Abstract
Proton motive force (PMF), intracellular end product concentrations, and ATP levels were determined when a steady-state Lactobacillus plantarum 8014 anaerobic chemostat culture was shifted to an aerobic condition or was shifted from pH 5.5 to 7.5. The PMF and intracellular ATP levels increased immediately after the culture was shifted from anaerobic to aerobic conditions. The concentrations of intracellular lactate and acetate, which exported protons that contributed to the proton gradient, changed in the same fashion. The H+/lactate stoichiometry, n, varied from 0.8 to 1.2, and the H+/acetate n value changed from 0.8 to 1.6 at 2 h after the shift to aerobic conditions. The n value for acetate excretion remained elevated at aerobic steady state. When the anaerobic culture was shifted from pH 5.5 to 7.5, intracellular ATP increased 20% immediately even though the PMF decreased 50% as a result of the depletion of the transmembrane proton gradient. The H+/lactate n value changed from 0.7 to 1.8, and n for H+/acetate increased from 0.9 to 1.9 at pH 7.5 steady state. In addition, the H+/acetate stoichiometry was always higher than the n value for H+/lactate; both were higher in alkaline than aerobic conditions, demonstrating that L. plantarum 8014 coexcreted more protons with end products to maintain intracellular pH homeostasis and generate proton gradients under aerobic and alkaline conditions. During the transient to pH 7.5, the n value for H+/acetate approached 3, which would spare one ATP.
Full text
PDF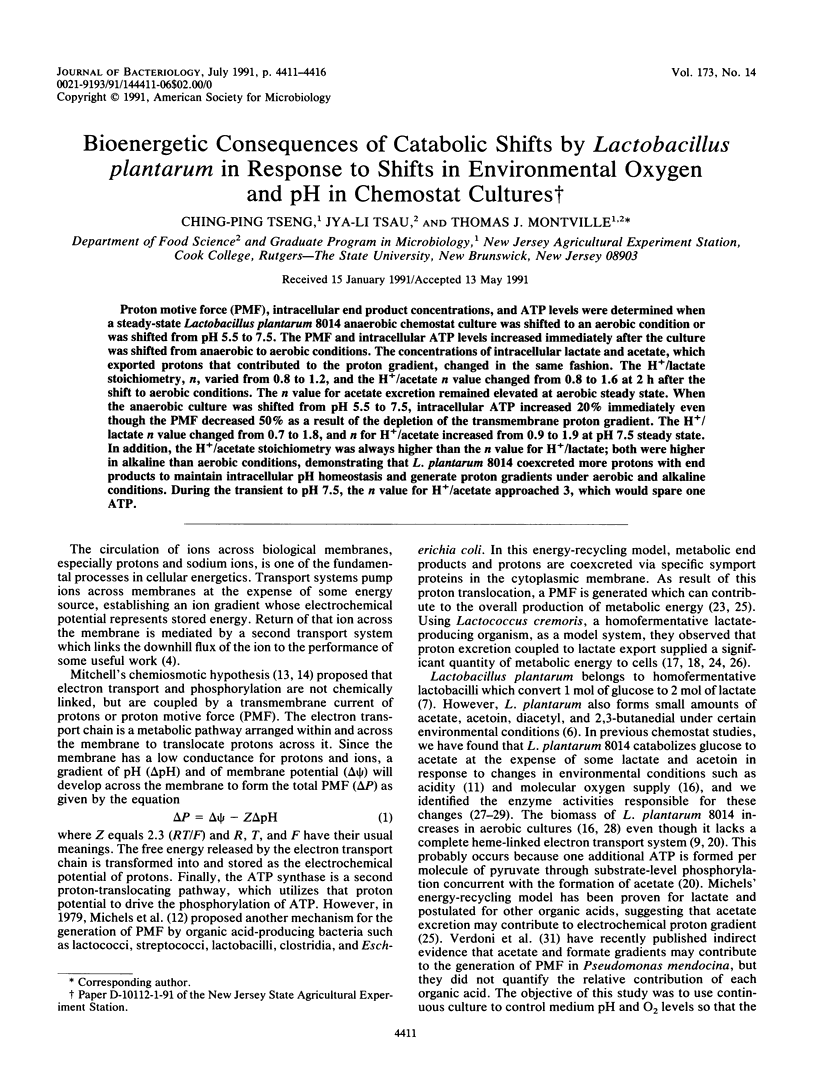
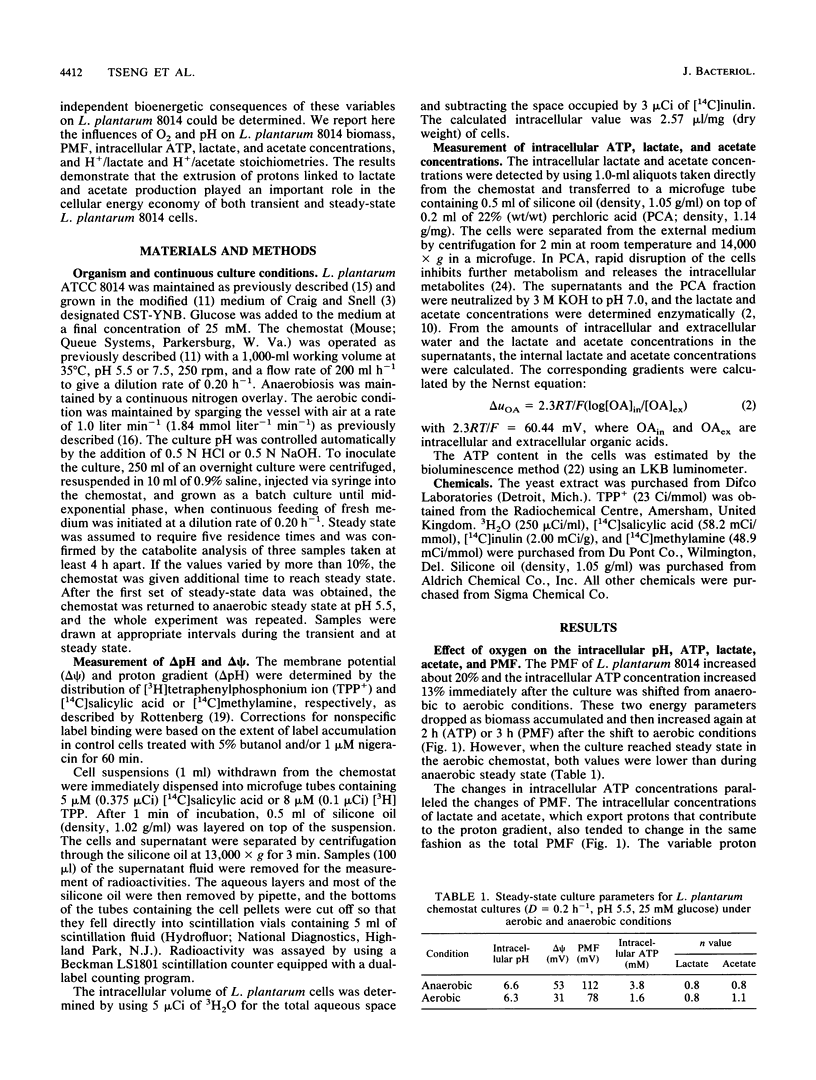
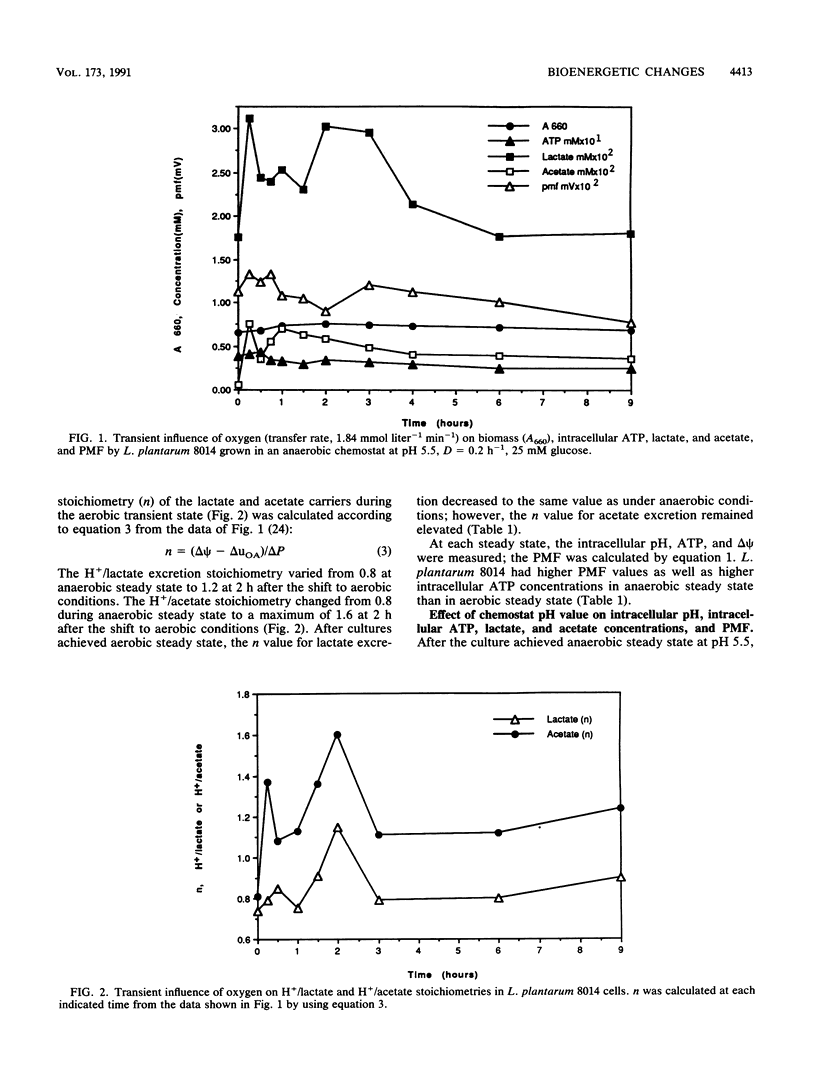
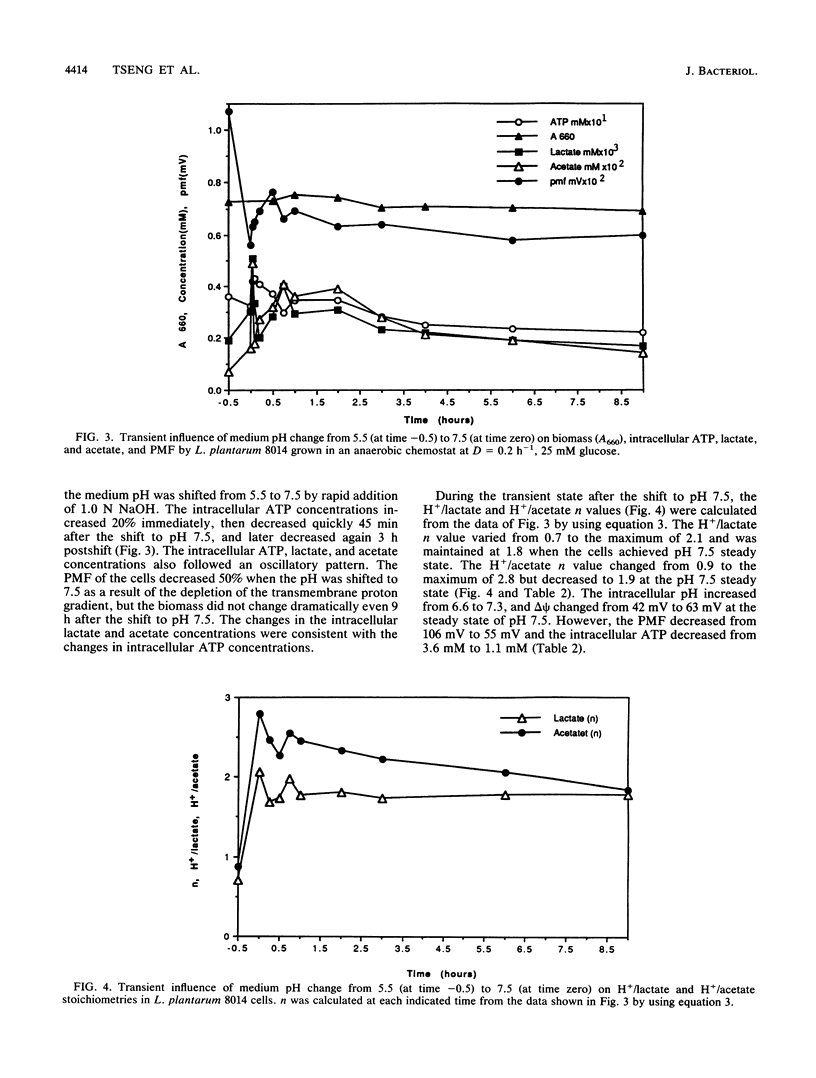
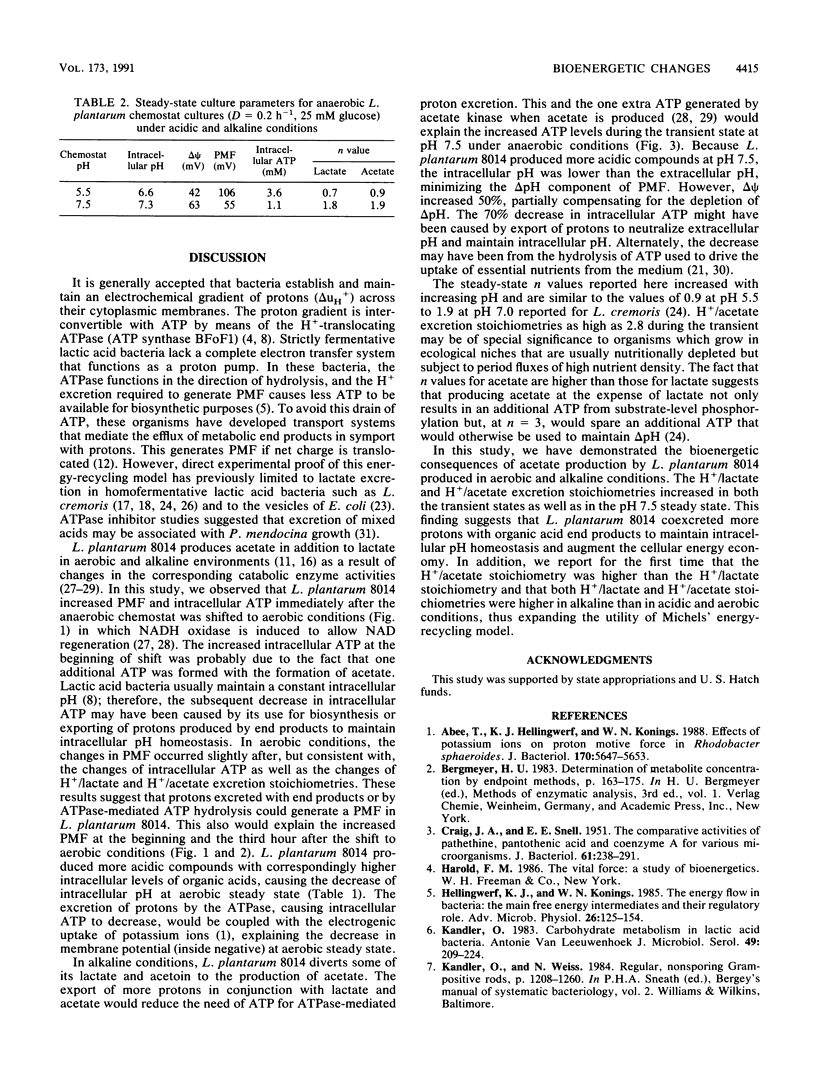
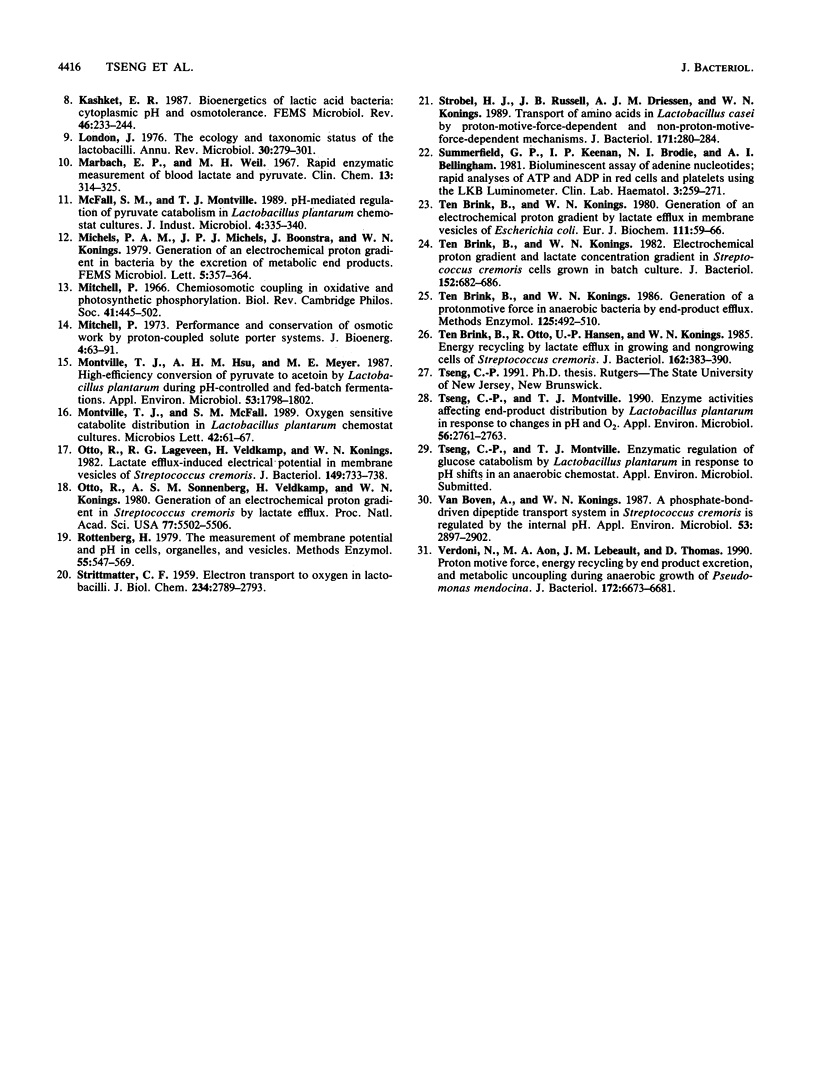
Selected References
These references are in PubMed. This may not be the complete list of references from this article.
- Abee T., Hellingwerf K. J., Konings W. N. Effects of potassium ions on proton motive force in Rhodobacter sphaeroides. J Bacteriol. 1988 Dec;170(12):5647–5653. doi: 10.1128/jb.170.12.5647-5653.1988. [DOI] [PMC free article] [PubMed] [Google Scholar]
- CRAIG J. A., SNELL E. E. The comparative activities of pantethine, pantothenic acid, and coenzyme A for various microorganisms. J Bacteriol. 1951 Mar;61(3):283–291. doi: 10.1128/jb.61.3.283-291.1951. [DOI] [PMC free article] [PubMed] [Google Scholar]
