Abstract
Three strains of Streptococcus lactis, two of Streptococcus cremoris, and one of Streptococcus thermophilus metabolized oxygen in the presence of added carbohydrate primarily via a closely coupled NADH oxidase/NADH peroxidase system. No buildup of the toxic intermediate H2O2 was detected with the three S. lactis strains. All six strains contained significant superoxide dismutase activity and are clearly aerotolerant. Lactose- or glucose-driven oxygen consumption was biphasic, with a rapid initial rate followed by a slower secondary rate which correlated with factors affecting the in vivo activation of lactate dehydrogenase. The rate of oxygen consumption was rapid under conditions that led to a reduction in lactate dehydrogenase activity (low intracellular fructose 1,6-bisphosphate concentration). These conditions could be achieved with nongrowing cells by adding lactose at a constant but limiting rate. When the rate of lactose fermentation was limited to 5% of its maximum, nongrowing cells of S. lactis strains ML3 and ML8 carried out an essentially homoacetic fermentation under aerobic conditions. These same cells carried out the expected homolactic fermentation when presented with excess lactose under anaerobic conditions. Homoacetic fermentation leads to the generation of more energy, by substrate-level phosphorylation via acetate kinase, than the homolactic fermentation. However, it was not observed in growing cells and was restricted to slow fermentation rates with nongrowing cells.
Full text
PDF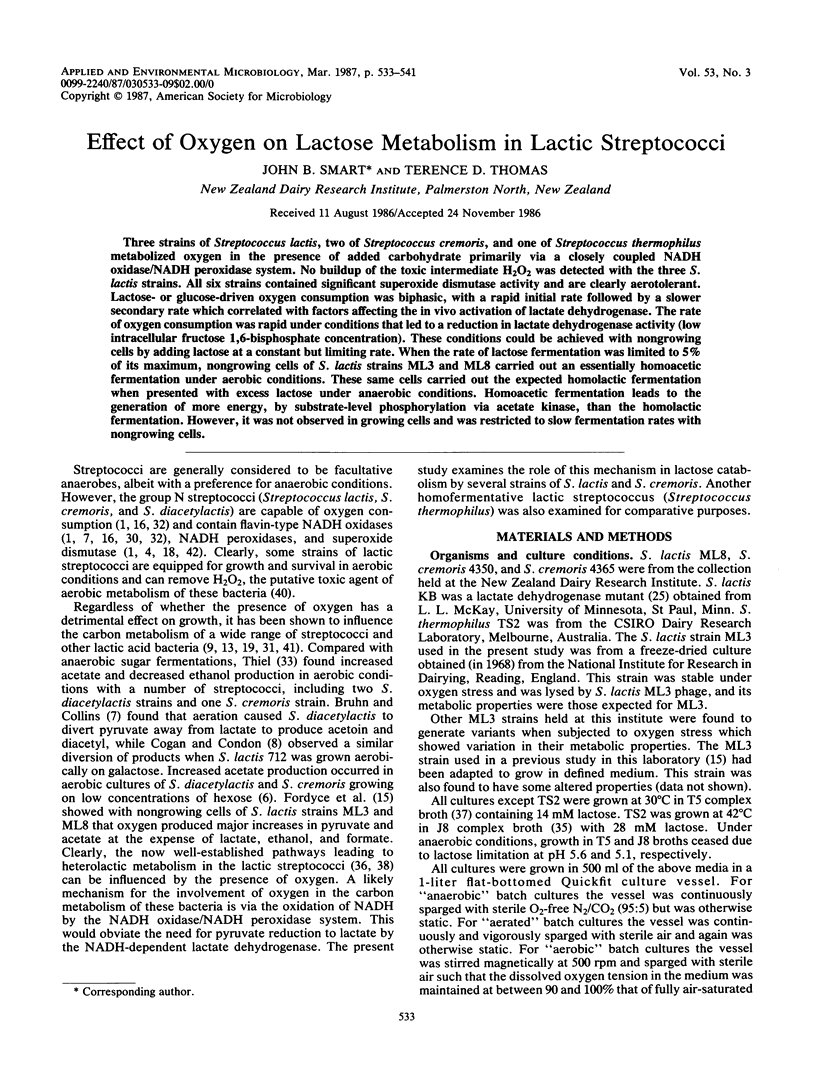
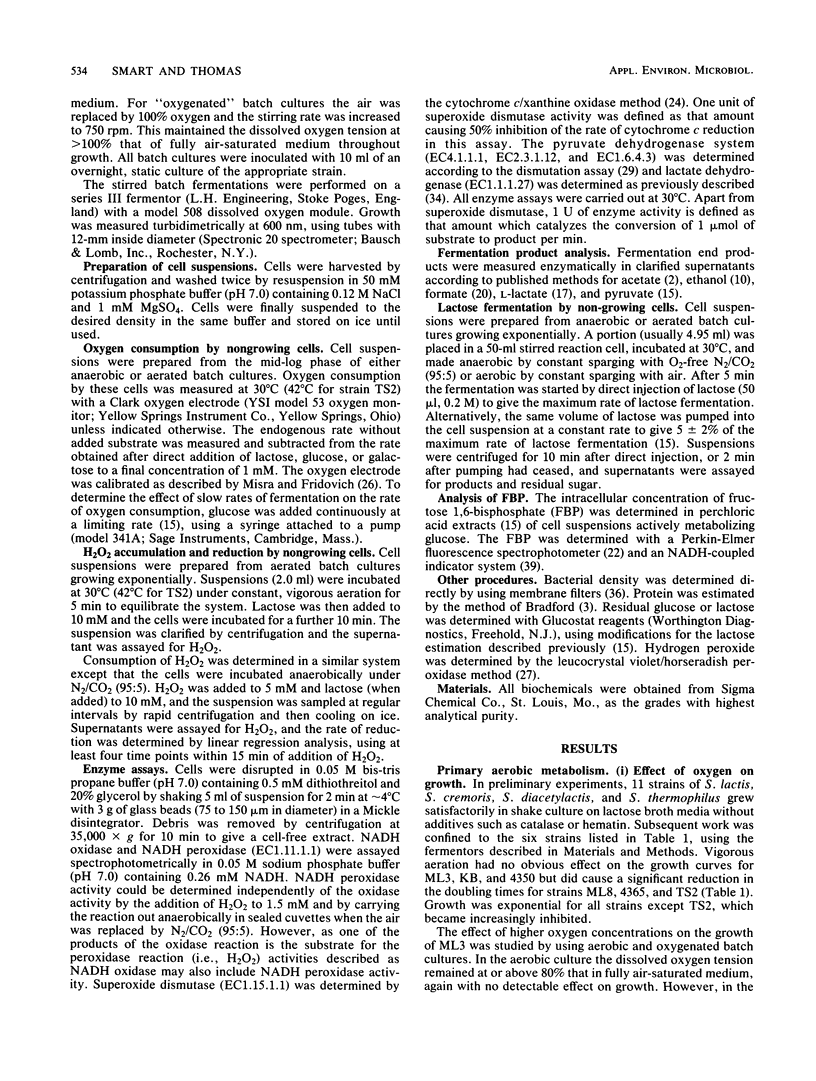
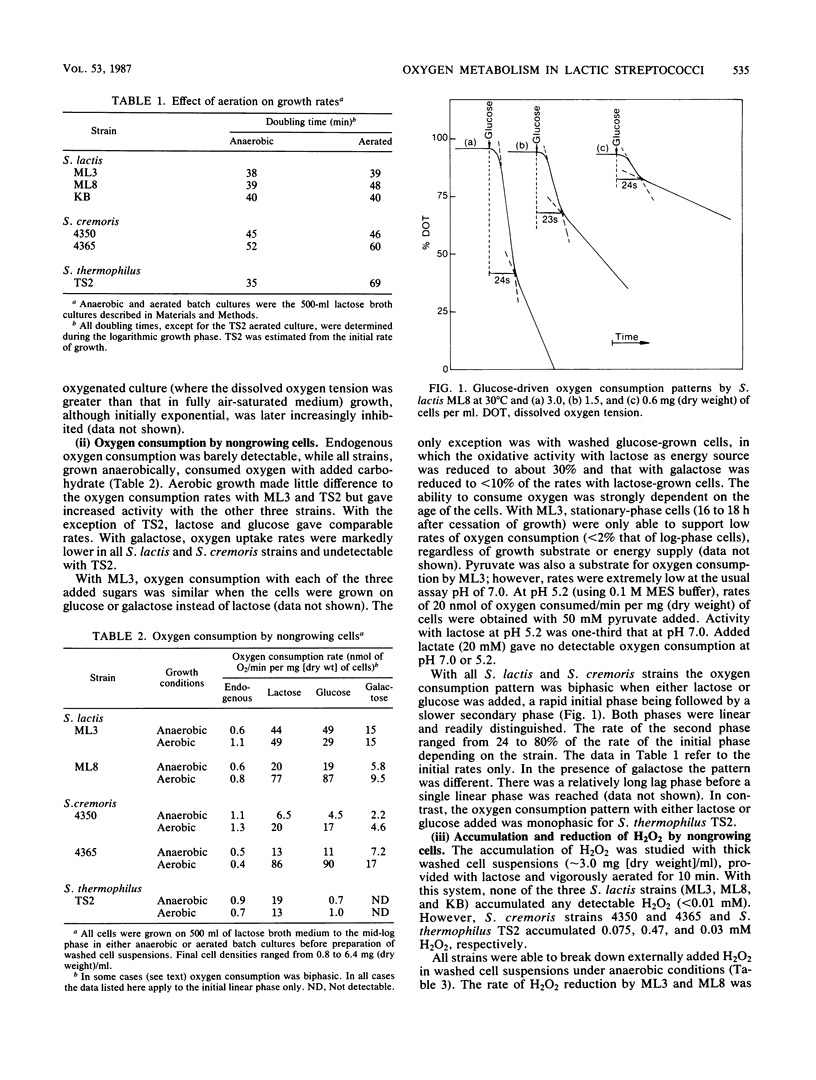
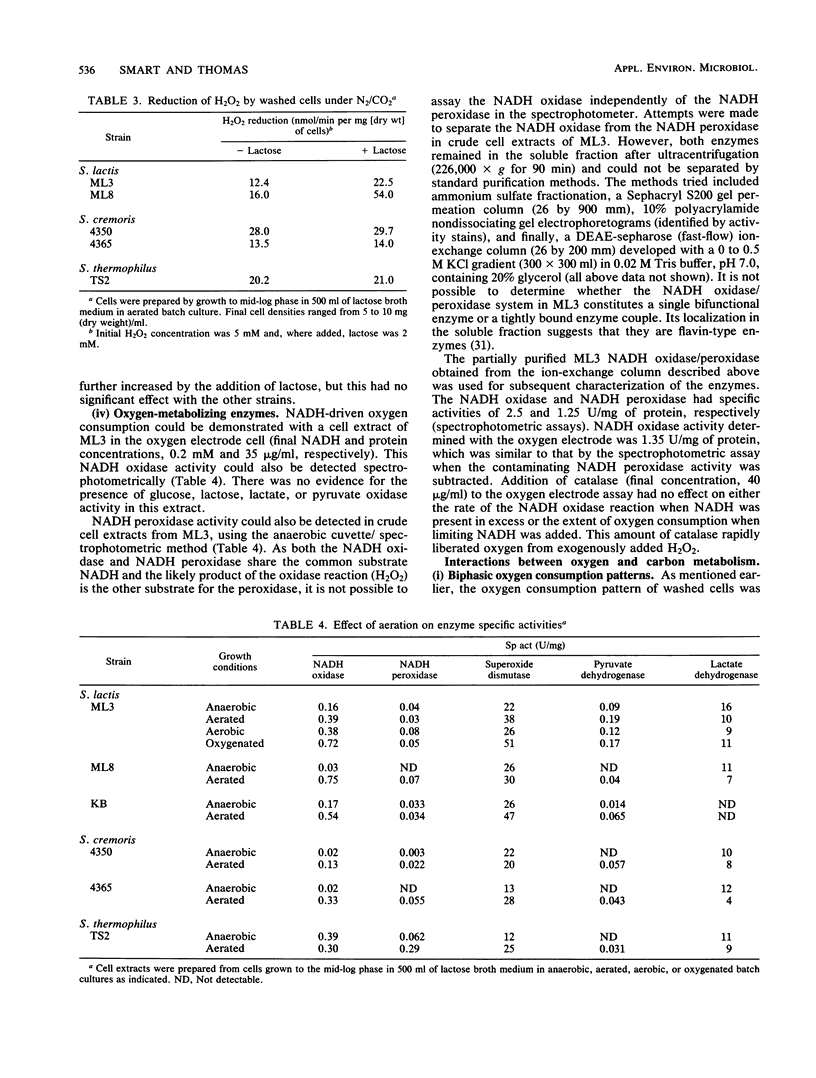
