Abstract
Rhodobacter sphaeroides exhibits two behavioral responses when exposed to some compounds: (i) a chemotactic response that results in accumulation and (ii) a sustained increase in swimming speed. This latter chemokinetic response occurs without any apparent long-term change in the size of the electrochemical proton gradient. The results presented here show that the chemokinetic response is separate from the chemotactic response, although some compounds can induce both responses. Compounds that caused only chemokinesis induced a sustained increase in the rate of flagellar rotation, but chemoeffectors which were also chemotactic caused an additional short-term change in both the stopping frequency and the duration of stops and runs. The response to a change in chemoattractant concentration was a transient increase in the stopping frequency when the concentration was reduced, with adaptation taking between 10 and 60 s. There was also a decrease in the stopping frequency when the concentration was increased, but adaptation took up to 60 min. The nature and duration of both the chemotactic and chemokinetic responses were concentration dependent. Weak organic acids elicited the strongest chemokinetic responses, and although many also caused chemotaxis, there were conditions under which chemokinesis occurred in the absence of chemotaxis. The transportable succinate analog malonate caused chemokinesis but not chemotaxis, as did acetate when added to a mutant able to transport but not grow on acetate. Chemokinesis also occurred after incubation with arsenate, conditions under which chemotaxis was lost, indicating that phosphorylation at some level may have a role in chemotaxis. Aspartate was the only chemoattractant amino acid to cause chemokinesis. Glutamate caused chemotaxis but not chemokinesis. These data suggest that (i) chemotaxis and chemokinesis are separate responses, (ii) metabolism is required for chemotaxis but not chemokinesis, (iii) a reduction in chemoattractant concentration may cause the major chemotactic signal, and (iv) a specific transport pathway(s) may be involved in chemokinetic signalling in R. sphaeroides.
