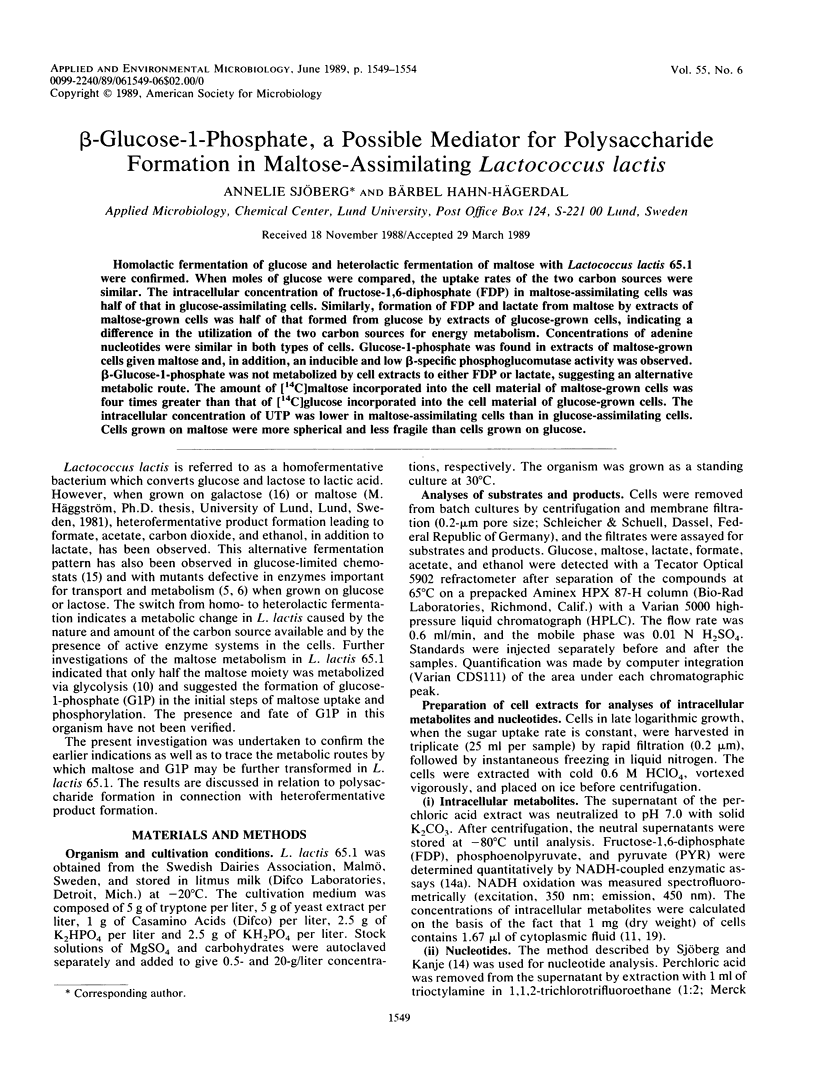
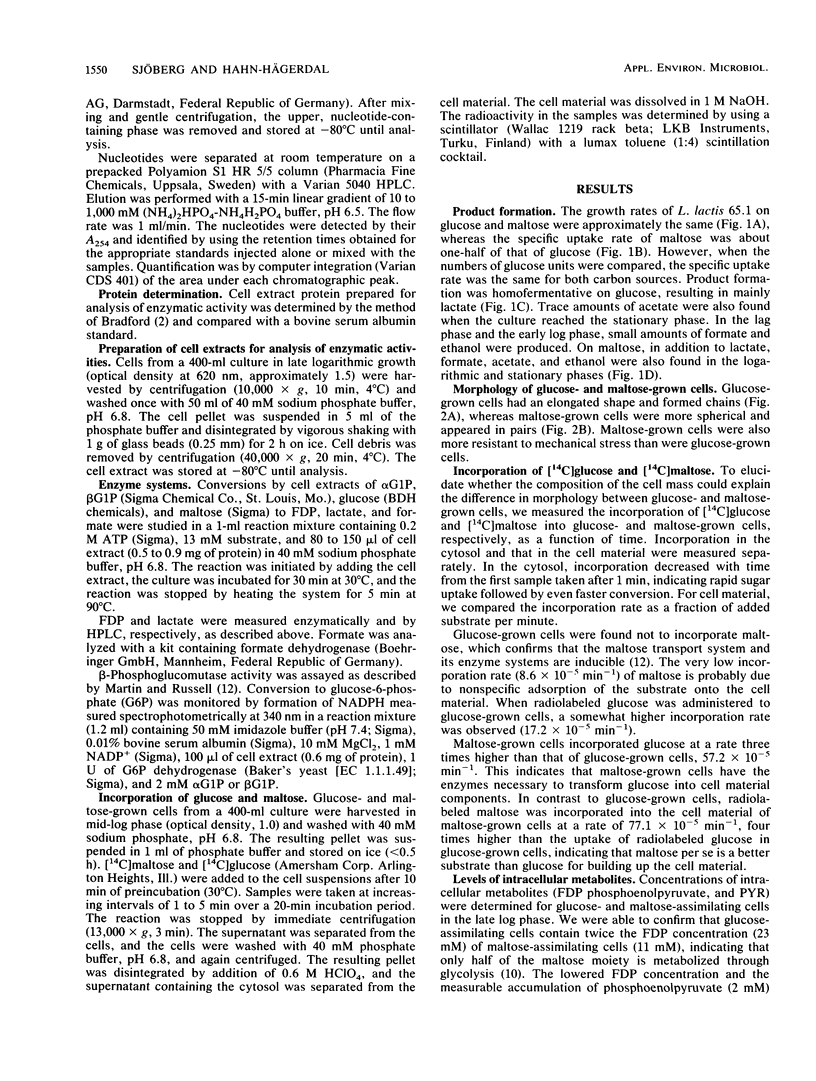