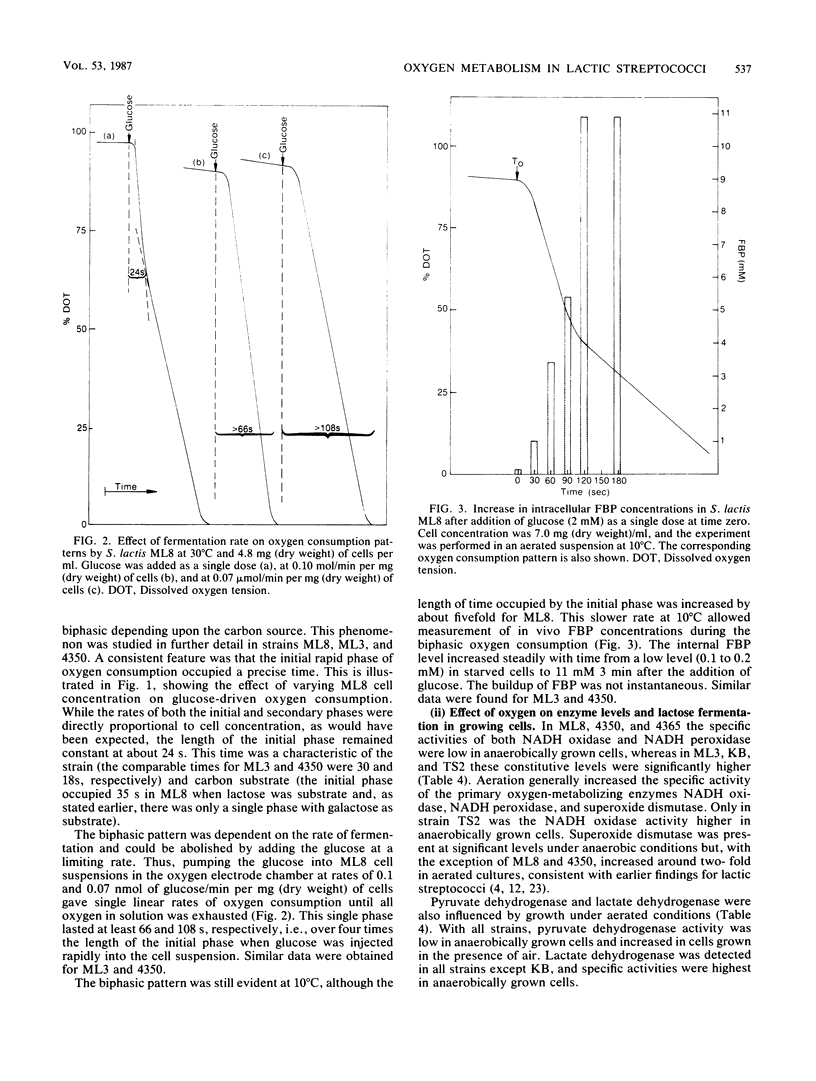
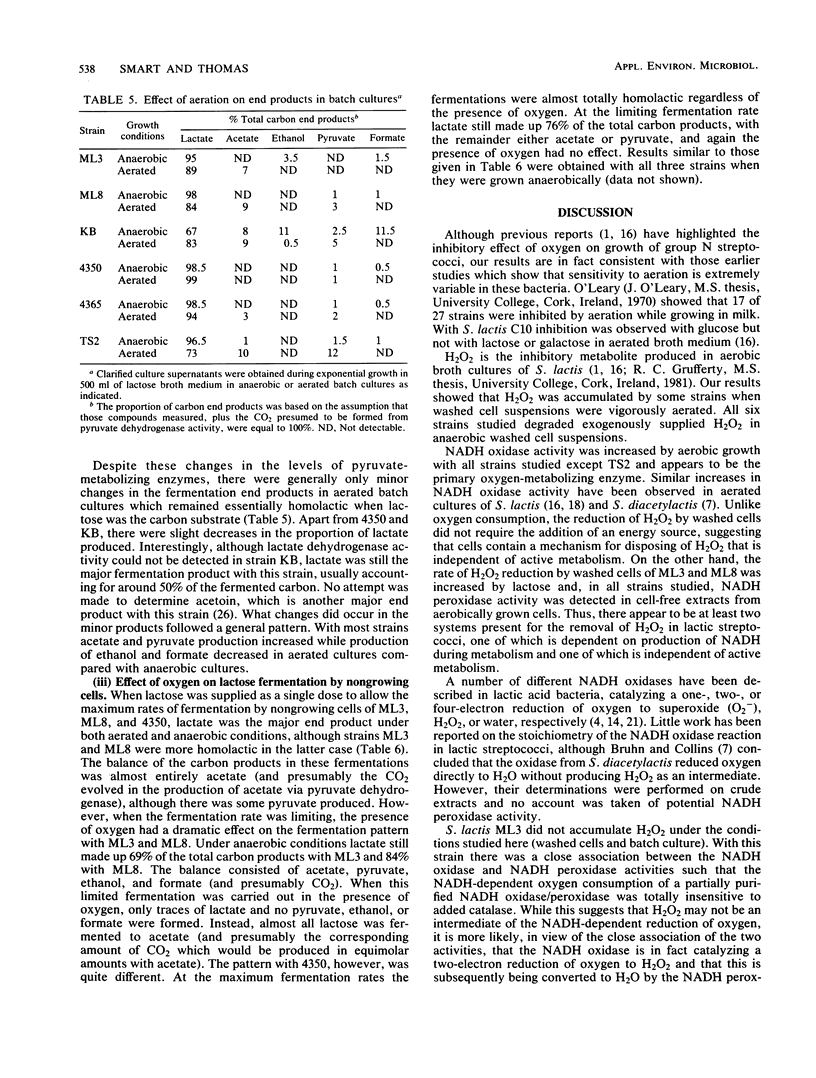
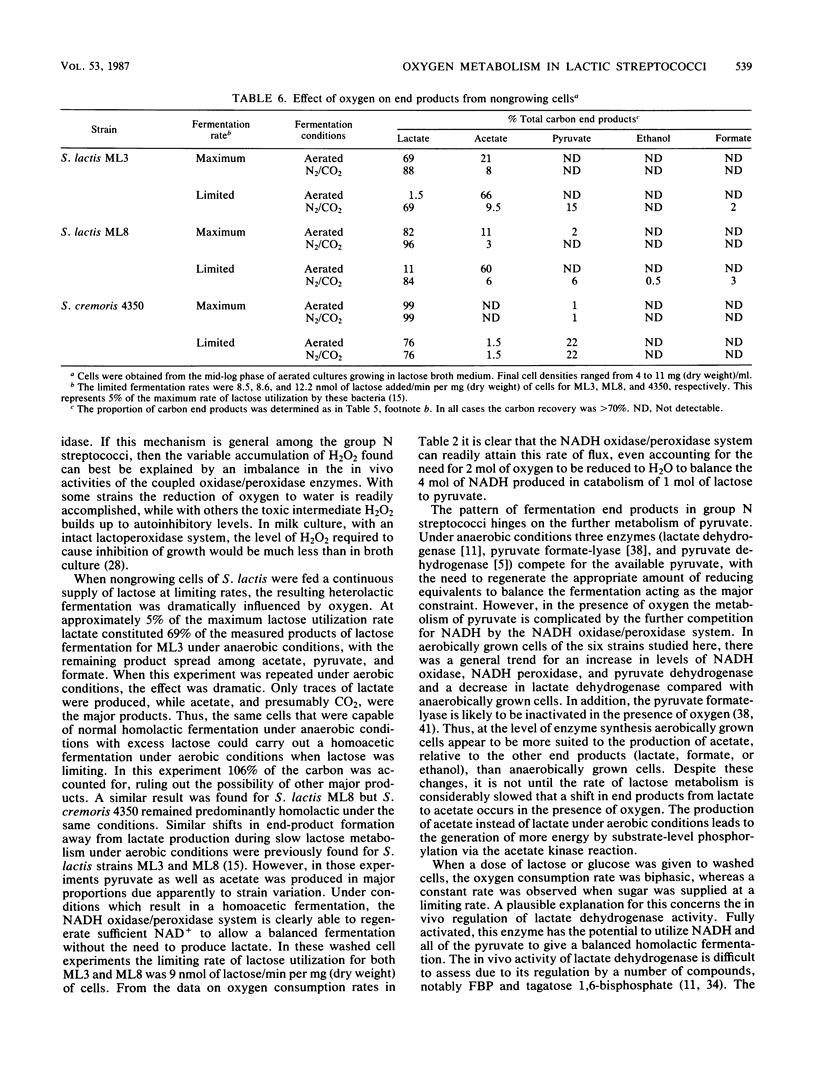
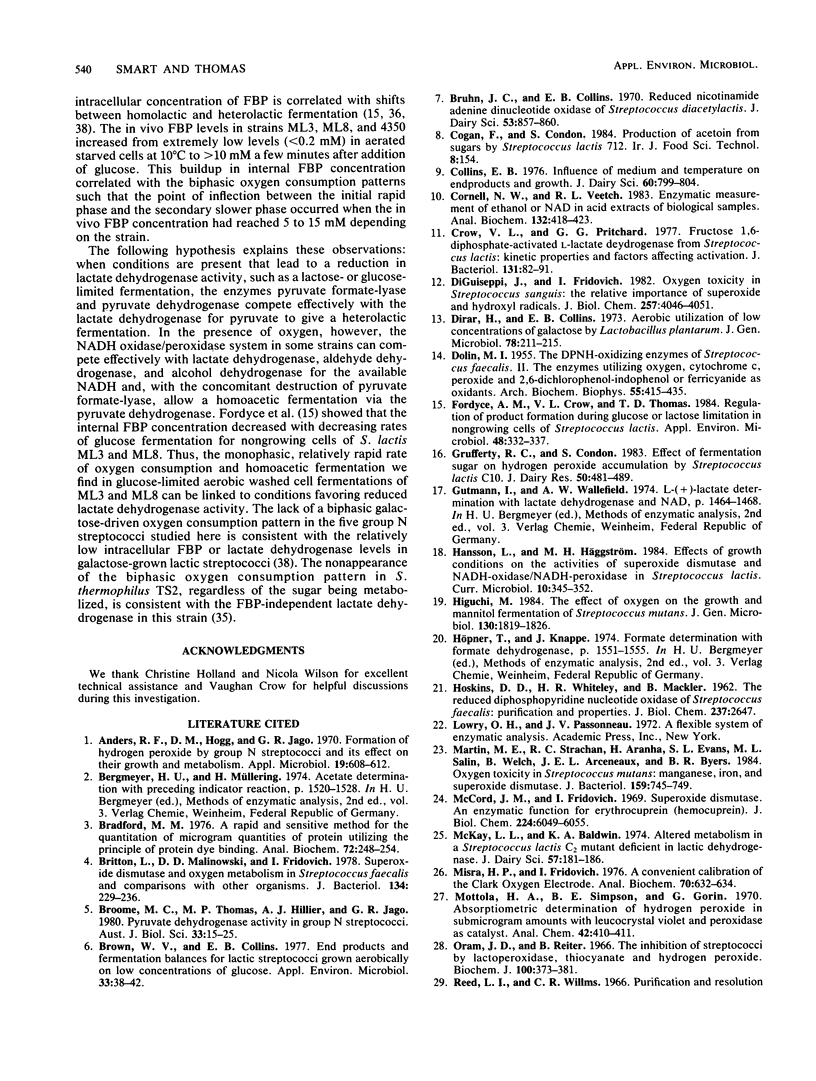
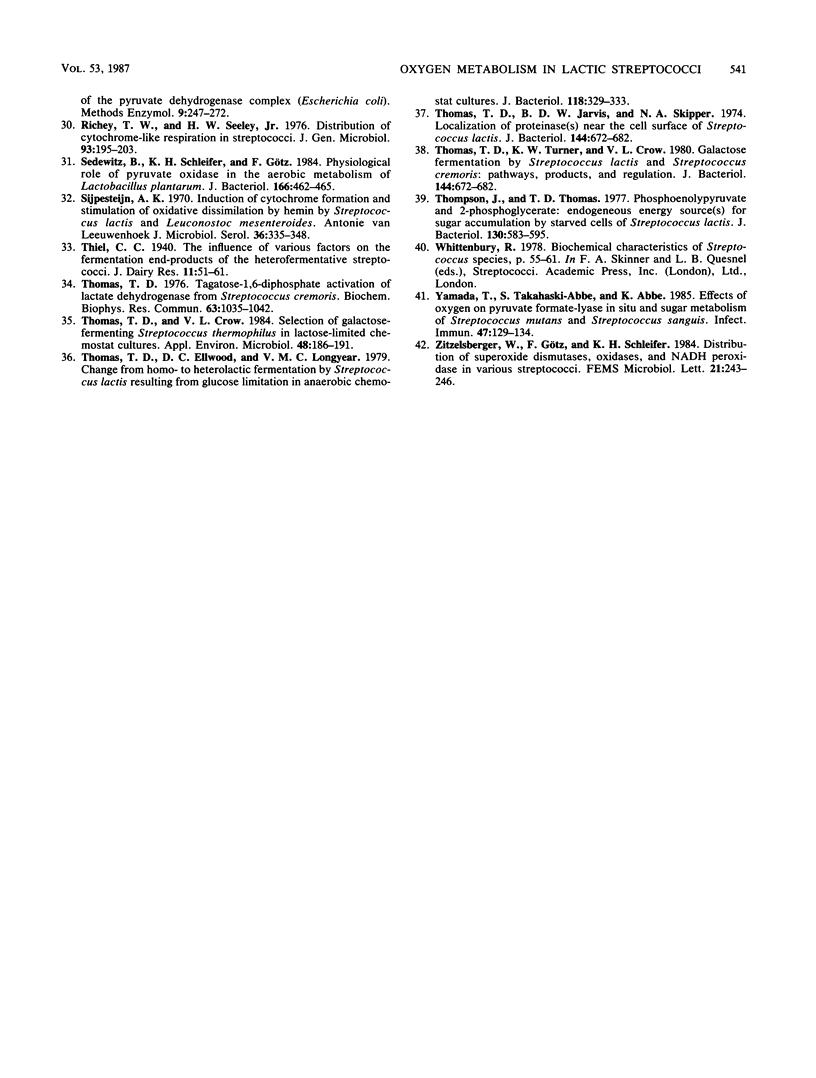
Selected References
These references are in PubMed. This may not be the complete list of references from this article.