Full text
PDF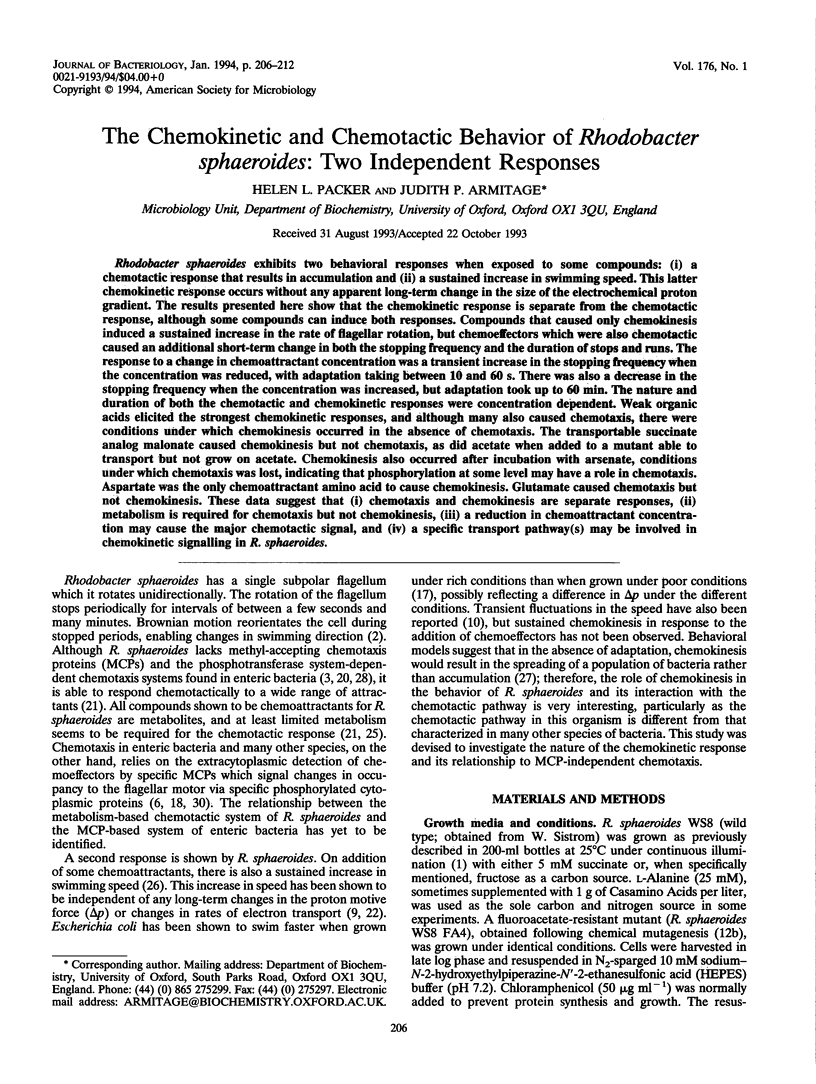
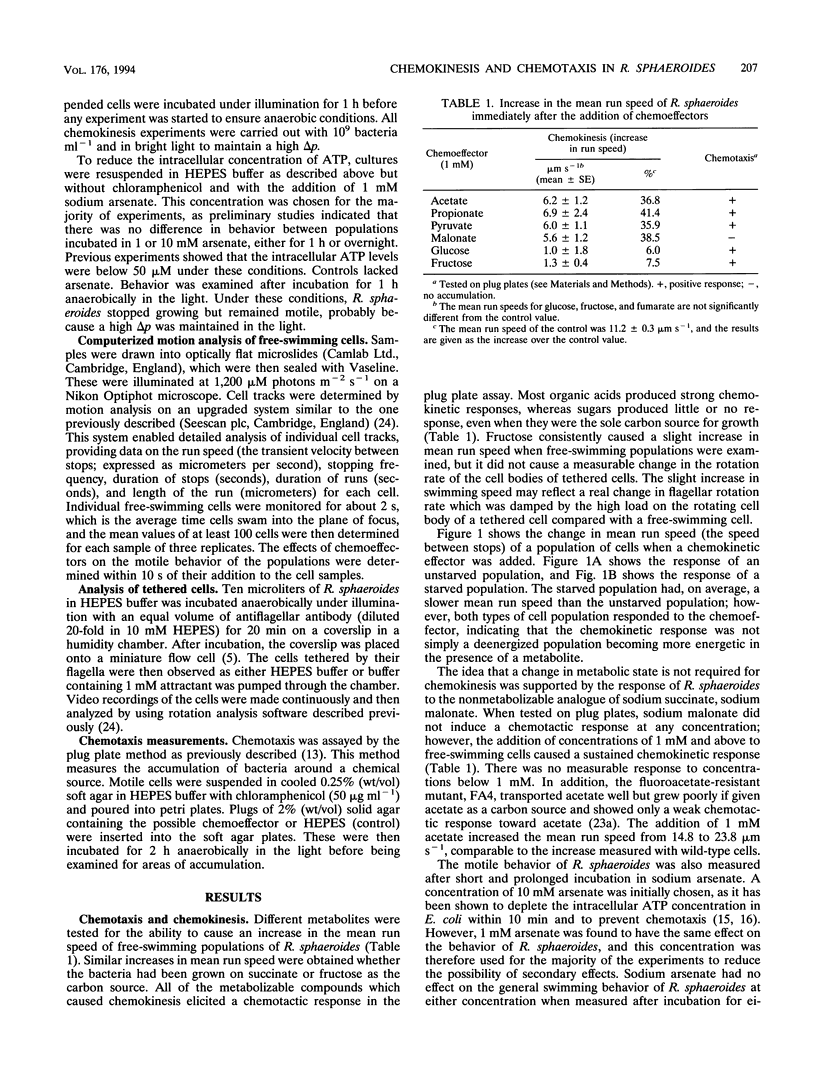
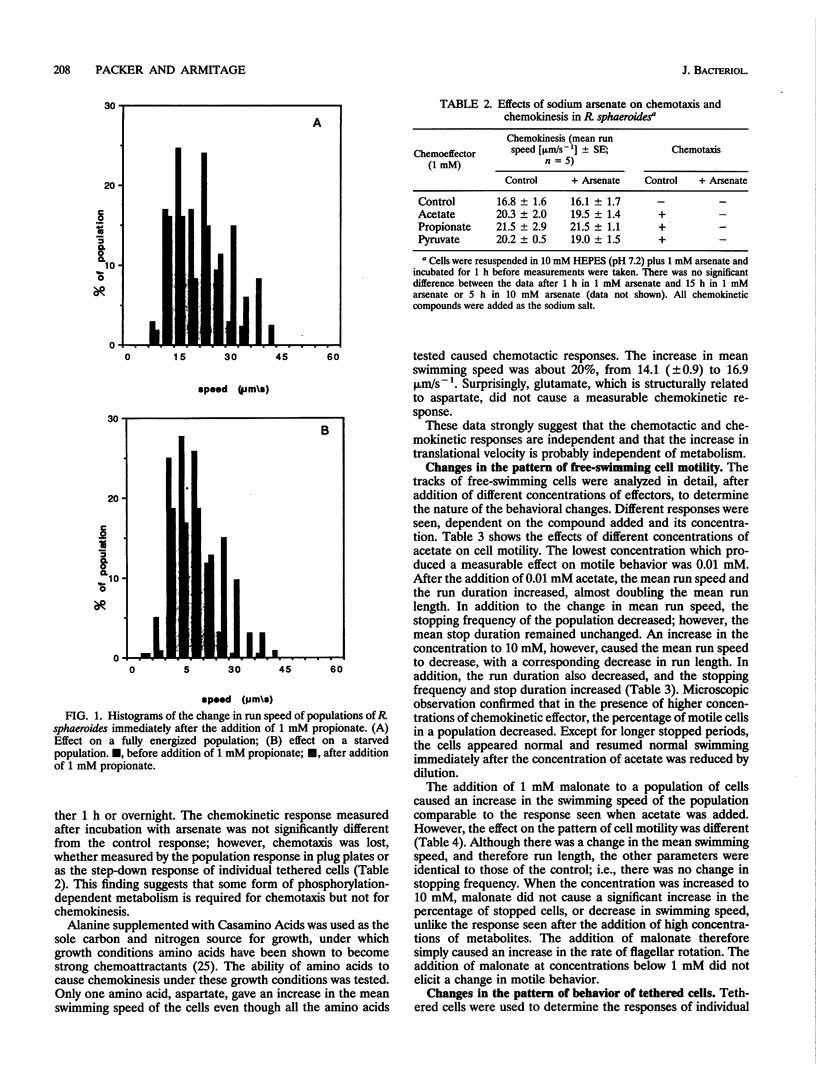
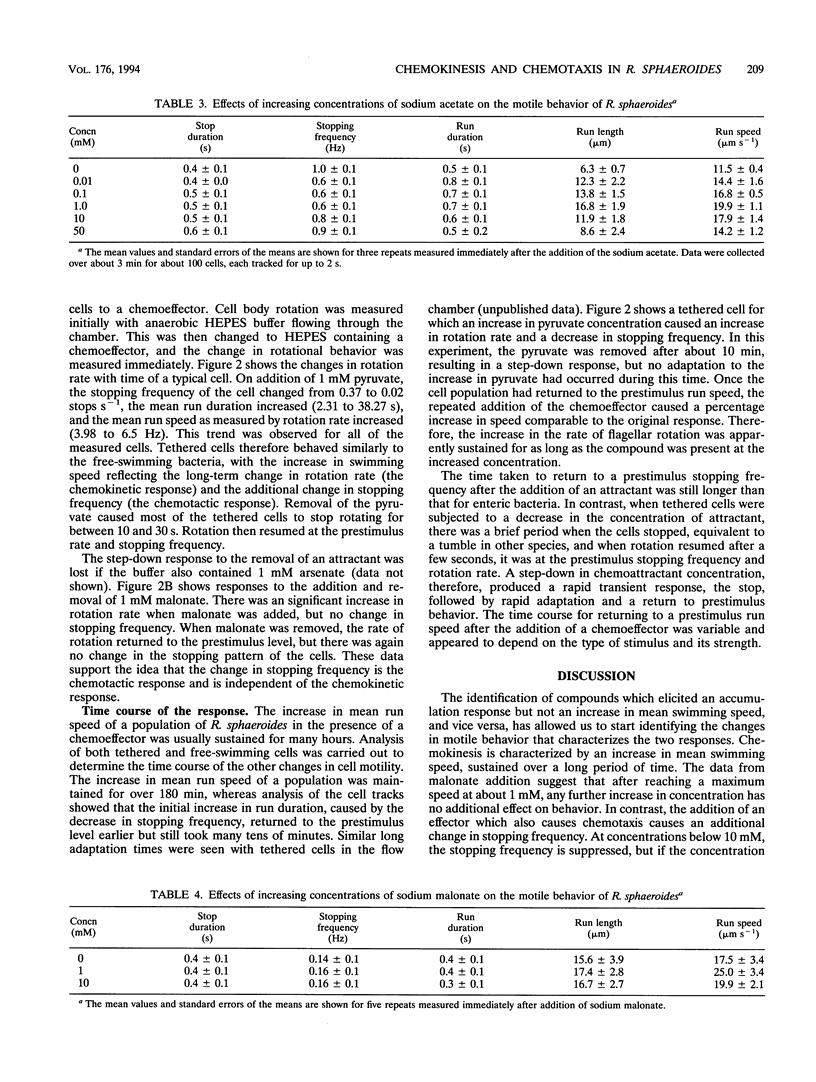
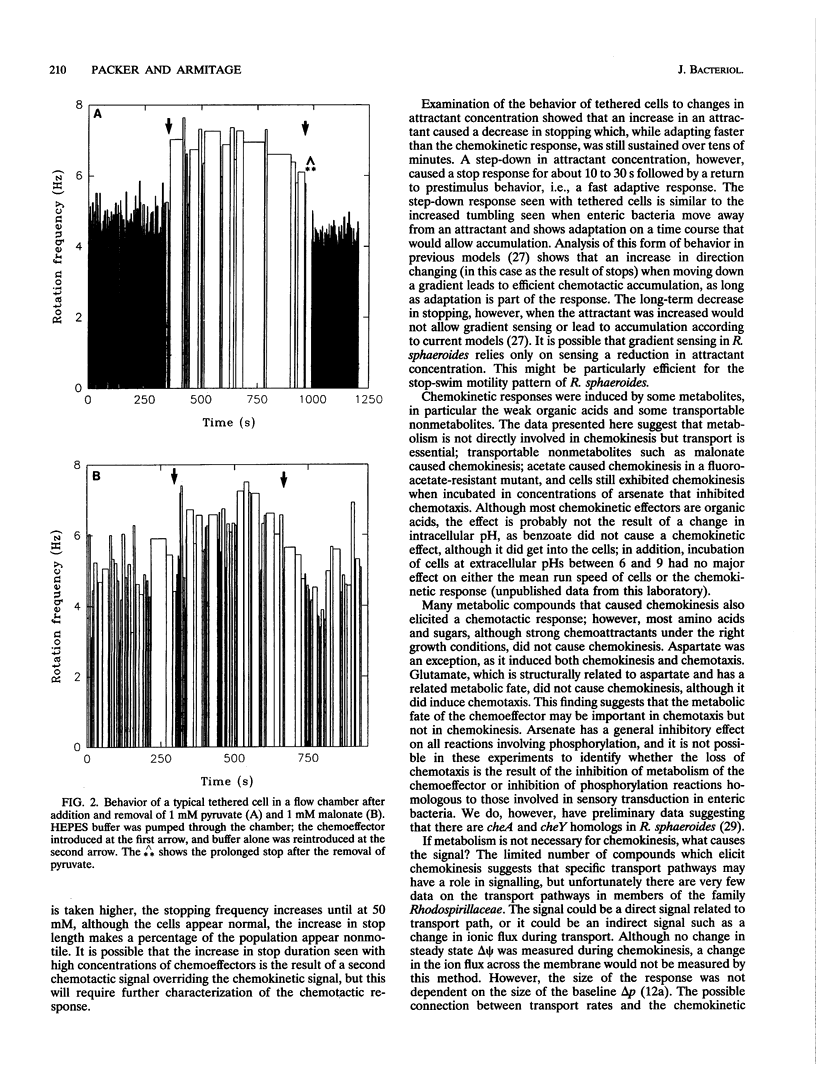
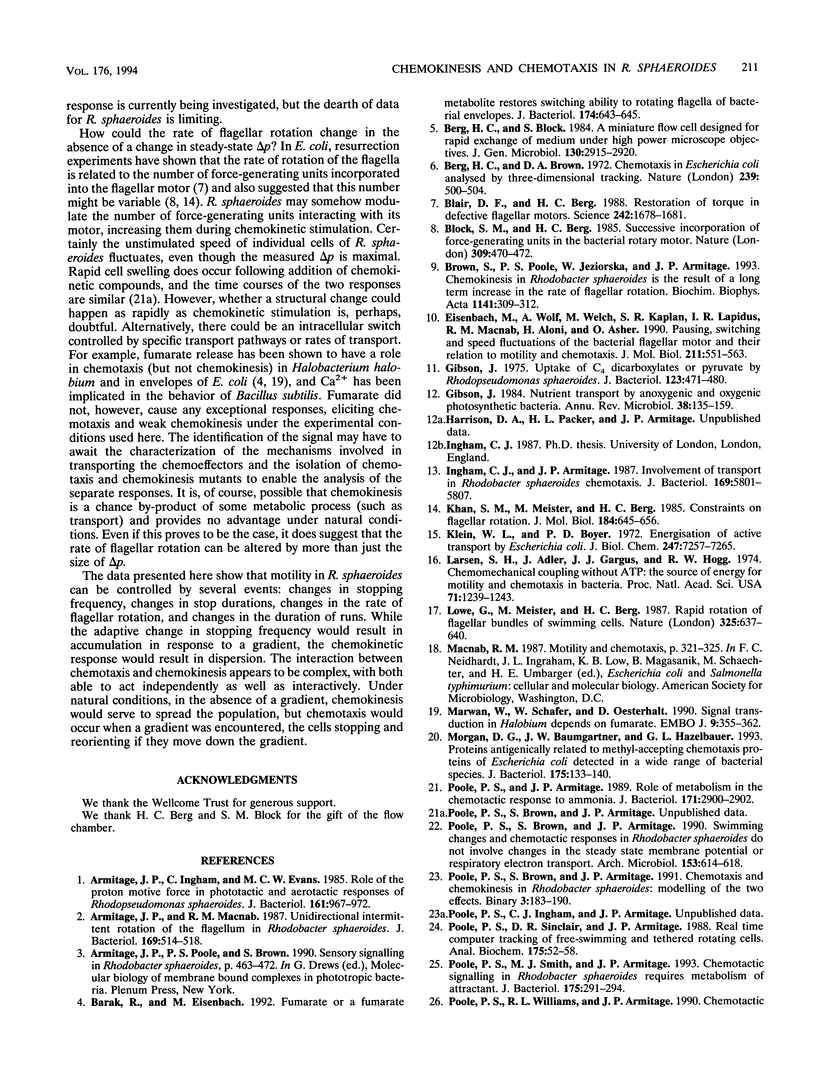
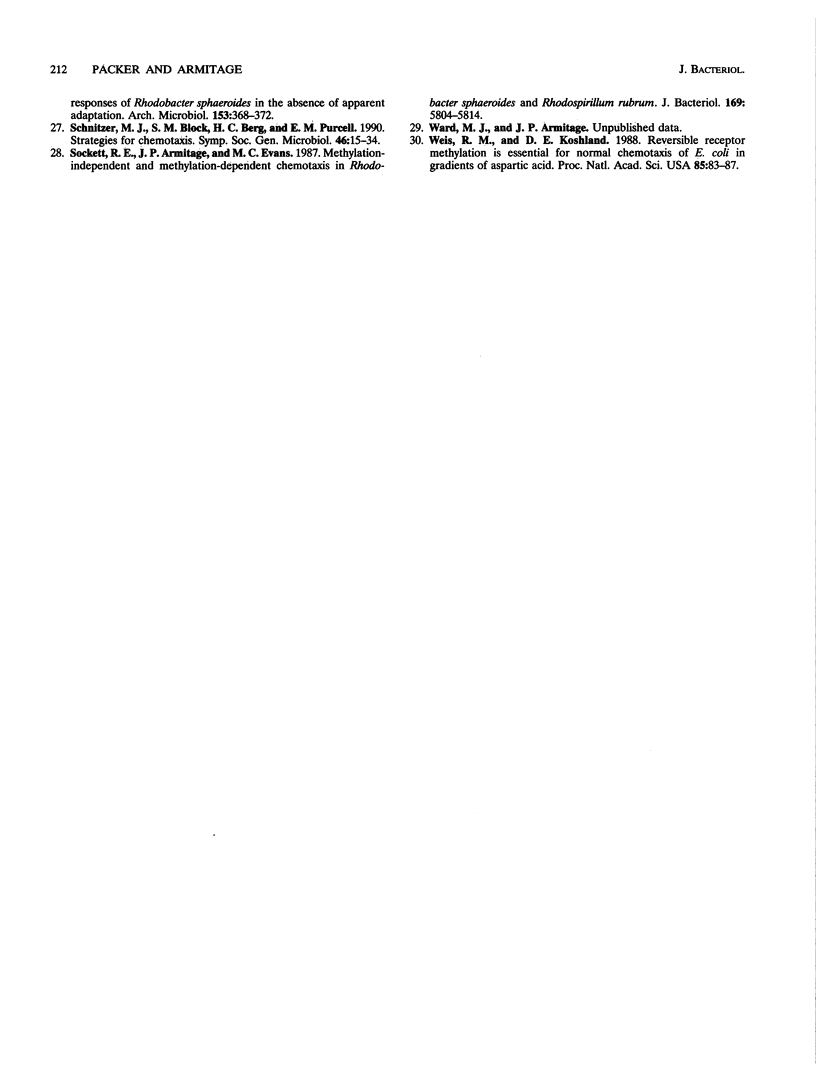
Selected References
These references are in PubMed. This may not be the complete list of references from this article.
- Armitage J. P., Ingham C., Evans M. C. Role of proton motive force in phototactic and aerotactic responses of Rhodopseudomonas sphaeroides. J Bacteriol. 1985 Mar;161(3):967–972. doi: 10.1128/jb.161.3.967-972.1985. [DOI] [PMC free article] [PubMed] [Google Scholar]
- Armitage J. P., Macnab R. M. Unidirectional, intermittent rotation of the flagellum of Rhodobacter sphaeroides. J Bacteriol. 1987 Feb;169(2):514–518. doi: 10.1128/jb.169.2.514-518.1987. [DOI] [PMC free article] [PubMed] [Google Scholar]
- Barak R., Eisenbach M. Fumarate or a fumarate metabolite restores switching ability to rotating flagella of bacterial envelopes. J Bacteriol. 1992 Jan;174(2):643–645. doi: 10.1128/jb.174.2.643-645.1992. [DOI] [PMC free article] [PubMed] [Google Scholar]