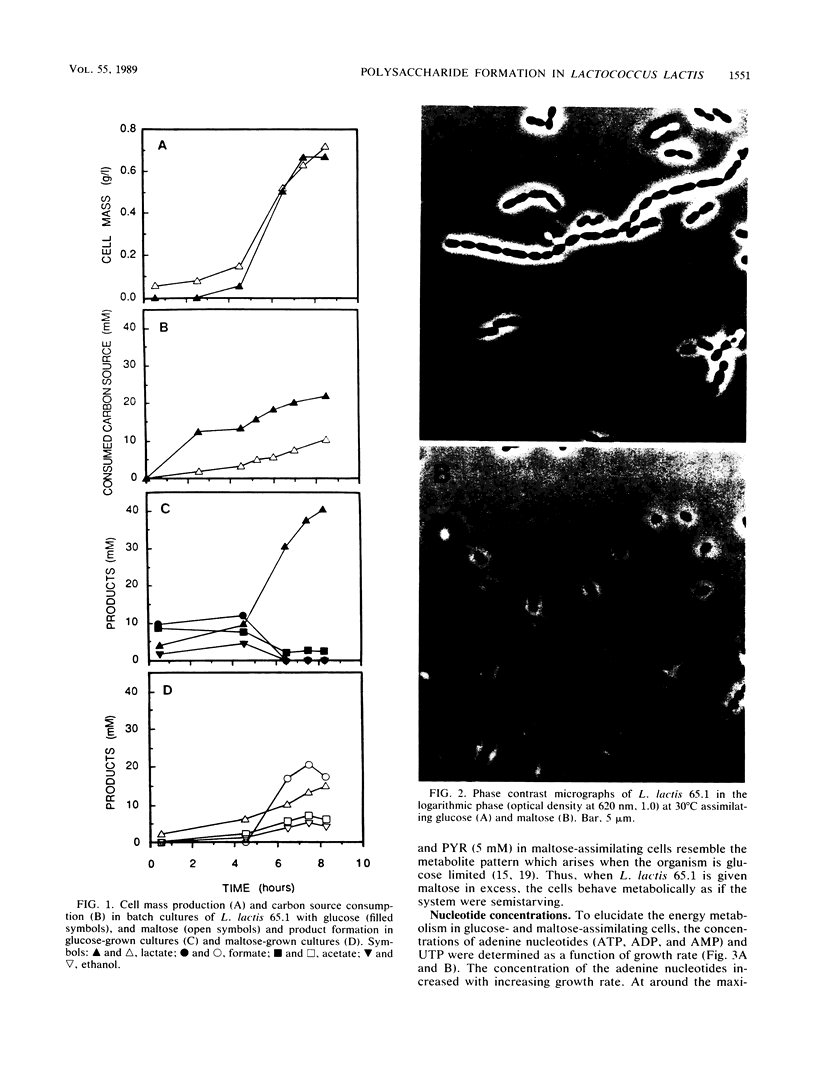
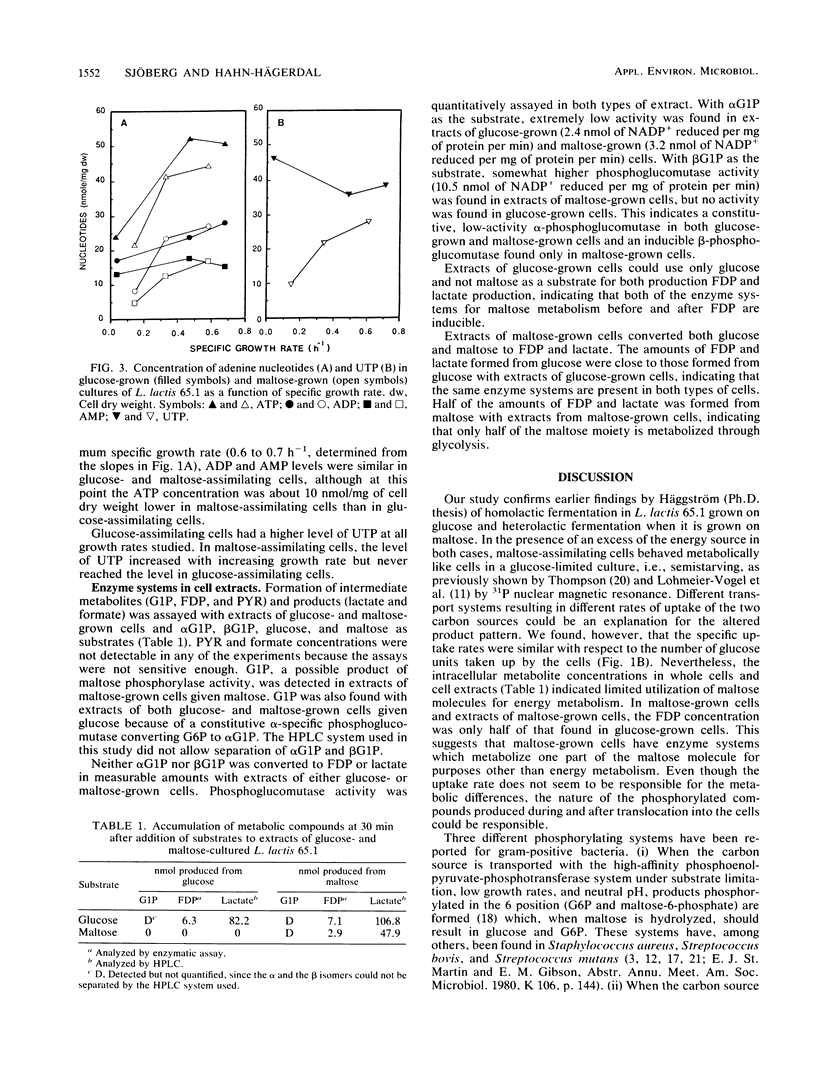
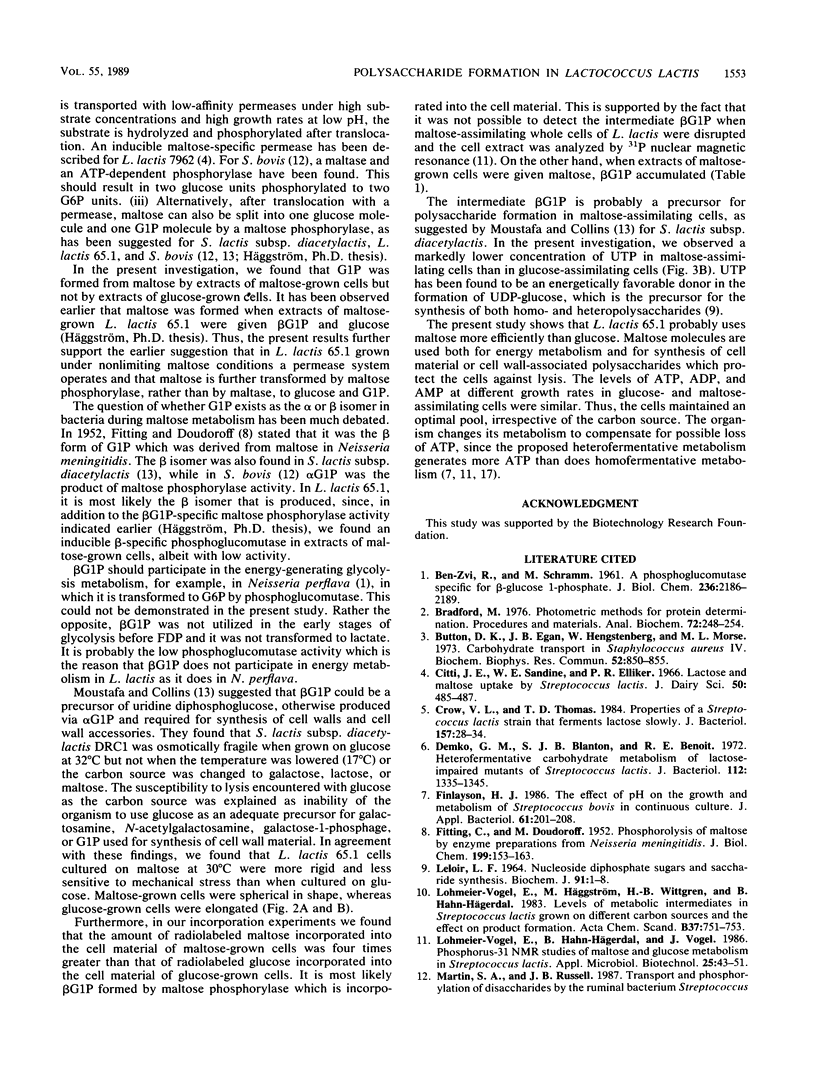
