Abstract
Salmonella typhimurium can encounter a wide variety of environments during its life cycle. One component of the environment which will fluctuate widely is pH. In nature, S. typhimurium can experience and survive dramatic acid stresses that occur in diverse ecological niches ranging from pond water to phagolysosomes. However, in vitro the organism is very sensitive to acid. To provide an explanation for how this organism survives acid in natural environments, the adaptive ability of S. typhimurium to become acid tolerant was tested. Logarithmically grown cells (pH 7.6) shifted to mild acid (pH 5.8) for one doubling as an adaptive procedure were 100 to 1,000 times more resistant to subsequent strong acid challenge (pH 3.3) than were unadapted cells shifted directly from pH 7.6 to 3.3. This acidification tolerance response required protein synthesis and appears to be a specific defense mechanism for acid. No cross protection was noted for hydrogen peroxide, SOS, or heat shock. Two-dimensional polyacrylamide gel electrophoretic analysis of acid-regulated polypeptides revealed 18 proteins with altered expression, 6 of which were repressed while 12 were induced by mild acid shifts. An avirulent phoP mutant was 1,000-fold more sensitive to acid than its virulent phoP+ parent, suggesting a correlation between acid tolerance and virulence. The Mg2(+)-dependent proton-translocating ATPase was also found to play an important role in acid tolerance. Mutants (unc) lacking this activity were unable to mount an acid tolerance response and were extremely acid sensitive. In contrast to these acid-sensitive mutants, a constitutively acid-tolerant mutant (atr) was isolated from wild-type LT2 after prolonged acid exposure. This mutant overexpressed several acidification tolerance response polypeptides. The data presented reveal an important acidification defense modulon with broad significance toward survival in biologically hostile environments.