- Berg H. C., Block S. M. A miniature flow cell designed for rapid exchange of media under high-power microscope objectives. J Gen Microbiol. 1984 Nov;130(11):2915–2920. doi: 10.1099/00221287-130-11-2915. [DOI] [PubMed] [Google Scholar]
- Berg H. C., Brown D. A. Chemotaxis in Escherichia coli analysed by three-dimensional tracking. Nature. 1972 Oct 27;239(5374):500–504. doi: 10.1038/239500a0. [DOI] [PubMed] [Google Scholar]
- Blair D. F., Berg H. C. Restoration of torque in defective flagellar motors. Science. 1988 Dec 23;242(4886):1678–1681. doi: 10.1126/science.2849208. [DOI] [PubMed] [Google Scholar]
- Block S. M., Berg H. C. Successive incorporation of force-generating units in the bacterial rotary motor. 1984 May 31-Jun 6Nature. 309(5967):470–472. doi: 10.1038/309470a0. [DOI] [PubMed] [Google Scholar]
- Eisenbach M., Wolf A., Welch M., Caplan S. R., Lapidus I. R., Macnab R. M., Aloni H., Asher O. Pausing, switching and speed fluctuation of the bacterial flagellar motor and their relation to motility and chemotaxis. J Mol Biol. 1990 Feb 5;211(3):551–563. doi: 10.1016/0022-2836(90)90265-N. [DOI] [PubMed] [Google Scholar]
- Gibson J. Nutrient transport by anoxygenic and oxygenic photosynthetic bacteria. Annu Rev Microbiol. 1984;38:135–159. doi: 10.1146/annurev.mi.38.100184.001031. [DOI] [PubMed] [Google Scholar]
- Gibson J. Uptake of C4 dicarboxylates and pyruvate by Rhodopseudomonas spheroides. J Bacteriol. 1975 Aug;123(2):471–480. doi: 10.1128/jb.123.2.471-480.1975. [DOI] [PMC free article] [PubMed] [Google Scholar]
- Ingham C. J., Armitage J. P. Involvement of transport in Rhodobacter sphaeroides chemotaxis. J Bacteriol. 1987 Dec;169(12):5801–5807. doi: 10.1128/jb.169.12.5801-5807.1987. [DOI] [PMC free article] [PubMed] [Google Scholar]
- Khan S., Meister M., Berg H. C. Constraints on flagellar rotation. J Mol Biol. 1985 Aug 20;184(4):645–656. doi: 10.1016/0022-2836(85)90310-9. [DOI] [PubMed] [Google Scholar]
- Klein W. L., Boyer P. D. Energization of active transport by Escherichia coli. J Biol Chem. 1972 Nov 25;247(22):7257–7265. [PubMed] [Google Scholar]
