Abstract
Directional growth in response to electric fields (galvanotropism) is known for eukaryotic cells as diverse as fibroblasts, neurons, algae, and fungal hyphae. The mechanism is not understood, but all proposals invoke actin either directly or indirectly. We applied electric fields to bacteria (which are inherently free of actin) to determine whether actin was essential for galvanotropism. Field-treated (but not control) Enterobacter cloacae and Escherichia coli cells curved rapidly toward the anode. The response was both field strength and pH dependent. The direction of curvature was reversed upon reversal of field polarity. The directional growth was not due to passive bending of the cells or to field-induced gradients of tropic substances in the medium. Field-treated Bacillus subtilis cells also curved, but the threshold was much higher than for E. cloacae or E. coli. Since the curved morphology must reflect spatial differences in the rates of cell wall synthesis and degradation, we looked for regions of active wall growth. Experiments in which the cells were decorated with latex beads revealed that the anode-facing ends of cells grew faster than the cathode-facing ends of the same cells. Inhibitors of cell wall synthesis caused spheroplasts to form on the convex regions of field-treated cells, suggesting that the initial curvature resulted from enhanced growth of cathode-facing regions. Our results indicate that an electric field modulates wall growth spatially and that the mechanism may involve differential stimulation of wall growth in both anode- and cathode-facing regions. Electric fields may therefore serve as valuable tools for studies of bacterial wall growth. Use of specific E. coli mutants may allow dissection of the galvanotropic mechanism at the molecular level.
Full text
PDF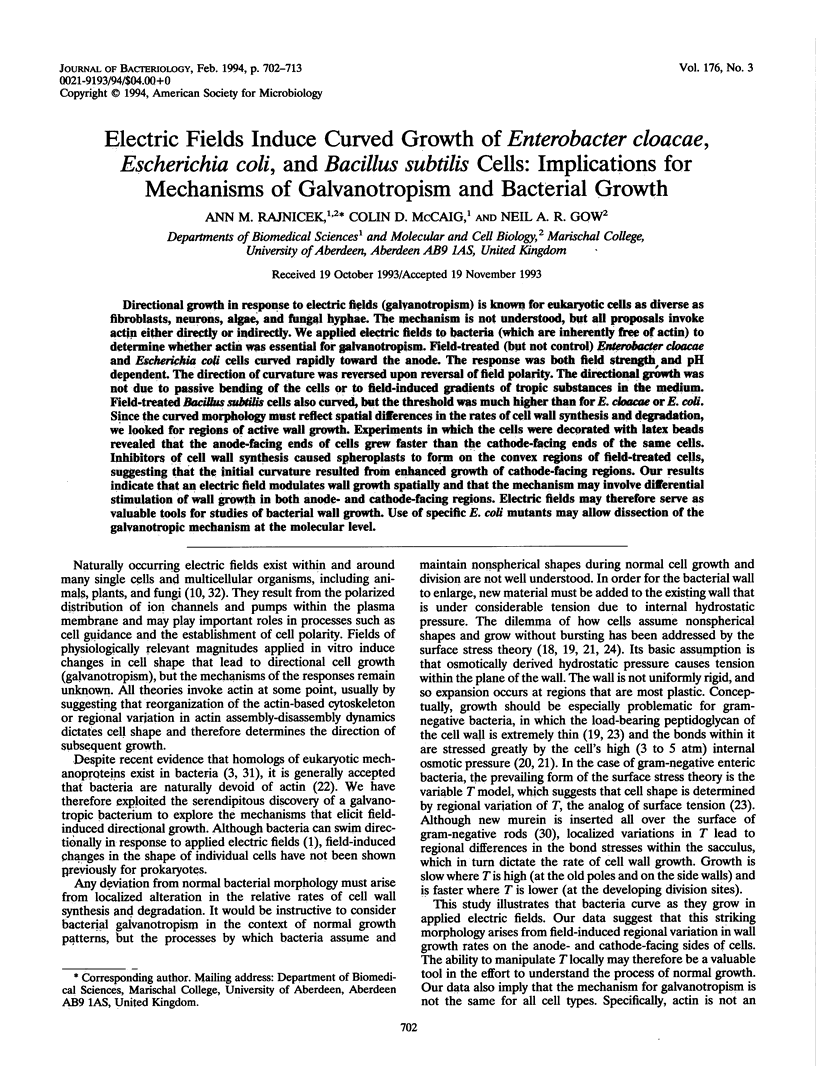
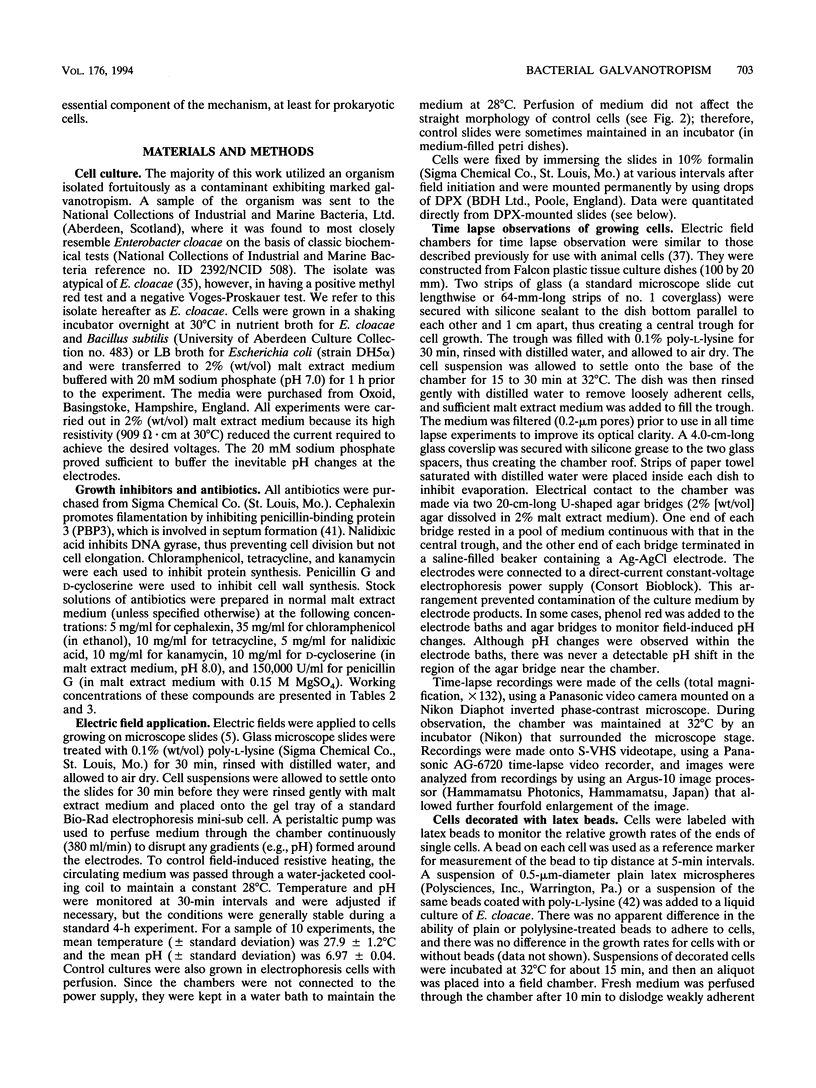
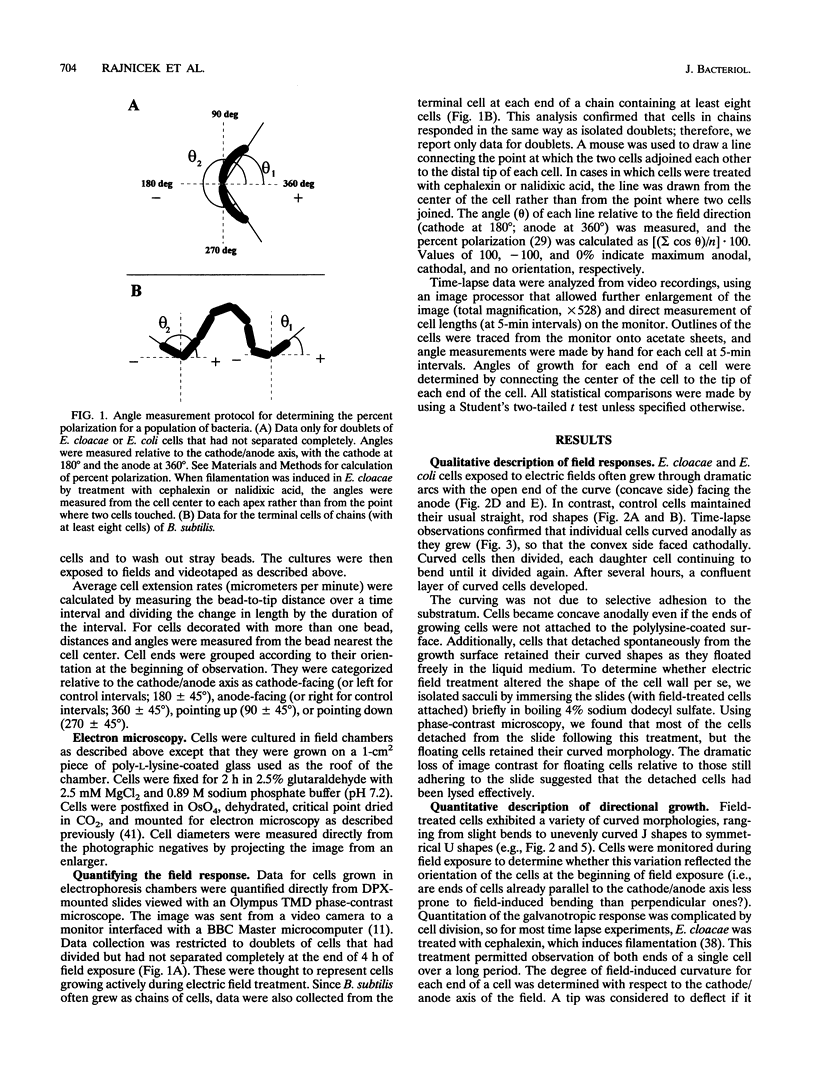
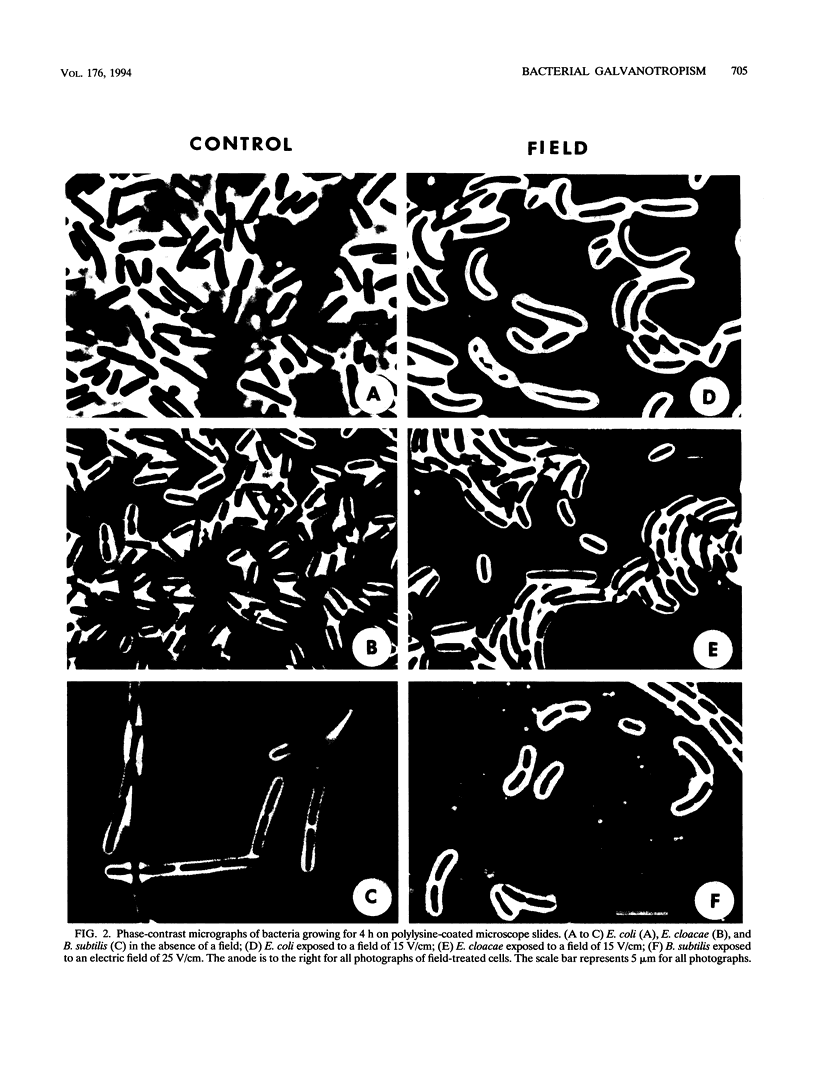
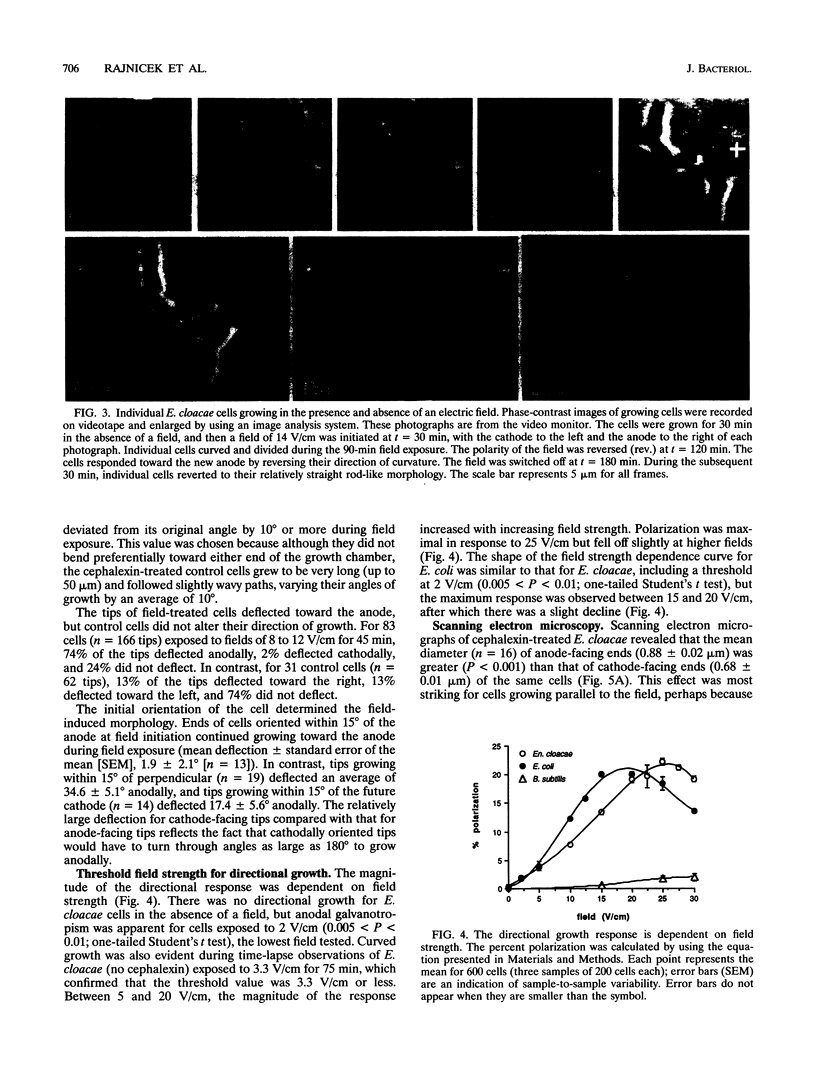
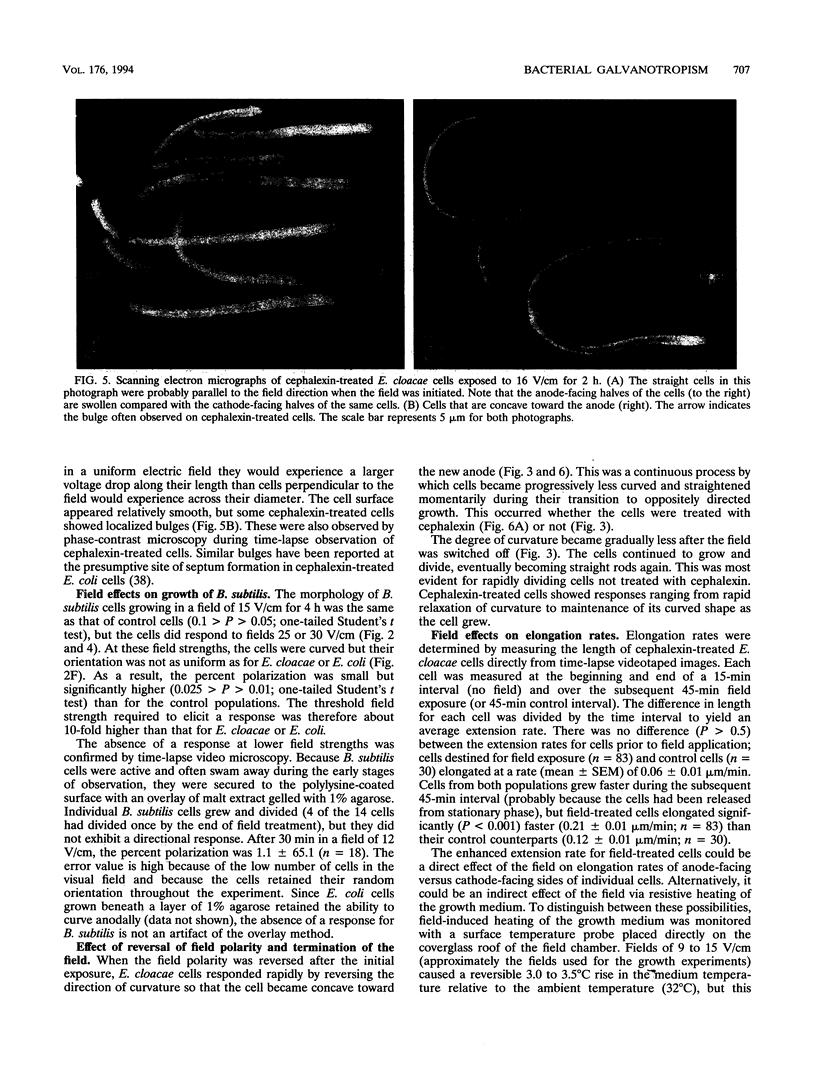
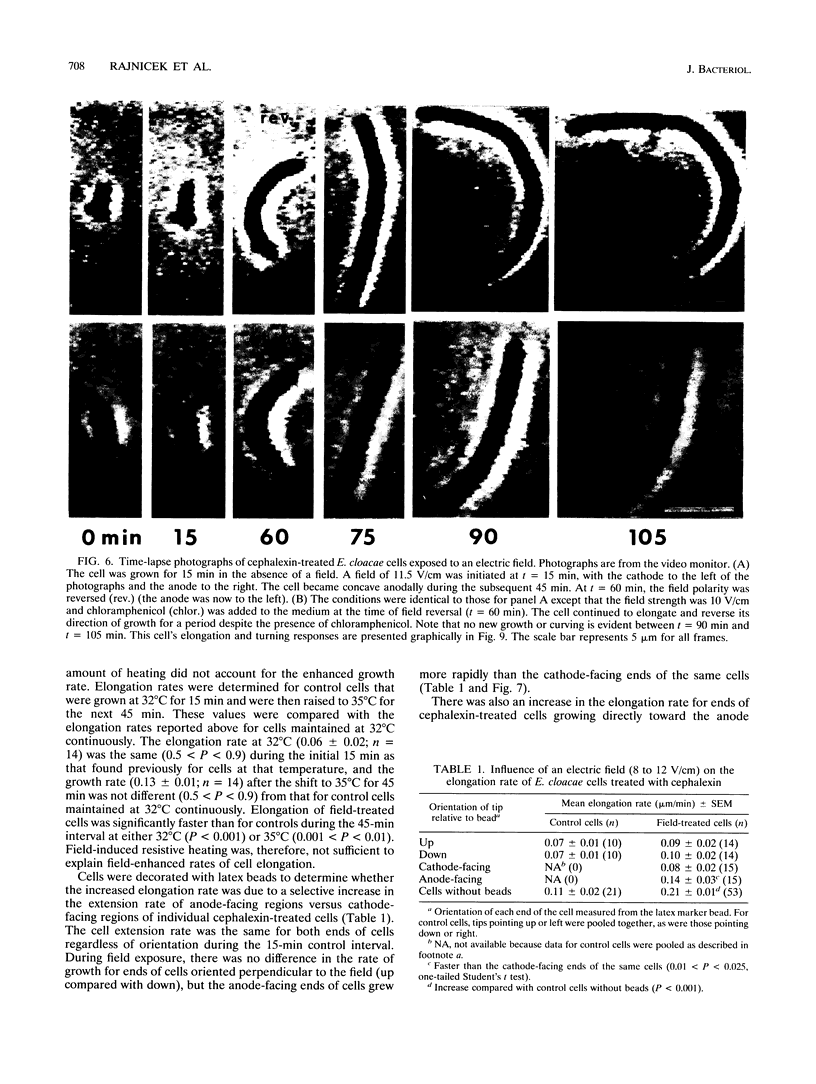
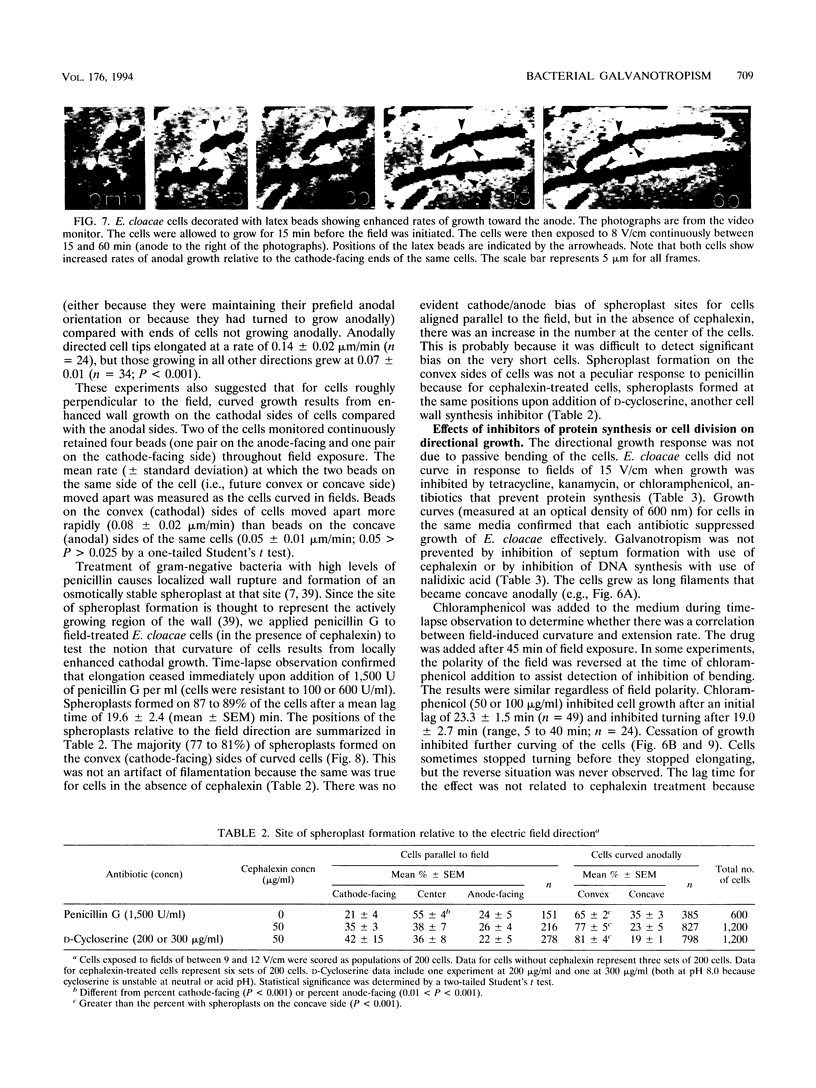
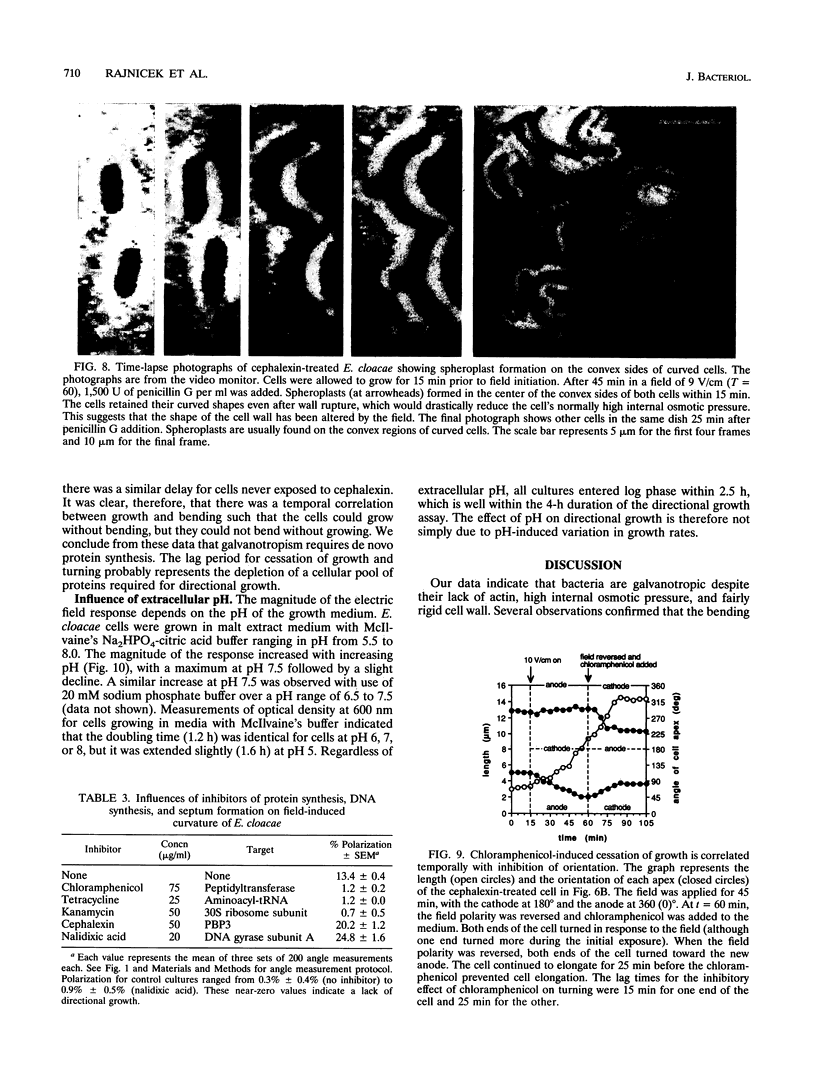
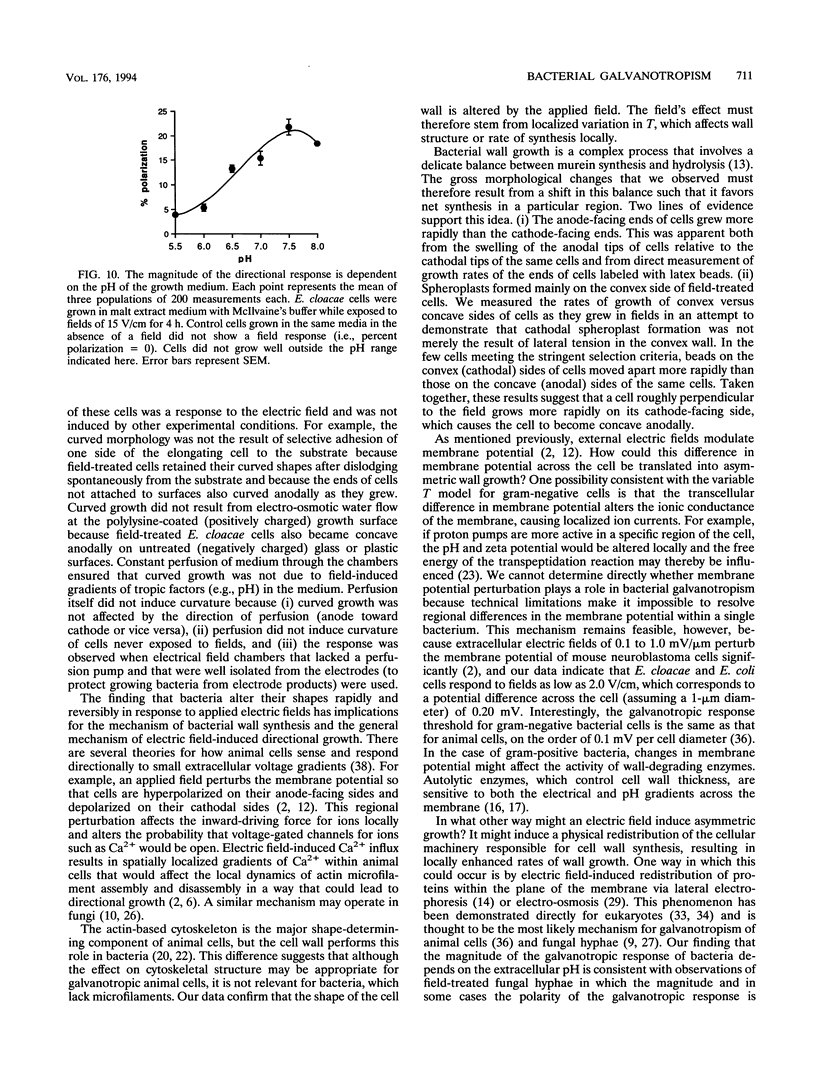
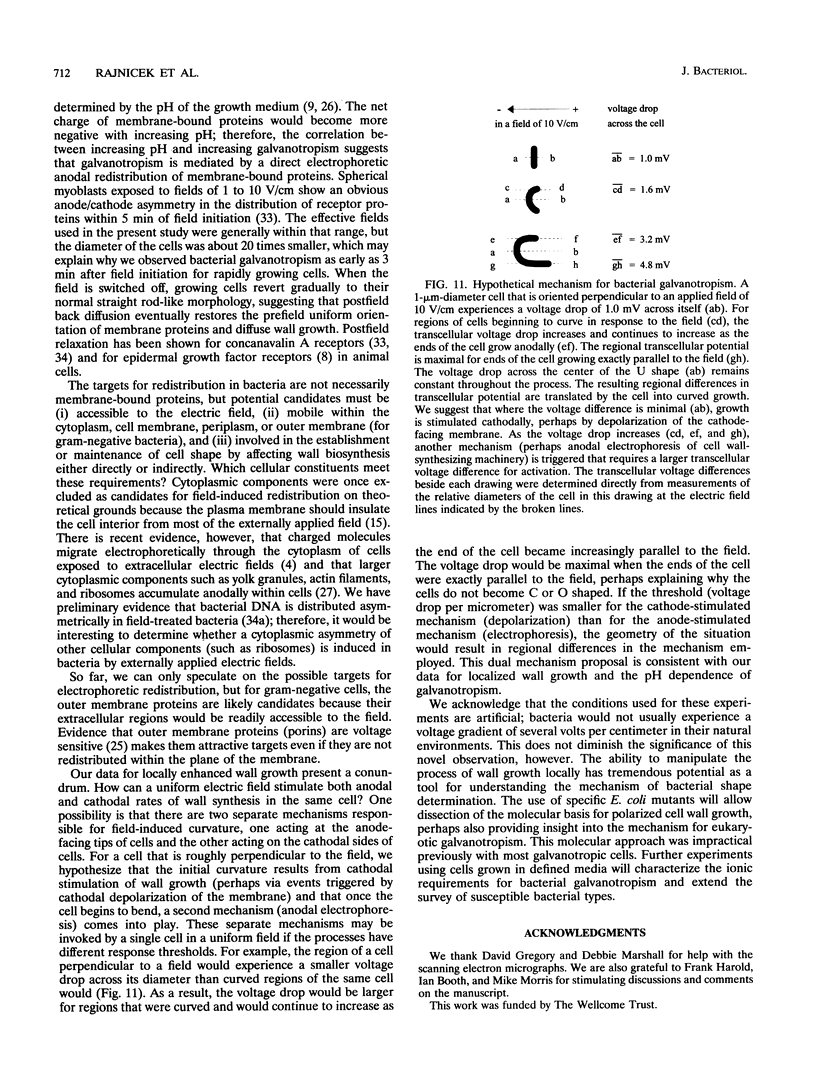