- Hellingwerf K. J., Konings W. N. The energy flow in bacteria: the main free energy intermediates and their regulatory role. Adv Microb Physiol. 1985;26:125–154. doi: 10.1016/s0065-2911(08)60396-3. [DOI] [PubMed] [Google Scholar]
- Kandler O. Carbohydrate metabolism in lactic acid bacteria. Antonie Van Leeuwenhoek. 1983 Sep;49(3):209–224. doi: 10.1007/BF00399499. [DOI] [PubMed] [Google Scholar]
- London J. The ecology and taxonomic status of the lactobacilli. Annu Rev Microbiol. 1976;30:279–301. doi: 10.1146/annurev.mi.30.100176.001431. [DOI] [PubMed] [Google Scholar]
- Marbach E. P., Weil M. H. Rapid enzymatic measurement of blood lactate and pyruvate. Use and significance of metaphosphoric acid as a common precipitant. Clin Chem. 1967 Apr;13(4):314–325. [PubMed] [Google Scholar]
- Mitchell P. Chemiosmotic coupling in oxidative and photosynthetic phosphorylation. Biol Rev Camb Philos Soc. 1966 Aug;41(3):445–502. doi: 10.1111/j.1469-185x.1966.tb01501.x. [DOI] [PubMed] [Google Scholar]
- Mitchell P. Performance and conservation of osmotic work by proton-coupled solute porter systems. J Bioenerg. 1973 Jan;4(1):63–91. doi: 10.1007/BF01516051. [DOI] [PubMed] [Google Scholar]
