Abstract
The heteropolysaccharide chains of enterobacterial common antigen (ECA) are made up of linear trisaccharide repeat units with the structure----3)-alpha-D-Fuc4NAc-(1----4)- beta-D-ManNAcA-(1----4)-alpha-D-GlcNAc-(1----, where Fuc4NAc is 4-acetamido-4,6-dideoxy-D-galactose, ManNAcA is N-acetyl-D-mannosaminuronic acid, and GlcNAc is N-acetyl-D-glucosamine. The assembly of these chains involves lipid-linked intermediates, and both GlcNAc-pyrophosphorylundecaprenol (lipid I) and ManNAcA-GlcNAc-pyrophosphorylundecaprenol (lipid II) are intermediates in ECA biosynthesis. In this study we demonstrated that lipid II serves as the acceptor of Fuc4NAc residues in the assembly of the trisaccharide repeat unit of ECA chains. Incubation of Escherichia coli membranes with UDP-GlcNAc, UDP-[14C]ManNAcA, and TDP-[3H]Fuc4NAc resulted in the synthesis of a radioactive glycolipid (lipid III) that contained both [14C]ManNAcA and [3H]Fuc4NAc. The oligosaccharide moiety of lipid III was identified as a trisaccharide by gel-permeation chromatography, and the in vitro synthesis of lipid III was dependent on prior synthesis of lipids I and II. Accordingly, the incorporation of [3H]Fuc4NAc into lipid III from the donor TDP-[3H]Fuc4NAc was dependent on the presence of both UDP-GlcNAc and UDP-ManNAcA in the reaction mixtures. In addition, the in vitro synthesis of lipid III was abolished by tunicamycin. Direct conversion of lipid II to lipid III was demonstrated in two-stage reactions in which membranes were initially incubated with UDP-GlcNAc and UDP-[14C]ManNAcA to allow the synthesis of radioactive lipid II. Subsequent addition of TDP-Fuc4Nac to the washed membranes resulted in almost complete conversion of radioactive lipid II to lipid III. The in vitro synthesis of lipid III was also accompanied by the apparent utilization of this lipid intermediate for the assembly of ECA heteropolysaccharide chains. Incubation of membranes with UDP-[3H]GlcNAc, UDP-ManNAcA, and TDP-Fuc4NAc resulted in the apparent incorporation of isotope into ECA polymers, as determined by sodium dodecyl sulfate-polyacrylamide gel electrophoresis and fluorography. In addition, the in vitro incorporation of [3H]Fuc4NAc into ECA heteropolysaccharide chains was demonstrated with ether-treated cells that were prepared from delta rfbA mutants of Salmonella typhimurium. These mutants are defective in the synthesis of TDP-Fuc4NAc; as a consequence, they are also defective in the synthesis of lipid III and they accumulate lipid II. Accordingly, incubation of ether-permeabilized cells of delta rfbA mutants with TDP-[3h]Fuc4NAc resulted in the incorporation of isotope into both lipid III and ECA heteropolysaccharide chains.