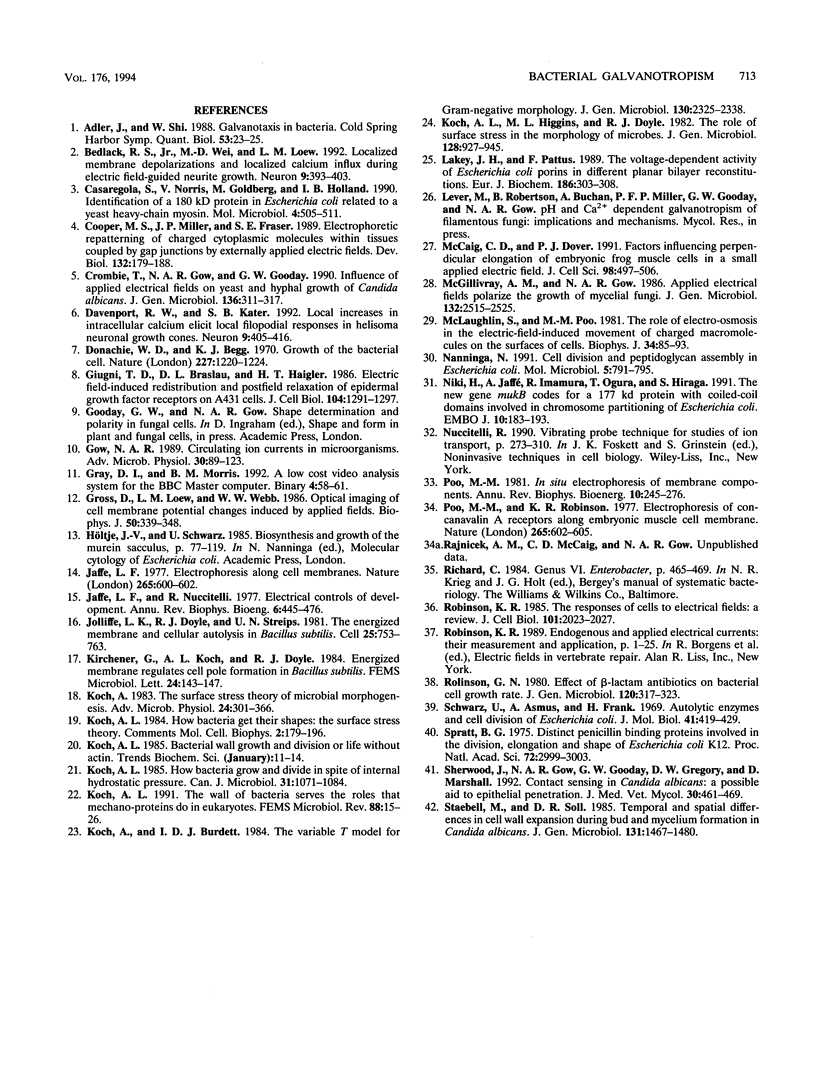
Images in this article
Selected References
These references are in PubMed. This may not be the complete list of references from this article.
- Adler J., Shi W. Galvanotaxis in bacteria. Cold Spring Harb Symp Quant Biol. 1988;53(Pt 1):23–25. doi: 10.1101/sqb.1988.053.01.006. [DOI] [PubMed] [Google Scholar]
- Bedlack R. S., Jr, Wei M., Loew L. M. Localized membrane depolarizations and localized calcium influx during electric field-guided neurite growth. Neuron. 1992 Sep;9(3):393–403. doi: 10.1016/0896-6273(92)90178-g. [DOI] [PubMed] [Google Scholar]
- Casaregola S., Norris V., Goldberg M., Holland I. B. Identification of a 180 kD protein in Escherichia coli related to a yeast heavy-chain myosin. Mol Microbiol. 1990 Mar;4(3):505–511. doi: 10.1111/j.1365-2958.1990.tb00617.x. [DOI] [PubMed] [Google Scholar]
- Cooper M. S., Miller J. P., Fraser S. E. Electrophoretic repatterning of charged cytoplasmic molecules within tissues coupled by gap junctions by externally applied electric fields. Dev Biol. 1989 Mar;132(1):179–188. doi: 10.1016/0012-1606(89)90216-9. [DOI] [PubMed] [Google Scholar]