- Larsen S. H., Adler J., Gargus J. J., Hogg R. W. Chemomechanical coupling without ATP: the source of energy for motility and chemotaxis in bacteria. Proc Natl Acad Sci U S A. 1974 Apr;71(4):1239–1243. doi: 10.1073/pnas.71.4.1239. [DOI] [PMC free article] [PubMed] [Google Scholar]
- Marwan W., Schäfer W., Oesterhelt D. Signal transduction in Halobacterium depends on fumarate. EMBO J. 1990 Feb;9(2):355–362. doi: 10.1002/j.1460-2075.1990.tb08118.x. [DOI] [PMC free article] [PubMed] [Google Scholar]
- Morgan D. G., Baumgartner J. W., Hazelbauer G. L. Proteins antigenically related to methyl-accepting chemotaxis proteins of Escherichia coli detected in a wide range of bacterial species. J Bacteriol. 1993 Jan;175(1):133–140. doi: 10.1128/jb.175.1.133-140.1993. [DOI] [PMC free article] [PubMed] [Google Scholar]
- Poole P. S., Armitage J. P. Role of metabolism in the chemotactic response of Rhodobacter sphaeroides to ammonia. J Bacteriol. 1989 May;171(5):2900–2902. doi: 10.1128/jb.171.5.2900-2902.1989. [DOI] [PMC free article] [PubMed] [Google Scholar]