Full text
PDF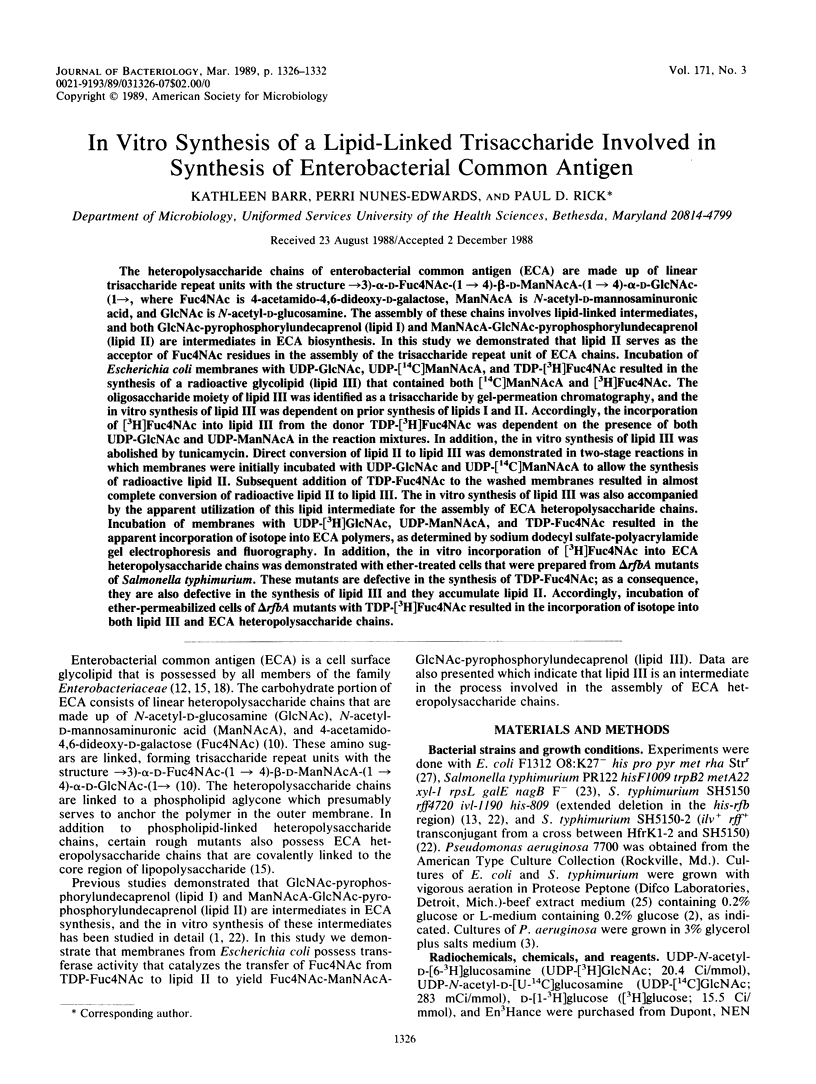
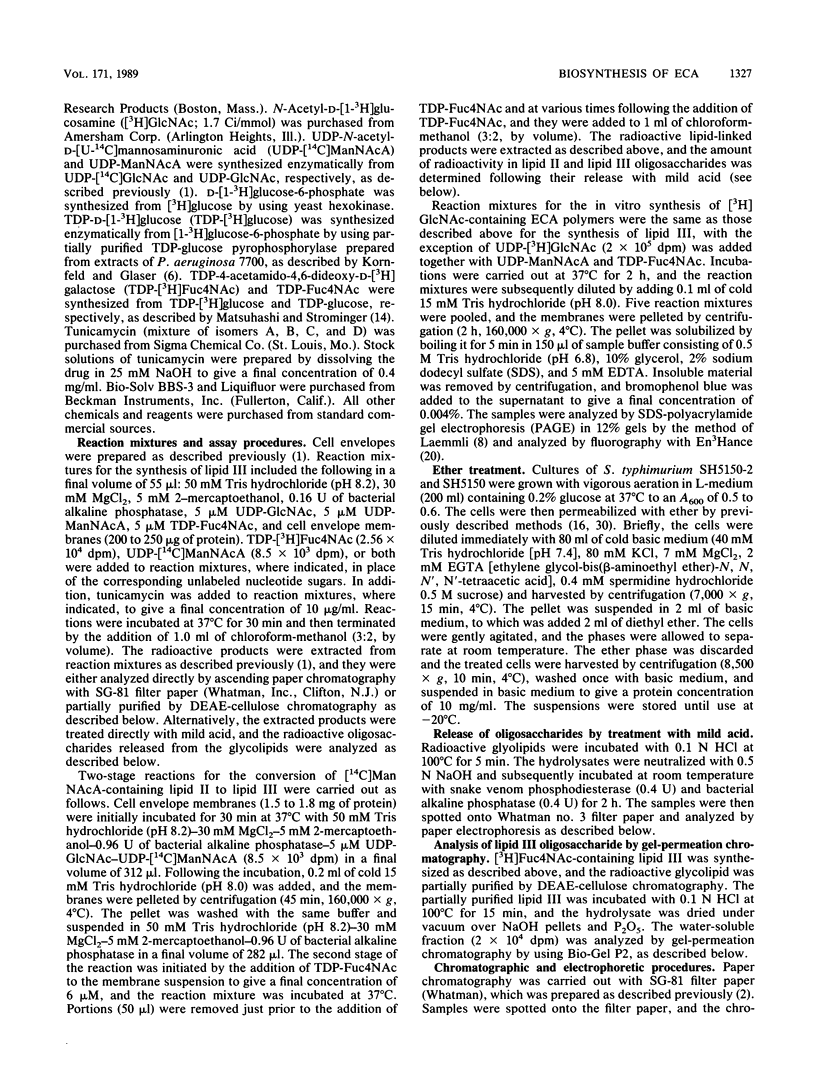