- Crombie T., Gow N. A., Gooday G. W. Influence of applied electrical fields on yeast and hyphal growth of Candida albicans. J Gen Microbiol. 1990 Feb;136(2):311–317. doi: 10.1099/00221287-136-2-311. [DOI] [PubMed] [Google Scholar]
- Davenport R. W., Kater S. B. Local increases in intracellular calcium elicit local filopodial responses in Helisoma neuronal growth cones. Neuron. 1992 Sep;9(3):405–416. doi: 10.1016/0896-6273(92)90179-h. [DOI] [PubMed] [Google Scholar]
- Donachie W. D., Begg K. J. Growth of the bacterial cell. Nature. 1970 Sep 19;227(5264):1220–1224. doi: 10.1038/2271220a0. [DOI] [PubMed] [Google Scholar]
- Giugni T. D., Braslau D. L., Haigler H. T. Electric field-induced redistribution and postfield relaxation of epidermal growth factor receptors on A431 cells. J Cell Biol. 1987 May;104(5):1291–1297. doi: 10.1083/jcb.104.5.1291. [DOI] [PMC free article] [PubMed] [Google Scholar]
- Gow N. A. Circulating ionic currents in micro-organisms. Adv Microb Physiol. 1989;30:89–123. doi: 10.1016/s0065-2911(08)60111-3. [DOI] [PubMed] [Google Scholar]
