Abstract
Toluene-treated cells of Streptococcus bovis JB1 phosphorylated cellobiose, glucose, maltose, and sucrose by the phosphoenolpyruvate-dependent phosphotransferase system. Glucose phosphorylation was constitutive, while all three disaccharide systems were inducible. Competition experiments indicated that separate phosphotransferase systems (enzymes II) existed for glucose, maltose, and sucrose. [14C]maltose transport was inhibited by excess (10 mM) glucose and to a lesser extent by sucrose (90 and 46%, respectively). [14C]glucose and [14C]sucrose transports were not inhibited by an excess of maltose. Since [14C]maltose phosphorylation in triethanolamine buffer was increased 160-fold as the concentration of Pi was increased from 0 to 100 mM, a maltose phosphorylase (Km for Pi, 9.5 mM) was present, and this activity was inducible. Maltose was also hydrolyzed by an inducible maltase. Glucose 1-phosphate arising from the maltose phosphorylase was metabolized by a constitutive phosphoglucomutase that was specific for alpha-glucose 1-phosphate (Km, 0.8 mM). Only sucrose-grown cells possessed sucrose hydrolase activity (Km, 3.1 mM), and this activity was much lower than the sucrose phosphotransferase system and sucrose-phosphate hydrolase activities.
Full text
PDF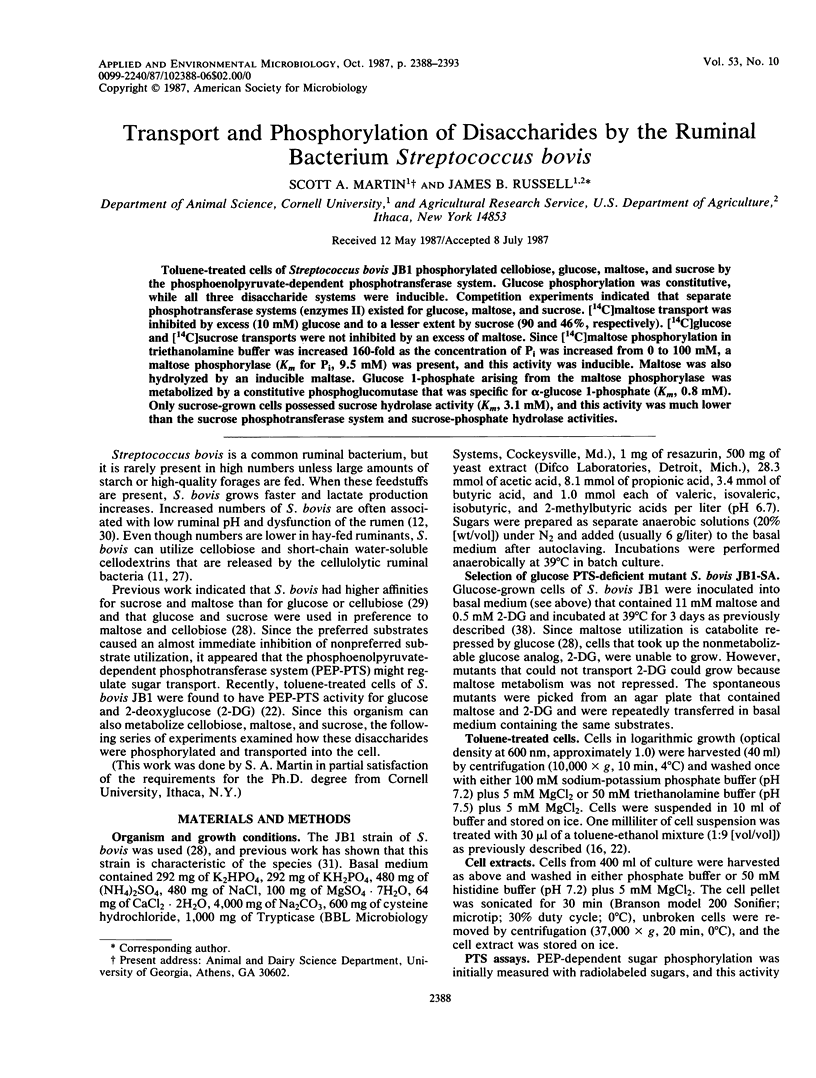
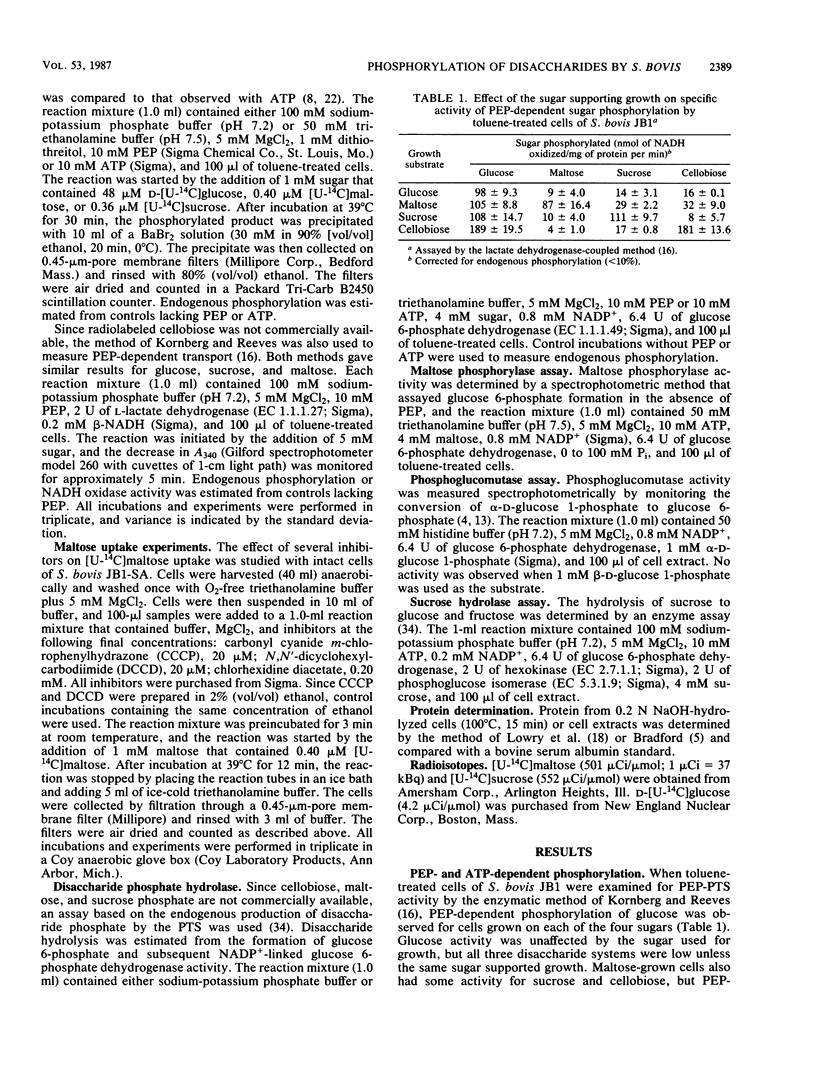