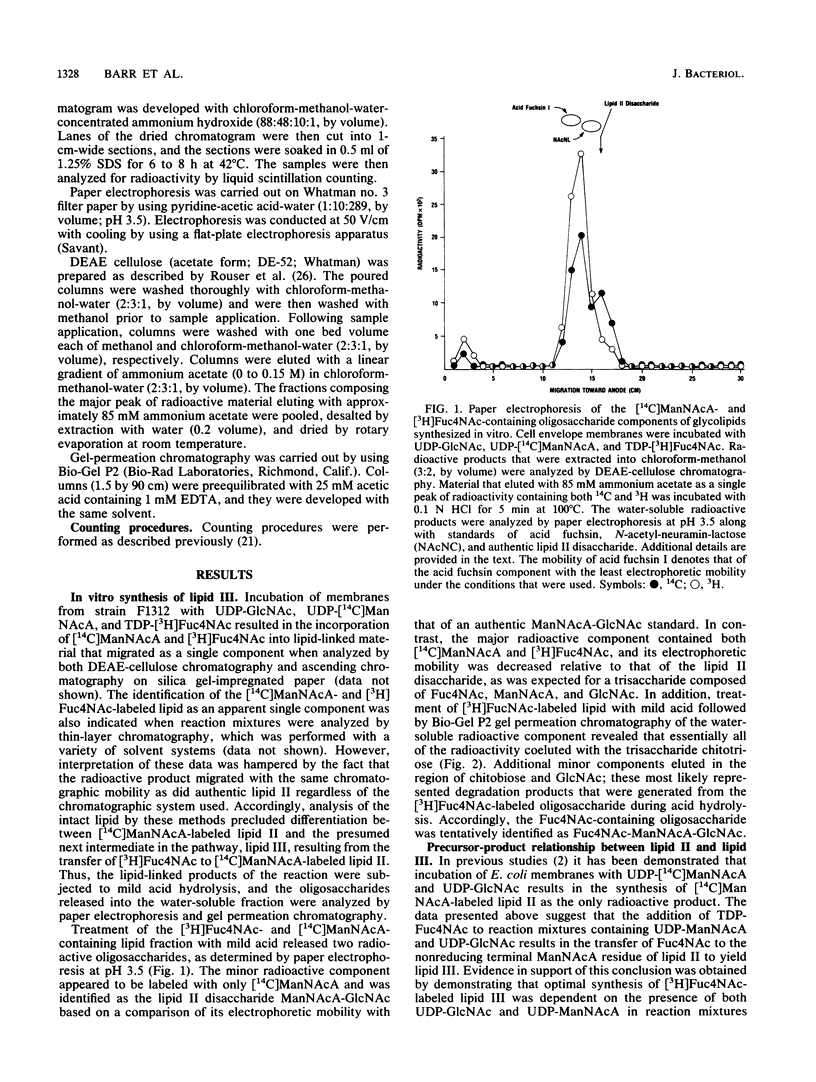
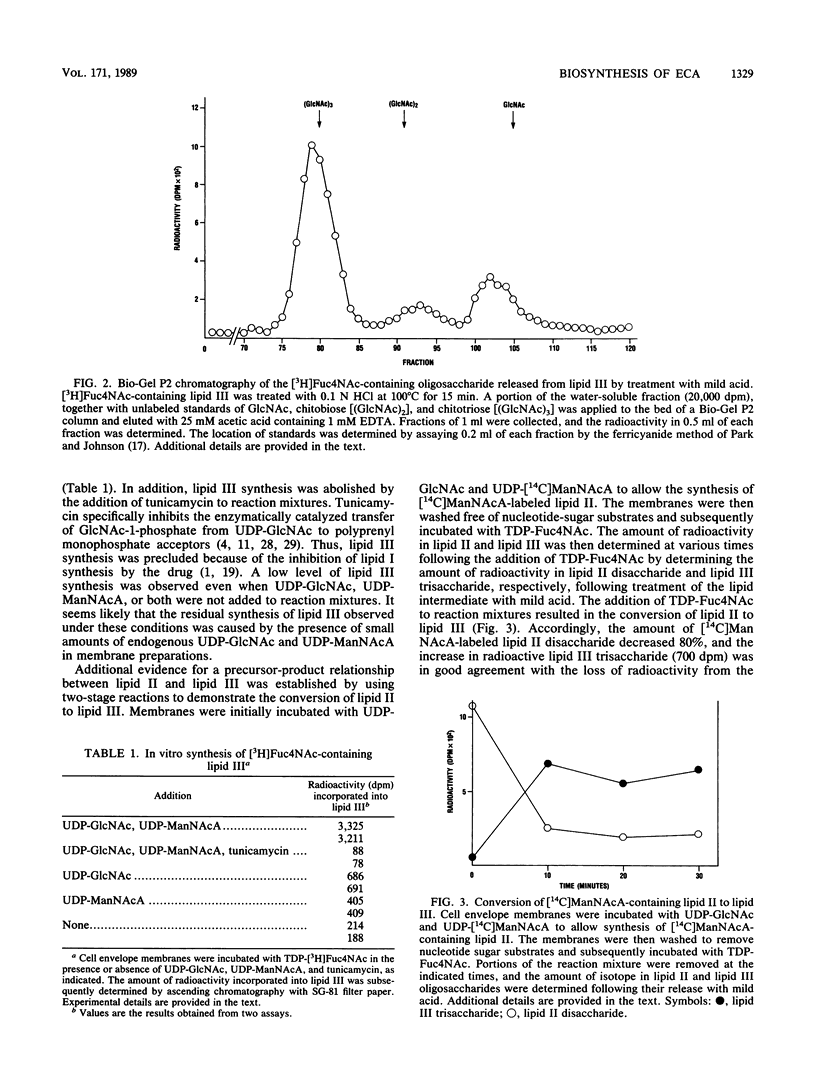