- Gross D., Loew L. M., Webb W. W. Optical imaging of cell membrane potential changes induced by applied electric fields. Biophys J. 1986 Aug;50(2):339–348. doi: 10.1016/S0006-3495(86)83467-1. [DOI] [PMC free article] [PubMed] [Google Scholar]
- Jaffe L. F. Electrophoresis along cell membranes. Nature. 1977 Feb 17;265(5595):600–602. doi: 10.1038/265600a0. [DOI] [PubMed] [Google Scholar]
- Jaffe L. F., Nuccitelli R. Electrical controls of development. Annu Rev Biophys Bioeng. 1977;6:445–476. doi: 10.1146/annurev.bb.06.060177.002305. [DOI] [PubMed] [Google Scholar]
- Jolliffe L. K., Doyle R. J., Streips U. N. The energized membrane and cellular autolysis in Bacillus subtilis. Cell. 1981 Sep;25(3):753–763. doi: 10.1016/0092-8674(81)90183-5. [DOI] [PubMed] [Google Scholar]
- Koch A. L., Burdett I. D. The variable T model for gram-negative morphology. J Gen Microbiol. 1984 Sep;130(9):2325–2338. doi: 10.1099/00221287-130-9-2325. [DOI] [PubMed] [Google Scholar]
- Koch A. L., Higgins M. L., Doyle R. J. The role of surface stress in the morphology of microbes. J Gen Microbiol. 1982 May;128(5):927–945. doi: 10.1099/00221287-128-5-927. [DOI] [PubMed] [Google Scholar]