- Poole P. S., Sinclair D. R., Armitage J. P. Real time computer tracking of free-swimming and tethered rotating cells. Anal Biochem. 1988 Nov 15;175(1):52–58. doi: 10.1016/0003-2697(88)90359-4. [DOI] [PubMed] [Google Scholar]
- Poole P. S., Smith M. J., Armitage J. P. Chemotactic signalling in Rhodobacter sphaeroides requires metabolism of attractants. J Bacteriol. 1993 Jan;175(1):291–294. doi: 10.1128/jb.175.1.291-294.1993. [DOI] [PMC free article] [PubMed] [Google Scholar]
- Sockett R. E., Armitage J. P., Evans M. C. Methylation-independent and methylation-dependent chemotaxis in Rhodobacter sphaeroides and Rhodospirillum rubrum. J Bacteriol. 1987 Dec;169(12):5808–5814. doi: 10.1128/jb.169.12.5808-5814.1987. [DOI] [PMC free article] [PubMed] [Google Scholar]
- Weis R. M., Koshland D. E., Jr Reversible receptor methylation is essential for normal chemotaxis of Escherichia coli in gradients of aspartic acid. Proc Natl Acad Sci U S A. 1988 Jan;85(1):83–87. doi: 10.1073/pnas.85.1.83. [DOI] [PMC free article] [PubMed] [Google Scholar]