Full text
PDF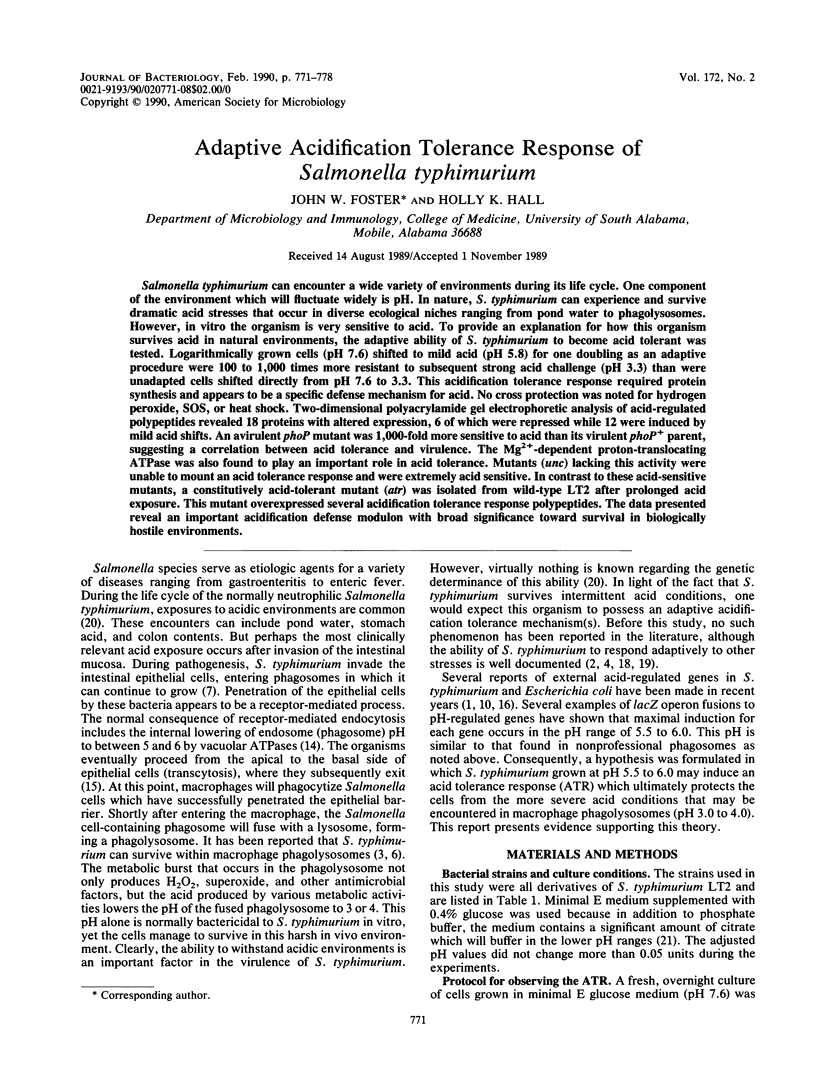
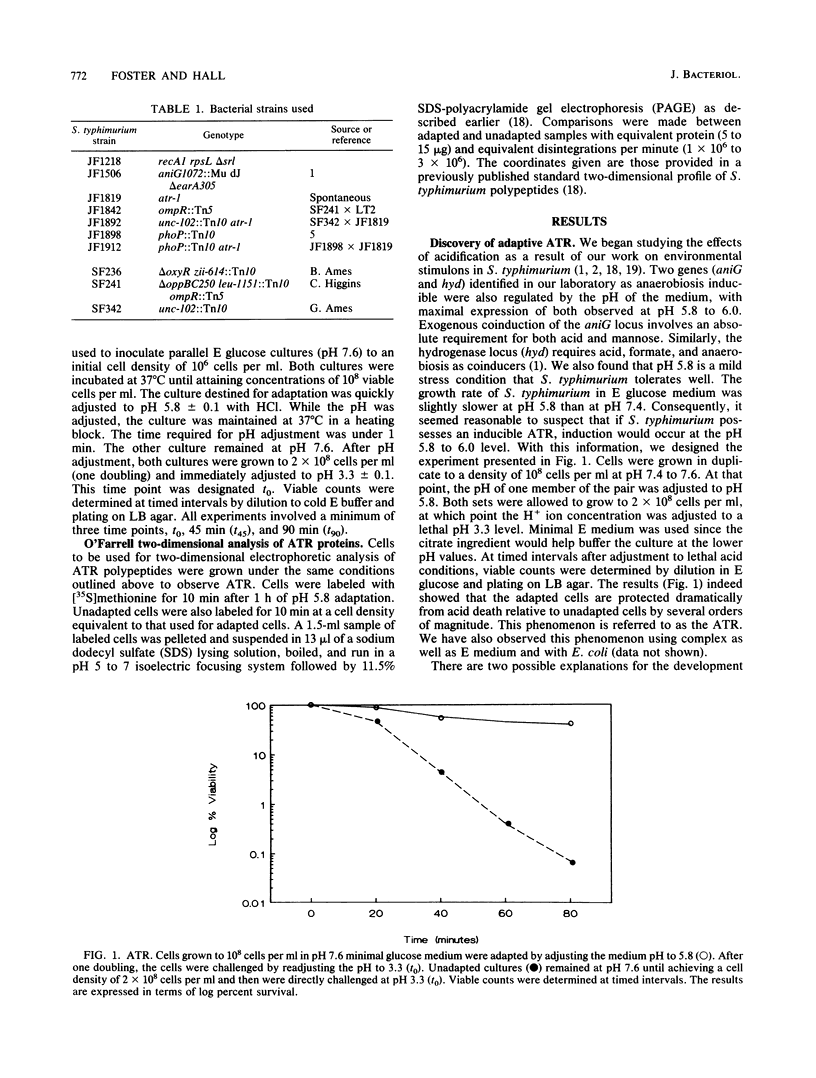
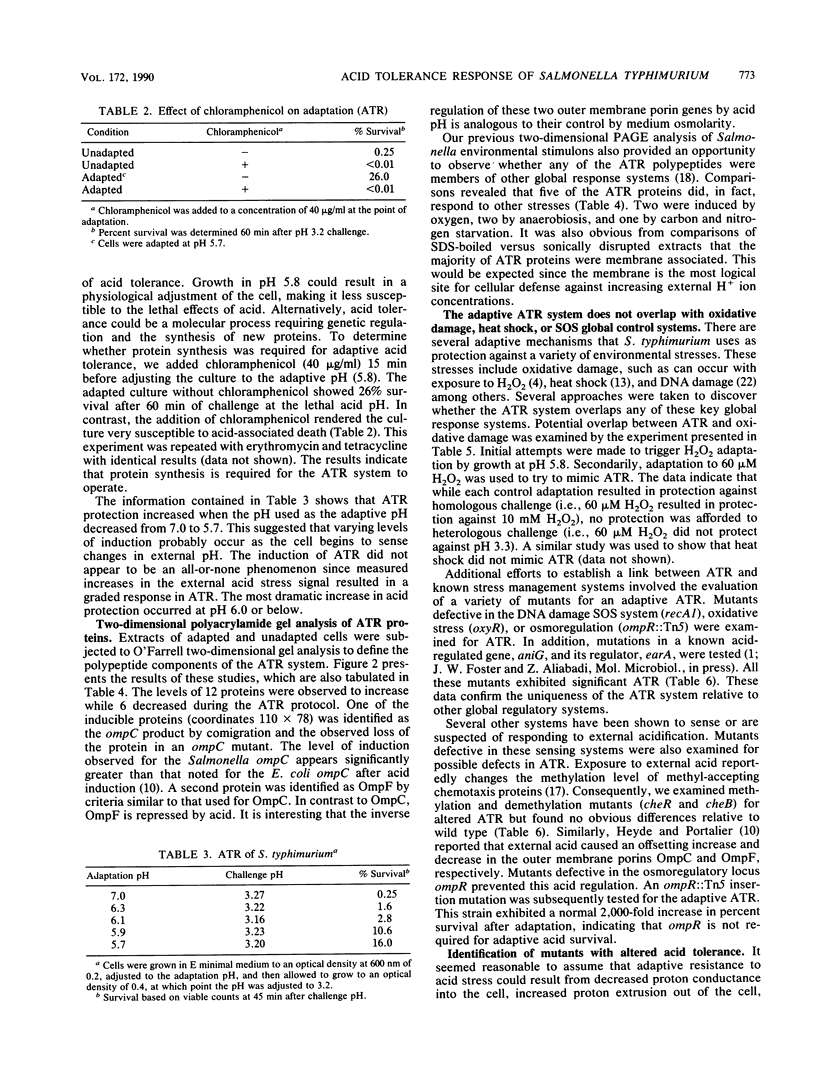
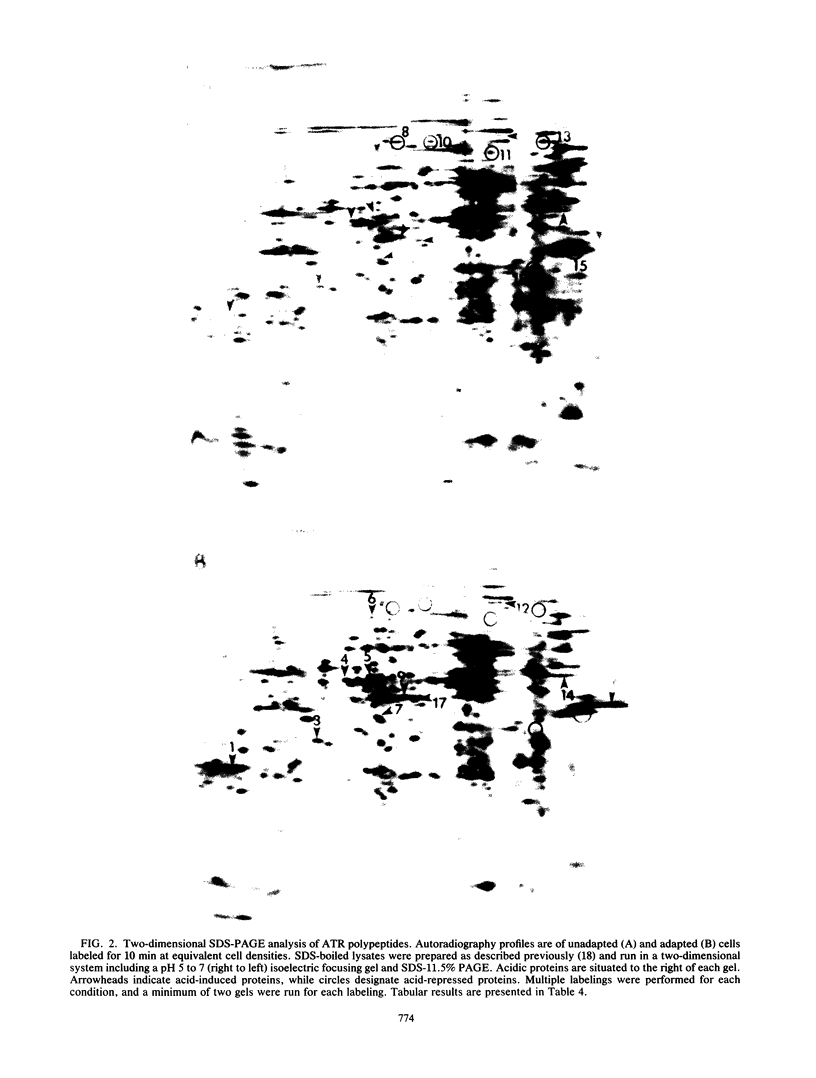
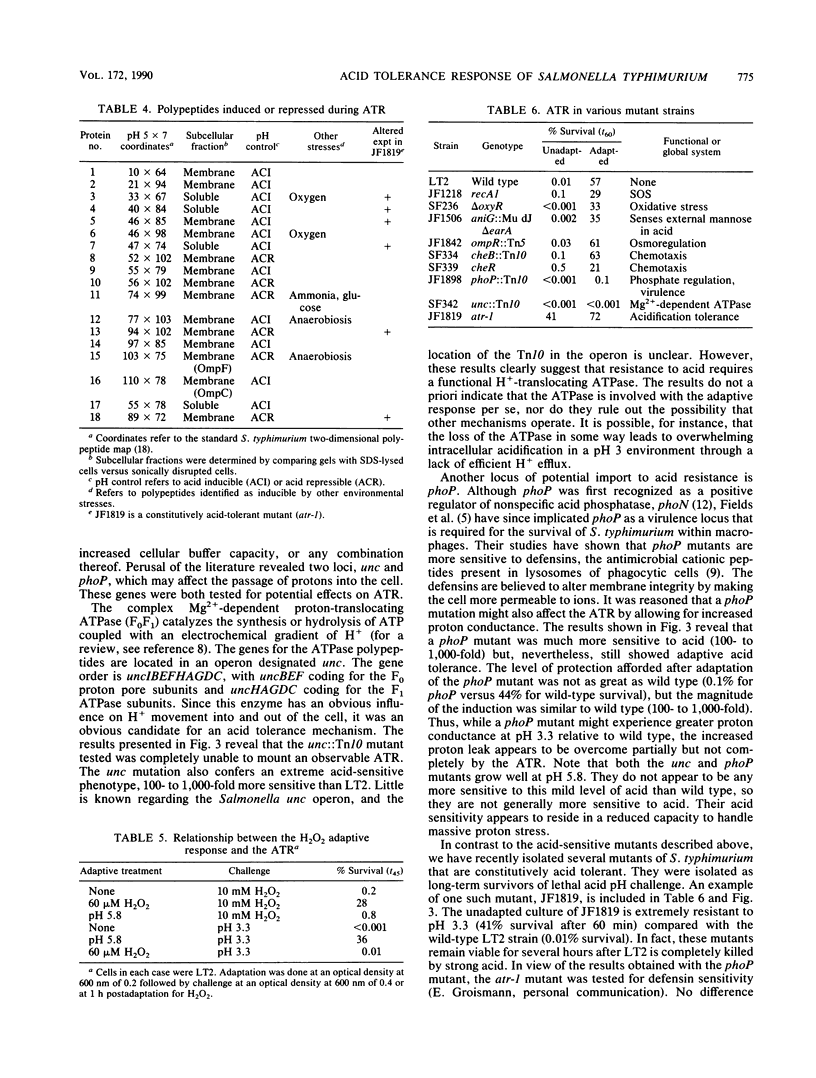
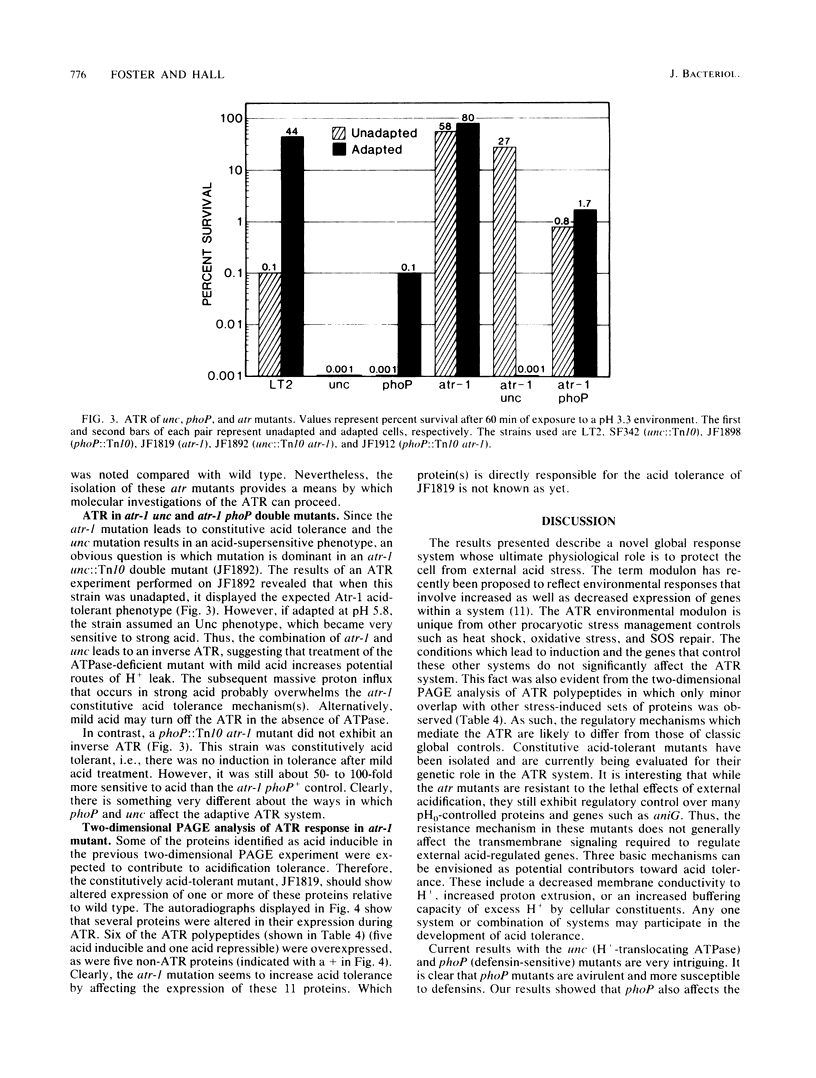
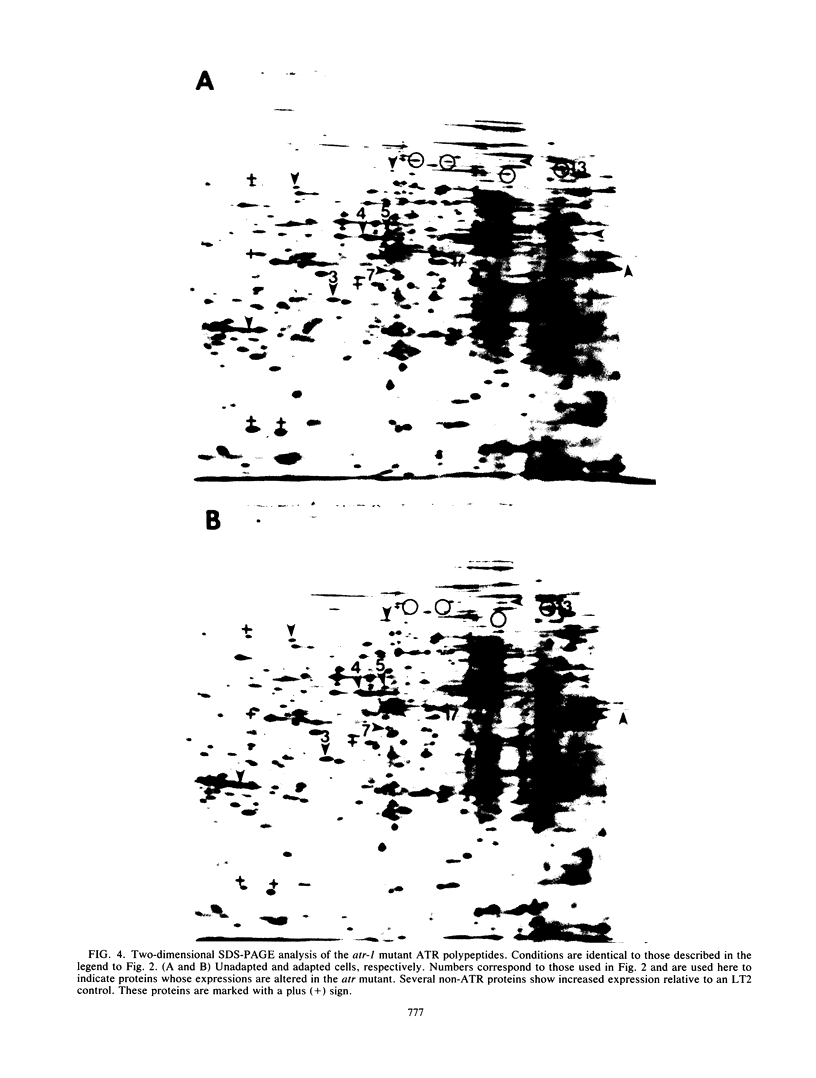
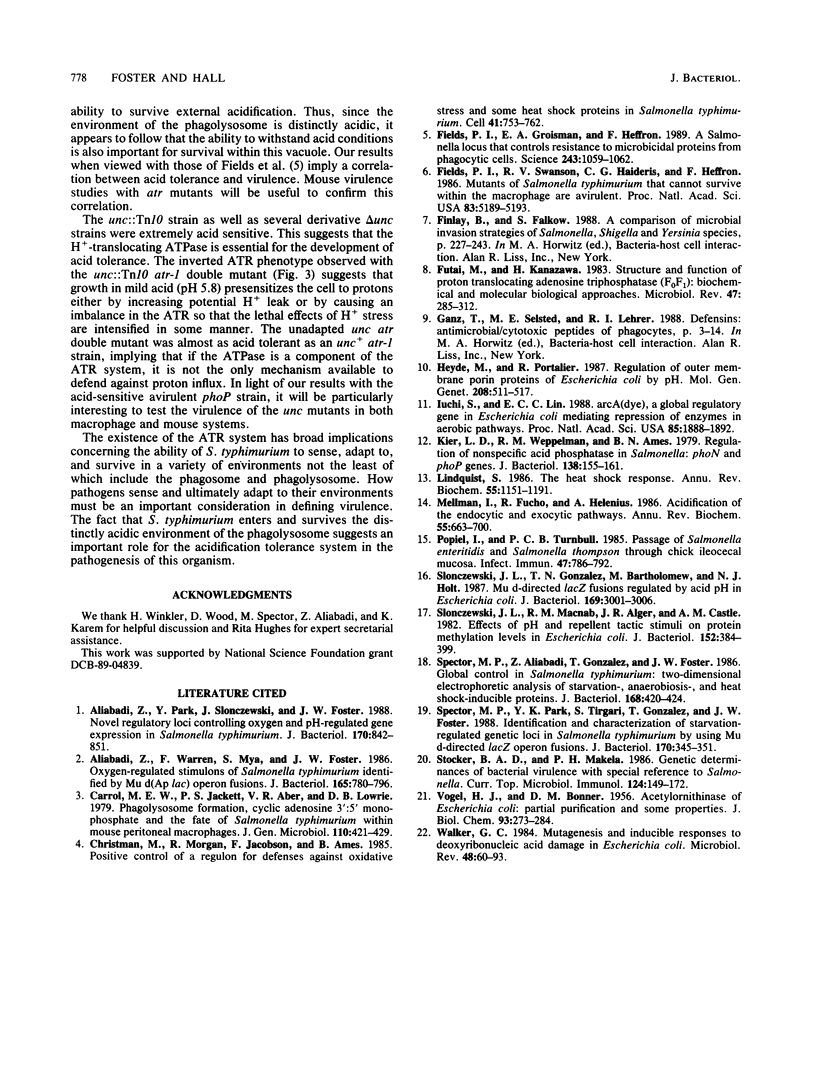
Images in this article
Selected References
These references are in PubMed. This may not be the complete list of references from this article.
- Aliabadi Z., Park Y. K., Slonczewski J. L., Foster J. W. Novel regulatory loci controlling oxygen- and pH-regulated gene expression in Salmonella typhimurium. J Bacteriol. 1988 Feb;170(2):842–851. doi: 10.1128/jb.170.2.842-851.1988. [DOI] [PMC free article] [PubMed] [Google Scholar]