- Montville T. J., Hsu A. H., Meyer M. E. High-Efficiency Conversion of Pyruvate to Acetoin by Lactobacillus plantarum during pH-Controlled and Fed-Batch Fermentations. Appl Environ Microbiol. 1987 Aug;53(8):1798–1802. doi: 10.1128/aem.53.8.1798-1802.1987. [DOI] [PMC free article] [PubMed] [Google Scholar]
- Otto R., Lageveen R. G., Veldkamp H., Konings W. N. Lactate efflux-induced electrical potential in membrane vesicles of Streptococcus cremoris. J Bacteriol. 1982 Feb;149(2):733–738. doi: 10.1128/jb.149.2.733-738.1982. [DOI] [PMC free article] [PubMed] [Google Scholar]
- Otto R., Sonnenberg A. S., Veldkamp H., Konings W. N. Generation of an electrochemical proton gradient in Streptococcus cremoris by lactate efflux. Proc Natl Acad Sci U S A. 1980 Sep;77(9):5502–5506. doi: 10.1073/pnas.77.9.5502. [DOI] [PMC free article] [PubMed] [Google Scholar]
- Rottenberg H. The measurement of membrane potential and deltapH in cells, organelles, and vesicles. Methods Enzymol. 1979;55:547–569. doi: 10.1016/0076-6879(79)55066-6. [DOI] [PubMed] [Google Scholar]
- STRITTMATTTER C. F. Electron transport to oxygen in lactobacilli. J Biol Chem. 1959 Oct;234:2789–2793. [PubMed] [Google Scholar]