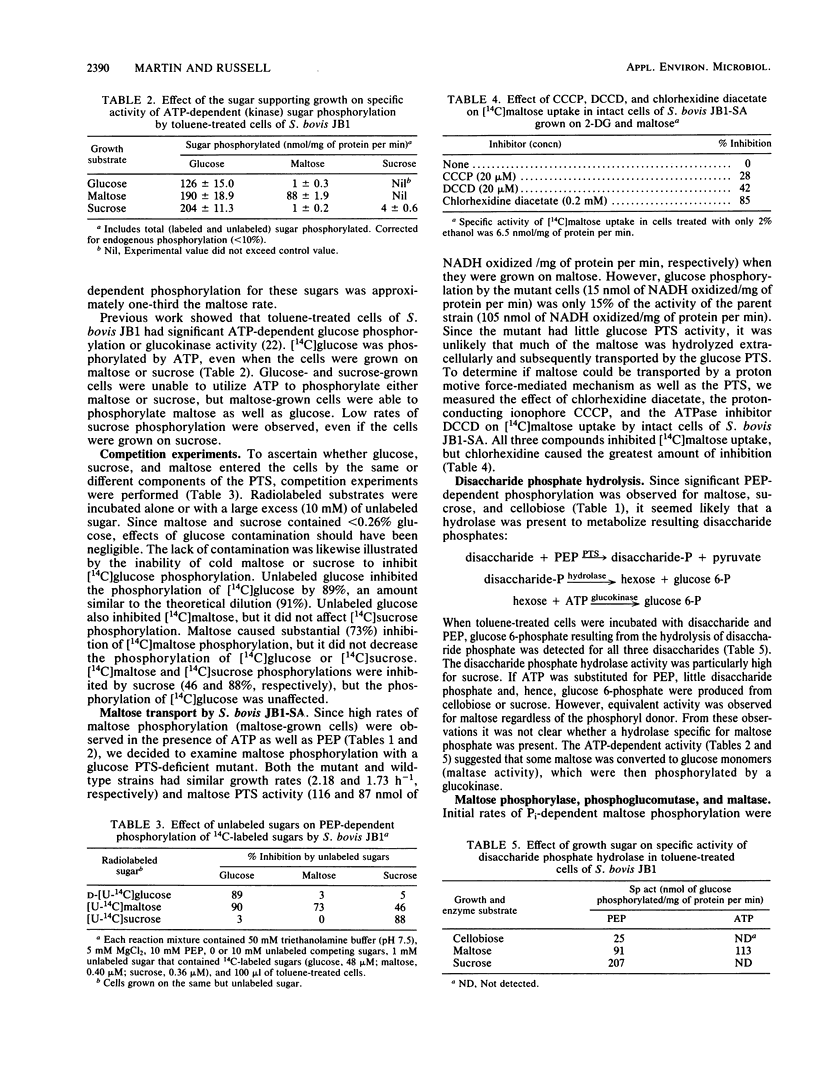
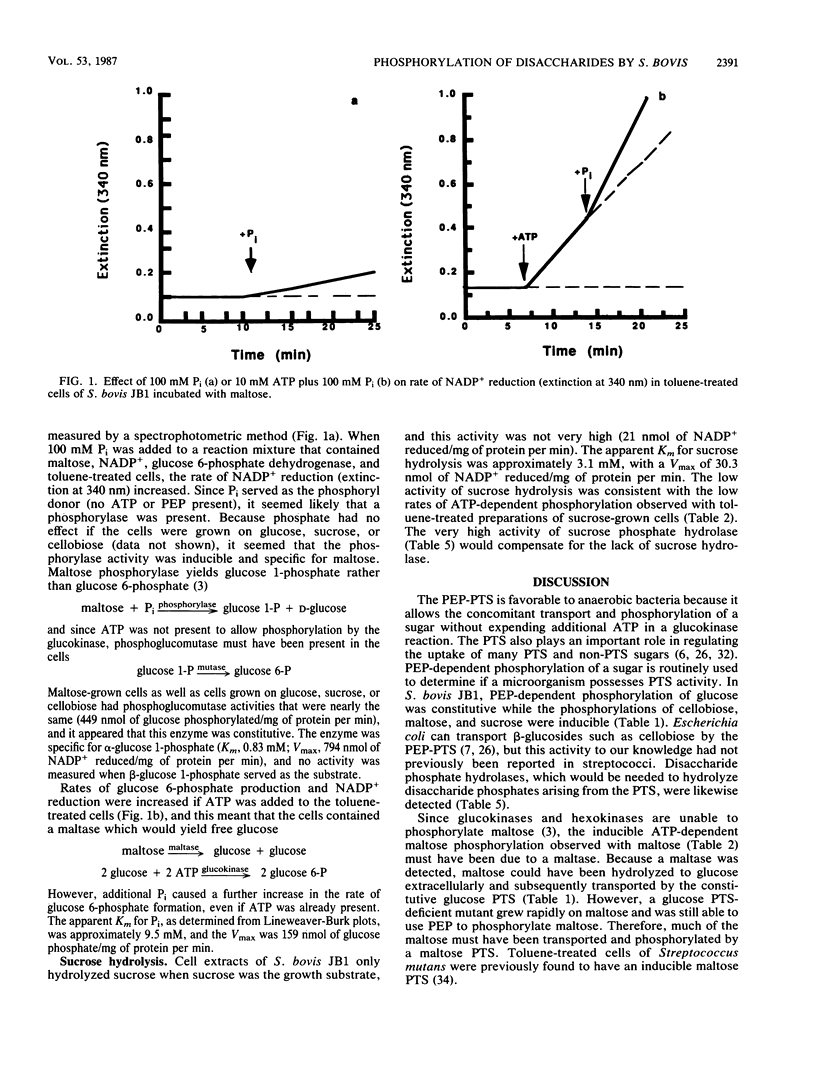
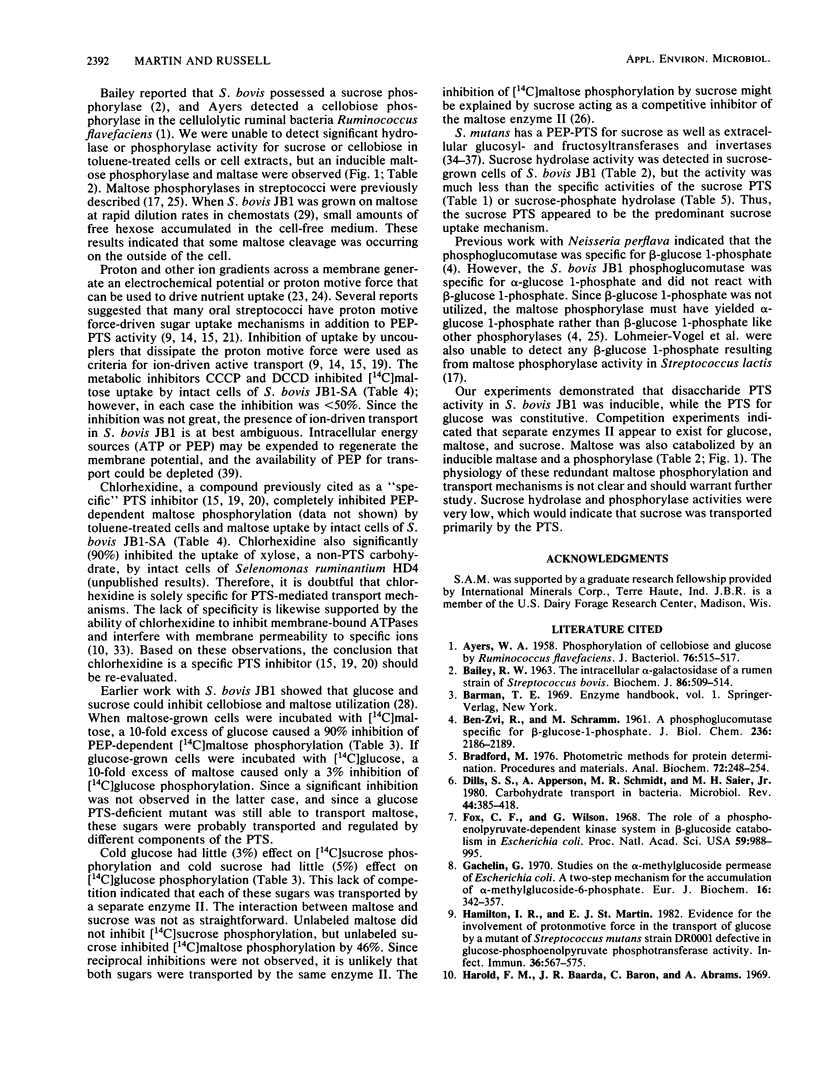
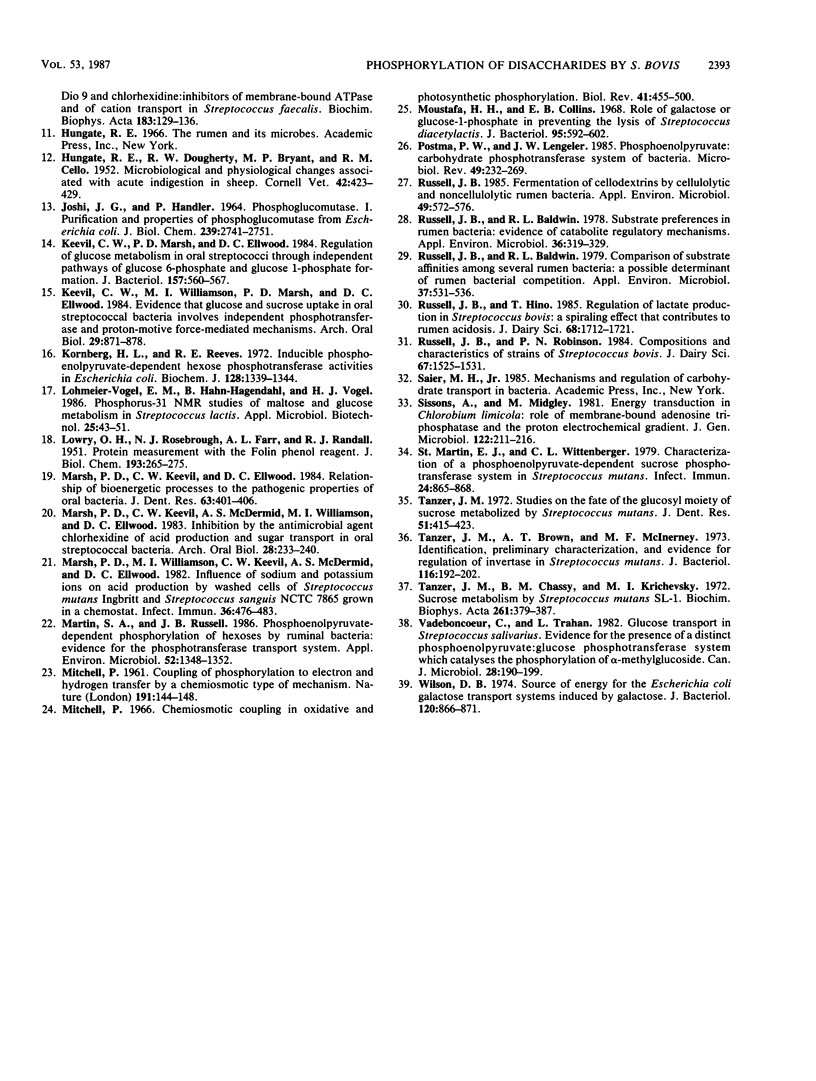
Selected References
These references are in PubMed. This may not be the complete list of references from this article.
- AYERS W. A. Phosphorylation of cellobiose and glucose by Ruminococcus flavefaciens. J Bacteriol. 1958 Nov;76(5):515–517. doi: 10.1128/jb.76.5.515-517.1958. [DOI] [PMC free article] [PubMed] [Google Scholar]
- BAILEY R. W. The intracellular alpha-galactodidase of a rumen strain of Streptococcus bovis. Biochem J. 1963 Mar;86:509–514. doi: 10.1042/bj0860509. [DOI] [PMC free article] [PubMed] [Google Scholar]
- Bradford M. M. A rapid and sensitive method for the quantitation of microgram quantities of protein utilizing the principle of protein-dye binding. Anal Biochem. 1976 May 7;72:248–254. doi: 10.1006/abio.1976.9999. [DOI] [PubMed] [Google Scholar]
- Dills S. S., Apperson A., Schmidt M. R., Saier M. H., Jr Carbohydrate transport in bacteria. Microbiol Rev. 1980 Sep;44(3):385–418. doi: 10.1128/mr.44.3.385-418.1980. [DOI] [PMC free article] [PubMed] [Google Scholar]
- Fox C. F., Wilson G. The role of a phosphoenolpyruvate-dependent kinase system in beta-glucoside catabolism in Escherichia coli. Proc Natl Acad Sci U S A. 1968 Mar;59(3):988–995. doi: 10.1073/pnas.59.3.988. [DOI] [PMC free article] [PubMed] [Google Scholar]