- Aliabadi Z., Warren F., Mya S., Foster J. W. Oxygen-regulated stimulons of Salmonella typhimurium identified by Mu d(Ap lac) operon fusions. J Bacteriol. 1986 Mar;165(3):780–786. doi: 10.1128/jb.165.3.780-786.1986. [DOI] [PMC free article] [PubMed] [Google Scholar]
- Carrol M. E., Jackett P. S., Aber V. R., Lowrie D. B. Phagolysosome formation, cyclic adenosine 3':5'-monophosphate and the fate of Salmonella typhimurium within mouse peritoneal macrophages. J Gen Microbiol. 1979 Feb;110(2):421–429. doi: 10.1099/00221287-110-2-421. [DOI] [PubMed] [Google Scholar]
- Christman M. F., Morgan R. W., Jacobson F. S., Ames B. N. Positive control of a regulon for defenses against oxidative stress and some heat-shock proteins in Salmonella typhimurium. Cell. 1985 Jul;41(3):753–762. doi: 10.1016/s0092-8674(85)80056-8. [DOI] [PubMed] [Google Scholar]
- Fields P. I., Groisman E. A., Heffron F. A Salmonella locus that controls resistance to microbicidal proteins from phagocytic cells. Science. 1989 Feb 24;243(4894 Pt 1):1059–1062. doi: 10.1126/science.2646710. [DOI] [PubMed] [Google Scholar]
- Fields P. I., Swanson R. V., Haidaris C. G., Heffron F. Mutants of Salmonella typhimurium that cannot survive within the macrophage are avirulent. Proc Natl Acad Sci U S A. 1986 Jul;83(14):5189–5193. doi: 10.1073/pnas.83.14.5189. [DOI] [PMC free article] [PubMed] [Google Scholar]
- Futai M., Kanazawa H. Structure and function of proton-translocating adenosine triphosphatase (F0F1): biochemical and molecular biological approaches. Microbiol Rev. 1983 Sep;47(3):285–312. doi: 10.1128/mr.47.3.285-312.1983. [DOI] [PMC free article] [PubMed] [Google Scholar]
- Heyde M., Portalier R. Regulation of major outer membrane porin proteins of Escherichia coli K 12 by pH. Mol Gen Genet. 1987 Jul;208(3):511–517. doi: 10.1007/BF00328148. [DOI] [PubMed] [Google Scholar]