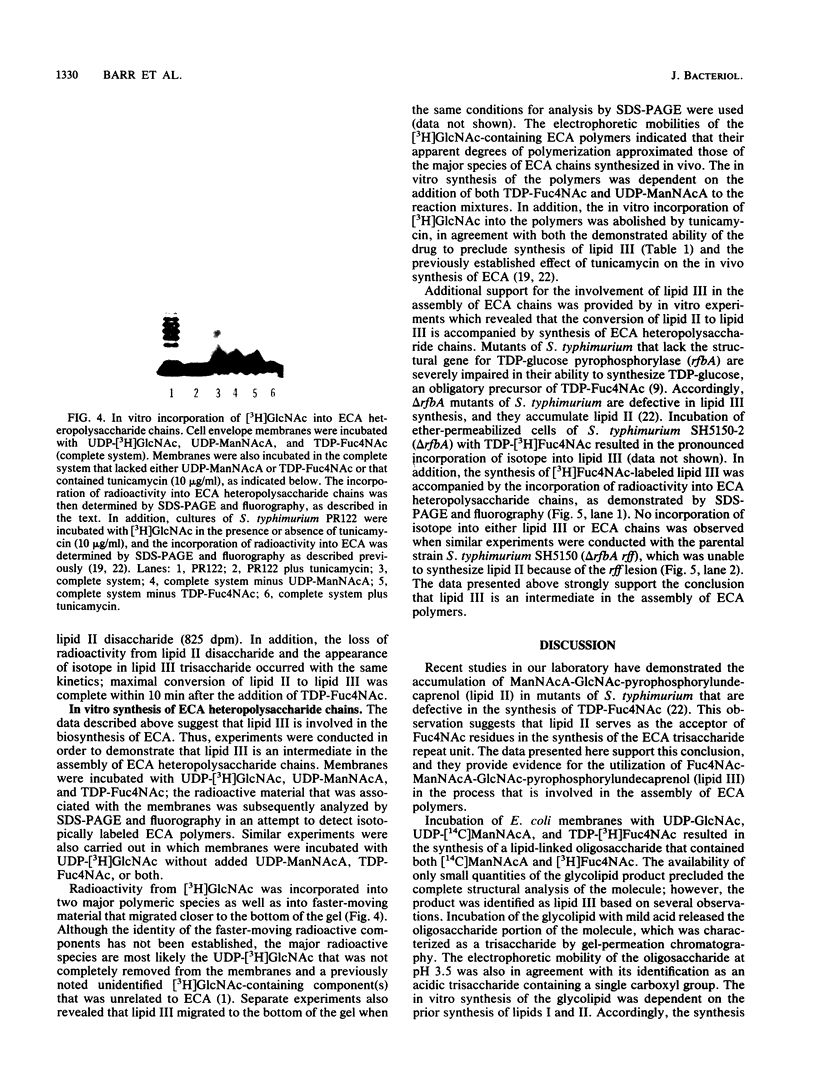
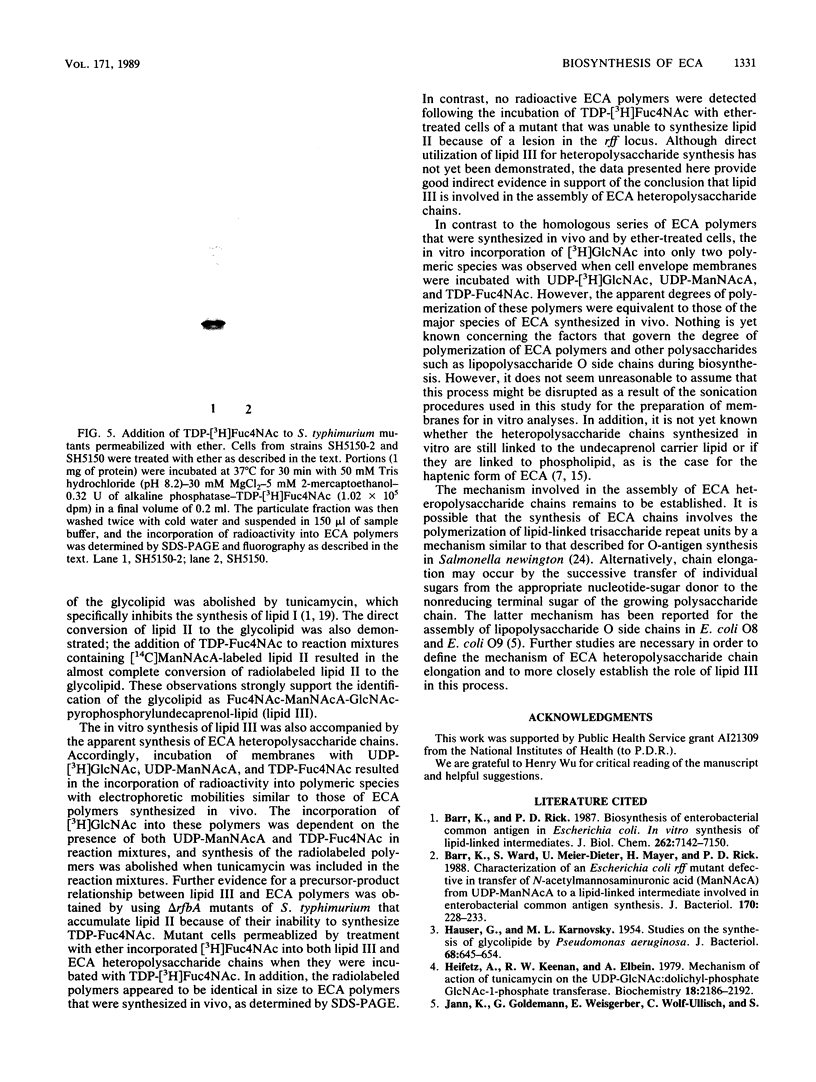