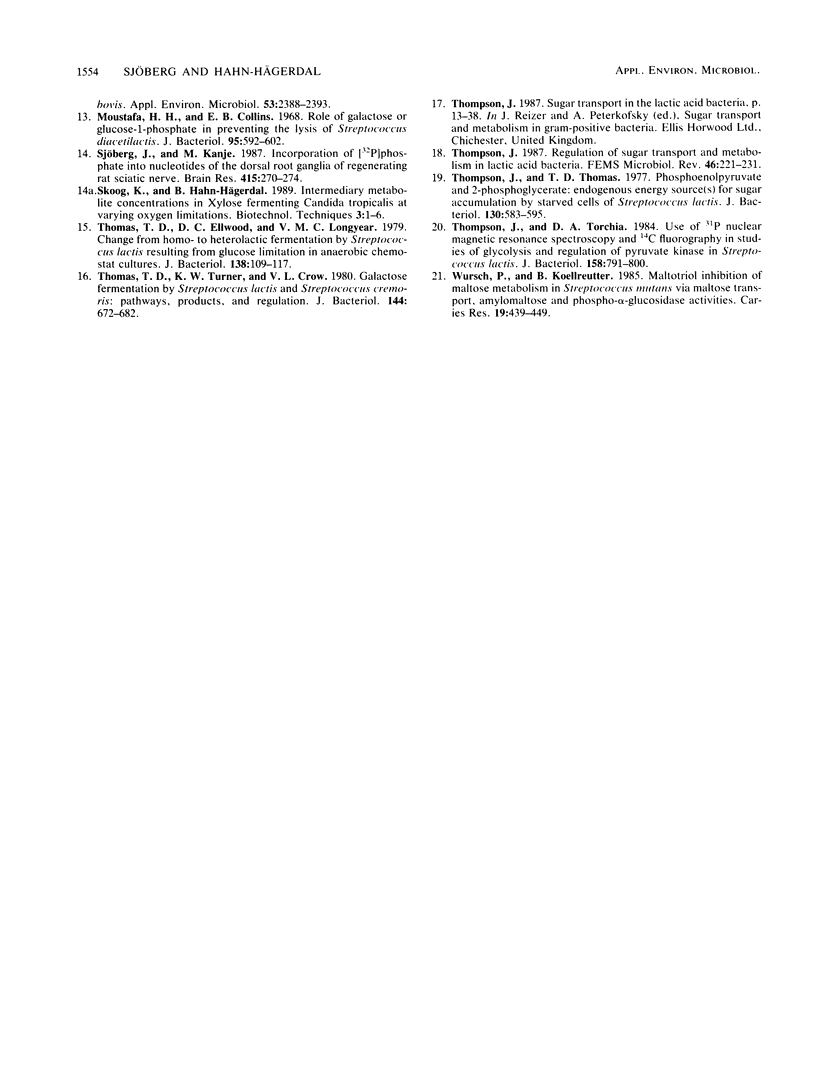
Images in this article
Selected References
These references are in PubMed. This may not be the complete list of references from this article.