- Gachelin G. Studies on the alpha-methylglucoside permease of Escherichia coli. A two-step mechanism for the accumulation of alpha-methylglucoside 6-phosphate. Eur J Biochem. 1970 Oct;16(2):342–357. doi: 10.1111/j.1432-1033.1970.tb01088.x. [DOI] [PubMed] [Google Scholar]
- HUNGATE R. E., DOUGHERTY R. W., BRYANT M. P., CELLO R. M. Microbiological and physiological changes associated with acute indigestion in sheep. Cornell Vet. 1952 Oct;42(4):423–449. [PubMed] [Google Scholar]
- Hamilton I. R., St Martin E. J. Evidence for the involvement of proton motive force in the transport of glucose by a mutant of Streptococcus mutans strain DR0001 defective in glucose-phosphoenolpyruvate phosphotransferase activity. Infect Immun. 1982 May;36(2):567–575. doi: 10.1128/iai.36.2.567-575.1982. [DOI] [PMC free article] [PubMed] [Google Scholar]
- Harold F. M., Baarda J. R., Baron C., Abrams A. Dio 9 and chlorhexidine: inhibitors of membrane-bound ATPase and of cation transport in Streptococcus faecalis. Biochim Biophys Acta. 1969 Jun 3;183(1):129–136. doi: 10.1016/0005-2736(69)90136-9. [DOI] [PubMed] [Google Scholar]
- JOSHI J. G., HANDLER P. PHOSPHOGLUCOMUTASE. I. PURIFICATION AND PROPERTIES OF PHOSPHOGLUCOMUTASE FROM ESCHERICHIA COLI. J Biol Chem. 1964 Sep;239:2741–2751. [PubMed] [Google Scholar]