- Koch A. L. How bacteria grow and divide in spite of internal hydrostatic pressure. Can J Microbiol. 1985 Dec;31(12):1071–1084. doi: 10.1139/m85-204. [DOI] [PubMed] [Google Scholar]
- Koch A. L. The surface stress theory of microbial morphogenesis. Adv Microb Physiol. 1983;24:301–366. doi: 10.1016/s0065-2911(08)60388-4. [DOI] [PubMed] [Google Scholar]
- Koch A. L. The wall of bacteria serves the roles that mechano-proteins do in eukaryotes. FEMS Microbiol Rev. 1991 Jul;8(1):15–25. doi: 10.1111/j.1574-6968.1991.tb04954.x. [DOI] [PubMed] [Google Scholar]
- Lakey J. H., Pattus F. The voltage-dependent activity of Escherichia coli porins in different planar bilayer reconstitutions. Eur J Biochem. 1989 Dec 8;186(1-2):303–308. doi: 10.1111/j.1432-1033.1989.tb15209.x. [DOI] [PubMed] [Google Scholar]
- McCaig C. D., Dover P. J. Factors influencing perpendicular elongation of embryonic frog muscle cells in a small applied electric field. J Cell Sci. 1991 Apr;98(Pt 4):497–506. doi: 10.1242/jcs.98.4.497. [DOI] [PubMed] [Google Scholar]
- McLaughlin S., Poo M. M. The role of electro-osmosis in the electric-field-induced movement of charged macromolecules on the surfaces of cells. Biophys J. 1981 Apr;34(1):85–93. doi: 10.1016/S0006-3495(81)84838-2. [DOI] [PMC free article] [PubMed] [Google Scholar]