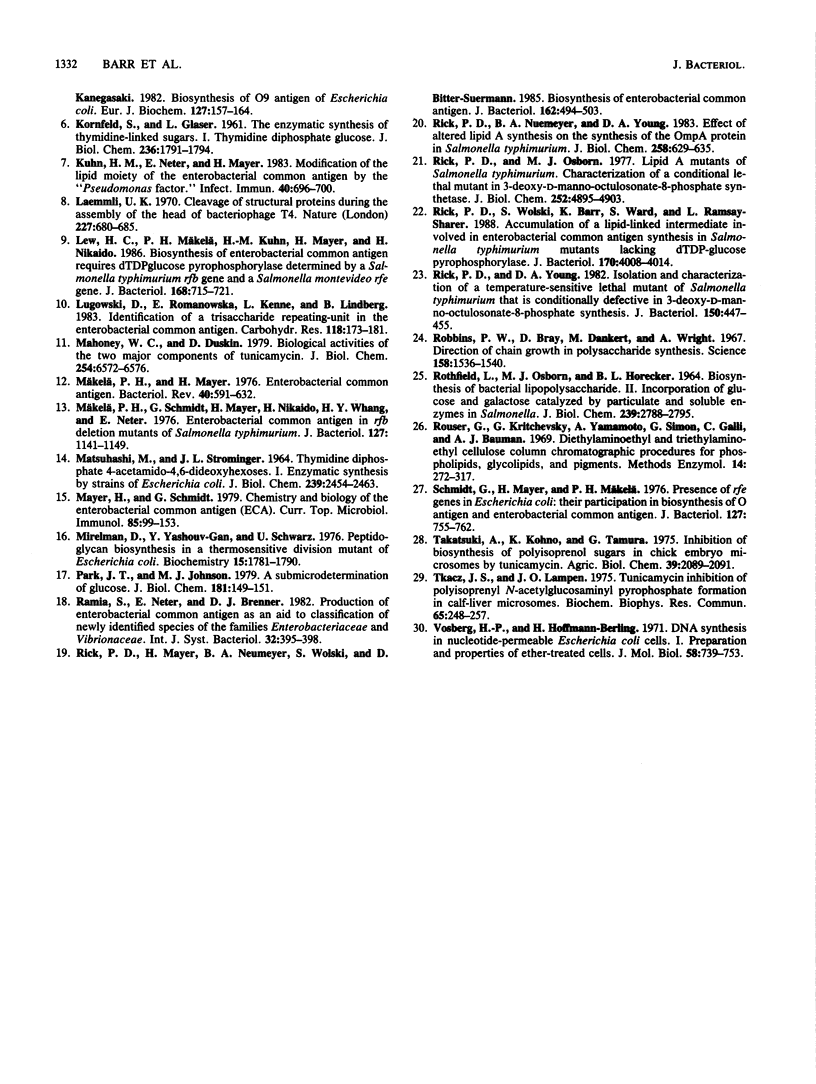
Images in this article
Selected References
These references are in PubMed. This may not be the complete list of references from this article.