- Bradford M. M. A rapid and sensitive method for the quantitation of microgram quantities of protein utilizing the principle of protein-dye binding. Anal Biochem. 1976 May 7;72:248–254. doi: 10.1006/abio.1976.9999. [DOI] [PubMed] [Google Scholar]
- Button D. K., Egan J. B., Hengstenberg W., Morse M. L. Carbohydrate transport in Staphylococcus aureus. IV. Maltose accumulation and metabolism. Biochem Biophys Res Commun. 1973 Jun 8;52(3):850–855. doi: 10.1016/0006-291x(73)91015-2. [DOI] [PubMed] [Google Scholar]
- Citti J. E., Sandine W. E., Elliker P. R. Lactose and maltose uptake by Streptococcus lactis. J Dairy Sci. 1967 Apr;50(4):485–487. doi: 10.3168/jds.S0022-0302(67)87451-4. [DOI] [PubMed] [Google Scholar]
- Crow V. L., Thomas T. D. Properties of a Streptococcus lactis strain that ferments lactose slowly. J Bacteriol. 1984 Jan;157(1):28–34. doi: 10.1128/jb.157.1.28-34.1984. [DOI] [PMC free article] [PubMed] [Google Scholar]
- Demko G. M., Blanton S. J., Benoit R. E. Heterofermentative carbohydrate metabolism of lactose-impaired mutants of Streptococcus lactis. J Bacteriol. 1972 Dec;112(3):1335–1345. doi: 10.1128/jb.112.3.1335-1345.1972. [DOI] [PMC free article] [PubMed] [Google Scholar]
- FITTING C., DOUDOROFF M. Phosphorolysis of maltose by enzyme preparations from Neisseria meningitidis. J Biol Chem. 1952 Nov;199(1):153–163. [PubMed] [Google Scholar]
- Finlayson H. J. The effect of pH on the growth and metabolism of Streptococcus bovis in continuous culture. J Appl Bacteriol. 1986 Sep;61(3):201–208. doi: 10.1111/j.1365-2672.1986.tb04277.x. [DOI] [PubMed] [Google Scholar]
- Leloir L. F. Nucleoside diphosphate sugars and saccharide synthesis. Biochem J. 1964 Apr;91(1):1–8. [PubMed] [Google Scholar]
- Martin S. A., Russell J. B. Transport and phosphorylation of disaccharides by the ruminal bacterium Streptococcus bovis. Appl Environ Microbiol. 1987 Oct;53(10):2388–2393. doi: 10.1128/aem.53.10.2388-2393.1987. [DOI] [PMC free article] [PubMed] [Google Scholar]
- Moustafa H. H., Collins E. B. Role of galactose or glucose-1-phosphate in preventing the lysis of Streptococcus diacetilactis. J Bacteriol. 1968 Feb;95(2):592–602. doi: 10.1128/jb.95.2.592-602.1968. [DOI] [PMC free article] [PubMed] [Google Scholar]
- Sjöberg J., Kanje M. Incorporation of [32P]phosphate into nucleotides of the dorsal root ganglia of regenerating rat sciatic nerve. Brain Res. 1987 Jul 14;415(2):270–274. doi: 10.1016/0006-8993(87)90208-3. [DOI] [PubMed] [Google Scholar]
- Thomas T. D., Ellwood D. C., Longyear V. M. Change from homo- to heterolactic fermentation by Streptococcus lactis resulting from glucose limitation in anaerobic chemostat cultures. J Bacteriol. 1979 Apr;138(1):109–117. doi: 10.1128/jb.138.1.109-117.1979. [DOI] [PMC free article] [PubMed] [Google Scholar]
- Thomas T. D., Turner K. W., Crow V. L. Galactose fermentation by Streptococcus lactis and Streptococcus cremoris: pathways, products, and regulation. J Bacteriol. 1980 Nov;144(2):672–682. doi: 10.1128/jb.144.2.672-682.1980. [DOI] [PMC free article] [PubMed] [Google Scholar]
- Thompson J., Thomas T. D. Phosphoenolpyruvate and 2-phosphoglycerate: endogenous energy source(s) for sugar accumulation by starved cells of Streptococcus lactis. J Bacteriol. 1977 May;130(2):583–595. doi: 10.1128/jb.130.2.583-595.1977. [DOI] [PMC free article] [PubMed] [Google Scholar]
- Thompson J., Torchia D. A. Use of 31P nuclear magnetic resonance spectroscopy and 14C fluorography in studies of glycolysis and regulation of pyruvate kinase in Streptococcus lactis. J Bacteriol. 1984 Jun;158(3):791–800. doi: 10.1128/jb.158.3.791-800.1984. [DOI] [PMC free article] [PubMed] [Google Scholar]
- Würsch P., Koellreutter B. Maltotriitol inhibition of maltose metabolism in Streptococcus mutans via maltose transport, amylomaltase and phospho-alpha-glucosidase activities. Caries Res. 1985;19(5):439–449. doi: 10.1159/000260879. [DOI] [PubMed] [Google Scholar]