- Nanninga N. Cell division and peptidoglycan assembly in Escherichia coli. Mol Microbiol. 1991 Apr;5(4):791–795. doi: 10.1111/j.1365-2958.1991.tb00751.x. [DOI] [PubMed] [Google Scholar]
- Niki H., Jaffé A., Imamura R., Ogura T., Hiraga S. The new gene mukB codes for a 177 kd protein with coiled-coil domains involved in chromosome partitioning of E. coli. EMBO J. 1991 Jan;10(1):183–193. doi: 10.1002/j.1460-2075.1991.tb07935.x. [DOI] [PMC free article] [PubMed] [Google Scholar]
- Poo M. In situ electrophoresis of membrane components. Annu Rev Biophys Bioeng. 1981;10:245–276. doi: 10.1146/annurev.bb.10.060181.001333. [DOI] [PubMed] [Google Scholar]
- Poo M., Robinson K. R. Electrophoresis of concanavalin A receptors along embryonic muscle cell membrane. Nature. 1977 Feb 17;265(5595):602–605. doi: 10.1038/265602a0. [DOI] [PubMed] [Google Scholar]
- Robinson K. R. The responses of cells to electrical fields: a review. J Cell Biol. 1985 Dec;101(6):2023–2027. doi: 10.1083/jcb.101.6.2023. [DOI] [PMC free article] [PubMed] [Google Scholar]