- Barr K., Rick P. D. Biosynthesis of enterobacterial common antigen in Escherichia coli. In vitro synthesis of lipid-linked intermediates. J Biol Chem. 1987 May 25;262(15):7142–7150. [PubMed] [Google Scholar]
- Barr K., Ward S., Meier-Dieter U., Mayer H., Rick P. D. Characterization of an Escherichia coli rff mutant defective in transfer of N-acetylmannosaminuronic acid (ManNAcA) from UDP-ManNAcA to a lipid-linked intermediate involved in enterobacterial common antigen synthesis. J Bacteriol. 1988 Jan;170(1):228–233. doi: 10.1128/jb.170.1.228-233.1988. [DOI] [PMC free article] [PubMed] [Google Scholar]
- HAUSER G., KARNOVSKY M. L. Studies on the production of glycolipide by Pseudomonas aeruginosa. J Bacteriol. 1954 Dec;68(6):645–654. doi: 10.1128/jb.68.6.645-654.1954. [DOI] [PMC free article] [PubMed] [Google Scholar]
- Heifetz A., Keenan R. W., Elbein A. D. Mechanism of action of tunicamycin on the UDP-GlcNAc:dolichyl-phosphate Glc-NAc-1-phosphate transferase. Biochemistry. 1979 May 29;18(11):2186–2192. doi: 10.1021/bi00578a008. [DOI] [PubMed] [Google Scholar]
- Jann K., Goldemann G., Weisgerber C., Wolf-Ullisch C., Kanegasaki S. Biosynthesis of the O9 antigen of Escherichia coli. Initial reaction and overall mechanism. Eur J Biochem. 1982 Sep;127(1):157–164. doi: 10.1111/j.1432-1033.1982.tb06850.x. [DOI] [PubMed] [Google Scholar]
- KORNFELD S., GLASER L. The enzymic synthesis of thymidine-linked sugars. I. Thymidine diphosphate glucose. J Biol Chem. 1961 Jun;236:1791–1794. [PubMed] [Google Scholar]
- Kuhn H. M., Neter E., Mayer H. Modification of the lipid moiety of the enterobacterial common antigen by the "Pseudomonas factor". Infect Immun. 1983 May;40(2):696–700. doi: 10.1128/iai.40.2.696-700.1983. [DOI] [PMC free article] [PubMed] [Google Scholar]
- Laemmli U. K. Cleavage of structural proteins during the assembly of the head of bacteriophage T4. Nature. 1970 Aug 15;227(5259):680–685. doi: 10.1038/227680a0. [DOI] [PubMed] [Google Scholar]
- Lew H. C., Mäkelä P. H., Kuhn H. M., Mayer H., Nikaido H. Biosynthesis of enterobacterial common antigen requires dTDPglucose pyrophosphorylase determined by a Salmonella typhimurium rfb gene and a Salmonella montevideo rfe gene. J Bacteriol. 1986 Nov;168(2):715–721. doi: 10.1128/jb.168.2.715-721.1986. [DOI] [PMC free article] [PubMed] [Google Scholar]
- MATSUHASHI M., STROMINGER J. L. THYMIDINE DIPHOSPHATE 4-ACETAMIDO-4, 6-DIDEOXYHEXOSES. I. ENZYMATIC SYNTHESIS BY STRAINS OF ESCHERICHIA COLI. J Biol Chem. 1964 Aug;239:2454–2463. [PubMed] [Google Scholar]
- Mahoney W. C., Duksin D. Biological activities of the two major components of tunicamycin. J Biol Chem. 1979 Jul 25;254(14):6572–6576. [PubMed] [Google Scholar]
- Mayer H., Schmidt G. Chemistry and biology of the enterobacterial common antigen (ECA). Curr Top Microbiol Immunol. 1979;85:99–153. doi: 10.1007/978-3-642-67322-1_3. [DOI] [PubMed] [Google Scholar]