- Keevil C. W., Marsh P. D., Ellwood D. C. Regulation of glucose metabolism in oral streptococci through independent pathways of glucose 6-phosphate and glucose 1-phosphate formation. J Bacteriol. 1984 Feb;157(2):560–567. doi: 10.1128/jb.157.2.560-567.1984. [DOI] [PMC free article] [PubMed] [Google Scholar]
- Keevil C. W., Williamson M. I., Marsh P. D., Ellwood D. C. Evidence that glucose and sucrose uptake in oral streptococcal bacteria involves independent phosphotransferase and proton-motive force-mediated mechanisms. Arch Oral Biol. 1984;29(11):871–878. doi: 10.1016/0003-9969(84)90085-2. [DOI] [PubMed] [Google Scholar]
- Kornberg H. L., Reeves R. E. Inducible phosphoenolpyruvate-dependent hexose phosphotransferase activities in Escherichia coli. Biochem J. 1972 Aug;128(5):1339–1344. doi: 10.1042/bj1281339. [DOI] [PMC free article] [PubMed] [Google Scholar]
- LOWRY O. H., ROSEBROUGH N. J., FARR A. L., RANDALL R. J. Protein measurement with the Folin phenol reagent. J Biol Chem. 1951 Nov;193(1):265–275. [PubMed] [Google Scholar]
- MITCHELL P. Coupling of phosphorylation to electron and hydrogen transfer by a chemi-osmotic type of mechanism. Nature. 1961 Jul 8;191:144–148. doi: 10.1038/191144a0. [DOI] [PubMed] [Google Scholar]
- Marsh P. D., Keevil C. W., Ellwood D. C. Relationship of bioenergetic processes to the pathogenic properties of oral bacteria. J Dent Res. 1984 Mar;63(3):401–406. doi: 10.1177/00220345840630030901. [DOI] [PubMed] [Google Scholar]