- Rolinson G. N. Effect of beta-lactam antibiotics on bacterial cell growth rate. J Gen Microbiol. 1980 Oct;120(2):317–323. doi: 10.1099/00221287-120-2-317. [DOI] [PubMed] [Google Scholar]
- Schwarz U., Asmus A., Frank H. Autolytic enzymes and cell division of Escherichia coli. J Mol Biol. 1969 May 14;41(3):419–429. doi: 10.1016/0022-2836(69)90285-x. [DOI] [PubMed] [Google Scholar]
- Sherwood J., Gow N. A., Gooday G. W., Gregory D. W., Marshall D. Contact sensing in Candida albicans: a possible aid to epithelial penetration. J Med Vet Mycol. 1992;30(6):461–469. doi: 10.1080/02681219280000621. [DOI] [PubMed] [Google Scholar]
- Spratt B. G. Distinct penicillin binding proteins involved in the division, elongation, and shape of Escherichia coli K12. Proc Natl Acad Sci U S A. 1975 Aug;72(8):2999–3003. doi: 10.1073/pnas.72.8.2999. [DOI] [PMC free article] [PubMed] [Google Scholar]
- Staebell M., Soll D. R. Temporal and spatial differences in cell wall expansion during bud and mycelium formation in Candida albicans. J Gen Microbiol. 1985 Jun;131(6):1467–1480. doi: 10.1099/00221287-131-6-1467. [DOI] [PubMed] [Google Scholar]