- Anders R. F., Hogg D. M., Jago G. R. Formation of hydrogen peroxide by group N streptococci and its effect on their growth and metabolism. Appl Microbiol. 1970 Apr;19(4):608–612. doi: 10.1128/am.19.4.608-612.1970. [DOI] [PMC free article] [PubMed] [Google Scholar]
- Bradford M. M. A rapid and sensitive method for the quantitation of microgram quantities of protein utilizing the principle of protein-dye binding. Anal Biochem. 1976 May 7;72:248–254. doi: 10.1006/abio.1976.9999. [DOI] [PubMed] [Google Scholar]
- Britton L., Malinowski D. P., Fridovich I. Superoxide dismutase and oxygen metabolism in Streptococcus faecalis and comparisons with other organisms. J Bacteriol. 1978 Apr;134(1):229–236. doi: 10.1128/jb.134.1.229-236.1978. [DOI] [PMC free article] [PubMed] [Google Scholar]
- Broome M. C., Thomas M. P., Hillier A. J., Jago G. R. Pyruvate dehydrogenase activity in group N streptococci. Aust J Biol Sci. 1980 Mar;33(1):15–25. [PubMed] [Google Scholar]
- Brown W. V., Collins E. B. End products and fermentation balances for lactic streptococci grown aerobically on low concentrations of glucose. Appl Environ Microbiol. 1977 Jan;33(1):38–42. doi: 10.1128/aem.33.1.38-42.1977. [DOI] [PMC free article] [PubMed] [Google Scholar]
- Bruhn J. C., Collins E. B. Reduced nicotinamide adenine dinucleotide oxidase of Streptococcus diacetilactis. J Dairy Sci. 1970 Jul;53(7):857–860. doi: 10.3168/jds.S0022-0302(70)86307-X. [DOI] [PubMed] [Google Scholar]
- Cornell N. W., Veech R. L. Enzymatic measurement of ethanol or NAD in acid extracts of biological samples. Anal Biochem. 1983 Jul 15;132(2):418–423. doi: 10.1016/0003-2697(83)90029-5. [DOI] [PubMed] [Google Scholar]
- Crow V. L., Pritchard G. G. Fructose 1,6-diphosphate-activated L-lactate dehydrogenase from Streptococcus lactis: kinetic properties and factors affecting activation. J Bacteriol. 1977 Jul;131(1):82–91. doi: 10.1128/jb.131.1.82-91.1977. [DOI] [PMC free article] [PubMed] [Google Scholar]
- DiGuiseppi J., Fridovich I. Oxygen toxicity in Streptococcus sanguis. The relative importance of superoxide and hydroxyl radicals. J Biol Chem. 1982 Apr 25;257(8):4046–4051. [PubMed] [Google Scholar]
- Dirar H., Collins E. B. Aerobic utilization of low concentrations of galactose by Lactobacillus plantarum. J Gen Microbiol. 1973 Oct;78(2):211–215. doi: 10.1099/00221287-78-2-211. [DOI] [PubMed] [Google Scholar]
- Fordyce A. M., Crow V. L., Thomas T. D. Regulation of product formation during glucose or lactose limitation in nongrowing cells of Streptococcus lactis. Appl Environ Microbiol. 1984 Aug;48(2):332–337. doi: 10.1128/aem.48.2.332-337.1984. [DOI] [PMC free article] [PubMed] [Google Scholar]
- HOSKINS D. D., WHITELEY H. R., MACKLER B. The reduced diphosphopyridine nucleotide oxidase of Streptococcus faecalis: purification and properties. J Biol Chem. 1962 Aug;237:2647–2651. [PubMed] [Google Scholar]
- Higuchi M. The effect of oxygen on the growth and mannitol fermentation of Streptococcus mutants. J Gen Microbiol. 1984 Jul;130(7):1819–1826. doi: 10.1099/00221287-130-7-1819. [DOI] [PubMed] [Google Scholar]
- Martin M. E., Strachan R. C., Aranha H., Evans S. L., Salin M. L., Welch B., Arceneaux J. E., Byers B. R. Oxygen toxicity in Streptococcus mutans: manganese, iron, and superoxide dismutase. J Bacteriol. 1984 Aug;159(2):745–749. doi: 10.1128/jb.159.2.745-749.1984. [DOI] [PMC free article] [PubMed] [Google Scholar]