- Mirelman D., Yashouv-Gan Y., Schwarz U. Peptidoglycan biosynthesis in a thermosensitive division mutant of Escherichia coli. Biochemistry. 1976 May 4;15(9):1781–1790. doi: 10.1021/bi00654a001. [DOI] [PubMed] [Google Scholar]
- Mäkelä P. H., Mayer H. Enterobacterial common antigen. Bacteriol Rev. 1976 Sep;40(3):591–632. doi: 10.1128/br.40.3.591-632.1976. [DOI] [PMC free article] [PubMed] [Google Scholar]
- Mäkelä P. H., Schmidt G., Mayer H., Nikaido H., Whang H. Y., Neter E. Enterobacterial common antigen in rfb deletion mutants of Salmonella typhimurium. J Bacteriol. 1976 Sep;127(3):1141–1149. doi: 10.1128/jb.127.3.1141-1149.1976. [DOI] [PMC free article] [PubMed] [Google Scholar]
- PARK J. T., JOHNSON M. J. A submicrodetermination of glucose. J Biol Chem. 1949 Nov;181(1):149–151. [PubMed] [Google Scholar]
- ROTHFIELD L., OSBORN M. J., HORECKER B. L. BIOSYNTHESIS OF BACTERIAL LIPOPOLYSACCHARIDE. II. INCORPORATION OF GLUCOSE AND GALACTOSE CATALYZED BY PARTICULATE AND SOLUBLE ENZYMES IN SALMONELLA. J Biol Chem. 1964 Sep;239:2788–2795. [PubMed] [Google Scholar]
- Rick P. D., Mayer H., Neumeyer B. A., Wolski S., Bitter-Suermann D. Biosynthesis of enterobacterial common antigen. J Bacteriol. 1985 May;162(2):494–503. doi: 10.1128/jb.162.2.494-503.1985. [DOI] [PMC free article] [PubMed] [Google Scholar]
- Rick P. D., Osborn M. J. Lipid A mutants of Salmonella typhimurium. Characterization of a conditional lethal mutant in 3-deoxy-D-mannooctulosonate-8-phosphate synthetase. J Biol Chem. 1977 Jul 25;252(14):4895–4903. [PubMed] [Google Scholar]
- Rick P. D., Young D. A. Isolation and characterization of a temperature-sensitive lethal mutant of Salmonella typhimurium that is conditionally defective in 3-deoxy-D-manno-octulosonate-8-phosphate synthesis. J Bacteriol. 1982 May;150(2):447–455. doi: 10.1128/jb.150.2.447-455.1982. [DOI] [PMC free article] [PubMed] [Google Scholar]
- Robbins P. W., Bray D., Dankert B. M., Wright A. Direction of chain growth in polysaccharide synthesis. Science. 1967 Dec 22;158(3808):1536–1542. doi: 10.1126/science.158.3808.1536. [DOI] [PubMed] [Google Scholar]
- Schmidt G., Mayer H., Mäkelä P. H. Presence of rfe genes in Escherichia coli: their participation in biosynthesis of O antigen and enterobacterial common antigen. J Bacteriol. 1976 Aug;127(2):755–762. doi: 10.1128/jb.127.2.755-762.1976. [DOI] [PMC free article] [PubMed] [Google Scholar]
- Tkacz J. S., Lampen O. Tunicamycin inhibition of polyisoprenyl N-acetylglucosaminyl pyrophosphate formation in calf-liver microsomes. Biochem Biophys Res Commun. 1975 Jul 8;65(1):248–257. doi: 10.1016/s0006-291x(75)80086-6. [DOI] [PubMed] [Google Scholar]
- Vosberg H. P., Hoffmann-Berling H. DNA synthesis in nucleotide-permeable Escherichia coli cells. I. Preparation and properties of ether-treated cells. J Mol Biol. 1971 Jun 28;58(3):739–753. doi: 10.1016/0022-2836(71)90037-4. [DOI] [PubMed] [Google Scholar]