- Iuchi S., Lin E. C. arcA (dye), a global regulatory gene in Escherichia coli mediating repression of enzymes in aerobic pathways. Proc Natl Acad Sci U S A. 1988 Mar;85(6):1888–1892. doi: 10.1073/pnas.85.6.1888. [DOI] [PMC free article] [PubMed] [Google Scholar]
- Kier L. D., Weppelman R. M., Ames B. N. Regulation of nonspecific acid phosphatase in Salmonella: phoN and phoP genes. J Bacteriol. 1979 Apr;138(1):155–161. doi: 10.1128/jb.138.1.155-161.1979. [DOI] [PMC free article] [PubMed] [Google Scholar]
- Lindquist S. The heat-shock response. Annu Rev Biochem. 1986;55:1151–1191. doi: 10.1146/annurev.bi.55.070186.005443. [DOI] [PubMed] [Google Scholar]
- Mellman I., Fuchs R., Helenius A. Acidification of the endocytic and exocytic pathways. Annu Rev Biochem. 1986;55:663–700. doi: 10.1146/annurev.bi.55.070186.003311. [DOI] [PubMed] [Google Scholar]
- Popiel I., Turnbull P. C. Passage of Salmonella enteritidis and Salmonella thompson through chick ileocecal mucosa. Infect Immun. 1985 Mar;47(3):786–792. doi: 10.1128/iai.47.3.786-792.1985. [DOI] [PMC free article] [PubMed] [Google Scholar]
- Slonczewski J. L., Gonzalez T. N., Bartholomew F. M., Holt N. J. Mu d-directed lacZ fusions regulated by low pH in Escherichia coli. J Bacteriol. 1987 Jul;169(7):3001–3006. doi: 10.1128/jb.169.7.3001-3006.1987. [DOI] [PMC free article] [PubMed] [Google Scholar]
- Slonczewski J. L., Macnab R. M., Alger J. R., Castle A. M. Effects of pH and repellent tactic stimuli on protein methylation levels in Escherichia coli. J Bacteriol. 1982 Oct;152(1):384–399. doi: 10.1128/jb.152.1.384-399.1982. [DOI] [PMC free article] [PubMed] [Google Scholar]
- Spector M. P., Aliabadi Z., Gonzalez T., Foster J. W. Global control in Salmonella typhimurium: two-dimensional electrophoretic analysis of starvation-, anaerobiosis-, and heat shock-inducible proteins. J Bacteriol. 1986 Oct;168(1):420–424. doi: 10.1128/jb.168.1.420-424.1986. [DOI] [PMC free article] [PubMed] [Google Scholar]
- Spector M. P., Park Y. K., Tirgari S., Gonzalez T., Foster J. W. Identification and characterization of starvation-regulated genetic loci in Salmonella typhimurium by using Mu d-directed lacZ operon fusions. J Bacteriol. 1988 Jan;170(1):345–351. doi: 10.1128/jb.170.1.345-351.1988. [DOI] [PMC free article] [PubMed] [Google Scholar]
- Stocker B. A., Mäkelä P. H. Genetic determination of bacterial virulence, with special reference to Salmonella. Curr Top Microbiol Immunol. 1986;124:149–172. doi: 10.1007/978-3-642-70986-9_9. [DOI] [PubMed] [Google Scholar]
- Walker G. C. Mutagenesis and inducible responses to deoxyribonucleic acid damage in Escherichia coli. Microbiol Rev. 1984 Mar;48(1):60–93. doi: 10.1128/mr.48.1.60-93.1984. [DOI] [PMC free article] [PubMed] [Google Scholar]