- McCord J. M., Fridovich I. Superoxide dismutase. An enzymic function for erythrocuprein (hemocuprein). J Biol Chem. 1969 Nov 25;244(22):6049–6055. [PubMed] [Google Scholar]
- McKay L. L., Baldwin K. A. Altered metabolism in a Streptococcus lactis C2 mutant deficient in lactic dehydrogenase. J Dairy Sci. 1974 Feb;57(2):181–186. doi: 10.3168/jds.S0022-0302(74)84857-5. [DOI] [PubMed] [Google Scholar]
- Misra H. P., Fridovich I. A convenient calibration of the Clark oxygen electrode. Anal Biochem. 1976 Feb;70(2):632–634. doi: 10.1016/0003-2697(76)90492-9. [DOI] [PubMed] [Google Scholar]
- Oram J. D., Reiter B. The inhibition of streptococci by lactoperoxidase, thiocyanate and hydrogen peroxide. The effect of the inhibitory system on susceptible and resistant strains of group N streptococci. Biochem J. 1966 Aug;100(2):373–381. doi: 10.1042/bj1000373. [DOI] [PMC free article] [PubMed] [Google Scholar]
- Ritchey T. W., Seely H. W., Jr Distribution of cytochrome-like respiration in streptococci. J Gen Microbiol. 1976 Apr;93(2):195–203. doi: 10.1099/00221287-93-2-195. [DOI] [PubMed] [Google Scholar]
- Sedewitz B., Schleifer K. H., Götz F. Physiological role of pyruvate oxidase in the aerobic metabolism of Lactobacillus plantarum. J Bacteriol. 1984 Oct;160(1):462–465. doi: 10.1128/jb.160.1.462-465.1984. [DOI] [PMC free article] [PubMed] [Google Scholar]
- Sijpesteijn A. K. Induction of cytochrome formation and stimulation of oxidative dissimilation by hemin in Streptococcus lactis and Leuconostoc mesenteroides. Antonie Van Leeuwenhoek. 1970;36(3):335–348. doi: 10.1007/BF02069035. [DOI] [PubMed] [Google Scholar]
- Thomas T. D., Crow V. L. Selection of Galactose-Fermenting Streptococcus thermophilus in Lactose-Limited Chemostat Cultures. Appl Environ Microbiol. 1984 Jul;48(1):186–191. doi: 10.1128/aem.48.1.186-191.1984. [DOI] [PMC free article] [PubMed] [Google Scholar]
- Thomas T. D., Jarvis B. D., Skipper N. A. Localization of proteinase(s) near the cell surface of Streptococcus lactis. J Bacteriol. 1974 May;118(2):329–333. doi: 10.1128/jb.118.2.329-333.1974. [DOI] [PMC free article] [PubMed] [Google Scholar]
- Thomas T. D. Tagatose-1, 6-diphosphate activation of lactate dehydrogenase from Streptococcus cremoris. Biochem Biophys Res Commun. 1975 Apr 21;63(4):1035–1042. doi: 10.1016/0006-291x(75)90673-7. [DOI] [PubMed] [Google Scholar]
- Thomas T. D., Turner K. W., Crow V. L. Galactose fermentation by Streptococcus lactis and Streptococcus cremoris: pathways, products, and regulation. J Bacteriol. 1980 Nov;144(2):672–682. doi: 10.1128/jb.144.2.672-682.1980. [DOI] [PMC free article] [PubMed] [Google Scholar]
- Thomas T. D., Turner K. W., Crow V. L. Galactose fermentation by Streptococcus lactis and Streptococcus cremoris: pathways, products, and regulation. J Bacteriol. 1980 Nov;144(2):672–682. doi: 10.1128/jb.144.2.672-682.1980. [DOI] [PMC free article] [PubMed] [Google Scholar]
- Thompson J., Thomas T. D. Phosphoenolpyruvate and 2-phosphoglycerate: endogenous energy source(s) for sugar accumulation by starved cells of Streptococcus lactis. J Bacteriol. 1977 May;130(2):583–595. doi: 10.1128/jb.130.2.583-595.1977. [DOI] [PMC free article] [PubMed] [Google Scholar]
- Yamada T., Takahashi-Abbe S., Abbe K. Effects of oxygen on pyruvate formate-lyase in situ and sugar metabolism of Streptococcus mutans and Streptococcus sanguis. Infect Immun. 1985 Jan;47(1):129–134. doi: 10.1128/iai.47.1.129-134.1985. [DOI] [PMC free article] [PubMed] [Google Scholar]