- Strobel H. J., Russell J. B., Driessen A. J., Konings W. N. Transport of amino acids in Lactobacillus casei by proton-motive-force-dependent and non-proton-motive-force-dependent mechanisms. J Bacteriol. 1989 Jan;171(1):280–284. doi: 10.1128/jb.171.1.280-284.1989. [DOI] [PMC free article] [PubMed] [Google Scholar]
- Summerfield G. P., Keenan J. P., Brodie N. J., Bellingham A. J. Bioluminescent assay of adenine nucleotides: rapid analysis of ATP and ADP in red cells and platelets using the LKB luminometer. Clin Lab Haematol. 1981;3(3):257–271. doi: 10.1111/j.1365-2257.1981.tb01340.x. [DOI] [PubMed] [Google Scholar]
- Ten Brink B., Konings W. N. Generation of an electrochemical proton gradient by lactate efflux in membrane vesicles of Escherichia coli. Eur J Biochem. 1980 Oct;111(1):59–66. doi: 10.1111/j.1432-1033.1980.tb06074.x. [DOI] [PubMed] [Google Scholar]
- Tseng C. P., Montville T. J. Enzyme Activities Affecting End Product Distribution by Lactobacillus plantarum in Response to Changes in pH and O(2). Appl Environ Microbiol. 1990 Sep;56(9):2761–2763. doi: 10.1128/aem.56.9.2761-2763.1990. [DOI] [PMC free article] [PubMed] [Google Scholar]