- Marsh P. D., Keevil C. W., McDermid A. S., Williamson M. I., Ellwood D. C. Inhibition by the antimicrobial agent chlorhexidine of acid production and sugar transport in oral streptococcal bacteria. Arch Oral Biol. 1983;28(3):233–240. doi: 10.1016/0003-9969(83)90152-8. [DOI] [PubMed] [Google Scholar]
- Marsh P. D., Williamson M. I., Keevil C. W., McDermid A. S., Ellwood D. C. Influence of sodium and potassium ions on acid production by washed cells of Streptococcus mutans ingbritt and Streptococcus sanguis NCTC 7865 grown in a chemostat. Infect Immun. 1982 May;36(2):476–483. doi: 10.1128/iai.36.2.476-483.1982. [DOI] [PMC free article] [PubMed] [Google Scholar]
- Martin S. A., Russell J. B. Phosphoenolpyruvate-dependent phosphorylation of hexoses by ruminal bacteria: evidence for the phosphotransferase transport system. Appl Environ Microbiol. 1986 Dec;52(6):1348–1352. doi: 10.1128/aem.52.6.1348-1352.1986. [DOI] [PMC free article] [PubMed] [Google Scholar]
- Moustafa H. H., Collins E. B. Role of galactose or glucose-1-phosphate in preventing the lysis of Streptococcus diacetilactis. J Bacteriol. 1968 Feb;95(2):592–602. doi: 10.1128/jb.95.2.592-602.1968. [DOI] [PMC free article] [PubMed] [Google Scholar]
- Postma P. W., Lengeler J. W. Phosphoenolpyruvate:carbohydrate phosphotransferase system of bacteria. Microbiol Rev. 1985 Sep;49(3):232–269. doi: 10.1128/mr.49.3.232-269.1985. [DOI] [PMC free article] [PubMed] [Google Scholar]
- Russell J. B., Baldwin R. L. Comparison of substrate affinities among several rumen bacteria: a possible determinant of rumen bacterial competition. Appl Environ Microbiol. 1979 Mar;37(3):531–536. doi: 10.1128/aem.37.3.531-536.1979. [DOI] [PMC free article] [PubMed] [Google Scholar]
- Russell J. B., Baldwin R. L. Substrate preferences in rumen bacteria: evidence of catabolite regulatory mechanisms. Appl Environ Microbiol. 1978 Aug;36(2):319–329. doi: 10.1128/aem.36.2.319-329.1978. [DOI] [PMC free article] [PubMed] [Google Scholar]
- Russell J. B. Fermentation of cellodextrins by cellulolytic and noncellulolytic rumen bacteria. Appl Environ Microbiol. 1985 Mar;49(3):572–576. doi: 10.1128/aem.49.3.572-576.1985. [DOI] [PMC free article] [PubMed] [Google Scholar]
- Russell J. B., Robinson P. H. Compositions and characteristics of strains of Streptococcus bovis. J Dairy Sci. 1984 Jul;67(7):1525–1531. doi: 10.3168/jds.S0022-0302(84)81471-X. [DOI] [PubMed] [Google Scholar]
- St Martin E. J., Wittenberger C. L. Characterization of a phosphoenolpyruvate-dependent sucrose phosphotransferase system in Streptococcus mutans. Infect Immun. 1979 Jun;24(3):865–868. doi: 10.1128/iai.24.3.865-868.1979. [DOI] [PMC free article] [PubMed] [Google Scholar]