- Verdoni N., Aon M. A., Lebeault J. M., Thomas D. Proton motive force, energy recycling by end product excretion, and metabolic uncoupling during anaerobic growth of Pseudomonas mendocina. J Bacteriol. 1990 Dec;172(12):6673–6681. doi: 10.1128/jb.172.12.6673-6681.1990. [DOI] [PMC free article] [PubMed] [Google Scholar]
- ten Brink B., Konings W. N. Electrochemical proton gradient and lactate concentration gradient in Streptococcus cremoris cells grown in batch culture. J Bacteriol. 1982 Nov;152(2):682–686. doi: 10.1128/jb.152.2.682-686.1982. [DOI] [PMC free article] [PubMed] [Google Scholar]
- ten Brink B., Konings W. N. Generation of a protonmotive force in anaerobic bacteria by end-product efflux. Methods Enzymol. 1986;125:492–510. doi: 10.1016/s0076-6879(86)25039-9. [DOI] [PubMed] [Google Scholar]
- ten Brink B., Otto R., Hansen U. P., Konings W. N. Energy recycling by lactate efflux in growing and nongrowing cells of Streptococcus cremoris. J Bacteriol. 1985 Apr;162(1):383–390. doi: 10.1128/jb.162.1.383-390.1985. [DOI] [PMC free article] [PubMed] [Google Scholar]
- van Boven A., Konings W. N. A Phosphate-Bond-Driven Dipeptide Transport System in Streptococcus cremoris Is Regulated by the Internal pH. Appl Environ Microbiol. 1987 Dec;53(12):2897–2902. doi: 10.1128/aem.53.12.2897-2902.1987. [DOI] [PMC free article] [PubMed] [Google Scholar]