- Tanzer J. M., Brown A. T., McInerney M. F. Identification, preliminary characterization, and evidence for regulation of invertase in Streptococcus mutans. J Bacteriol. 1973 Oct;116(1):192–202. doi: 10.1128/jb.116.1.192-202.1973. [DOI] [PMC free article] [PubMed] [Google Scholar]
- Tanzer J. M., Chassy B. M., Krichevsky M. I. Sucrose metabolism by Streptococcus mutans, SL-I. Biochim Biophys Acta. 1971 Feb 28;261(2):379–387. doi: 10.1016/0304-4165(72)90062-1. [DOI] [PubMed] [Google Scholar]
- Tanzer J. M. Studies on the fate of the glucosyl moiety of sucrose metabolized by Streptococcus mutans. J Dent Res. 1972 Mar-Apr;51(2):415–423. doi: 10.1177/00220345720510023001. [DOI] [PubMed] [Google Scholar]
- Vadeboncoeur C., Trahan L. Glucose transport in Streptococcus salivarius. Evidence for the presence of a distinct phosphoenolpyruvate: glucose phosphotransferase system which catalyses the phosphorylation of alpha-methyl glucoside. Can J Microbiol. 1982 Feb;28(2):190–199. doi: 10.1139/m82-025. [DOI] [PubMed] [Google Scholar]
- Wilson D. B. Source of energy for the Escherichia coli galactose transport systems induced by galactose. J Bacteriol. 1974 Nov;120(2):866–871. doi: 10.1128/jb.120.2.866-871.1974. [DOI] [PMC free article] [PubMed] [Google Scholar]