Abstract
Streptococcus cremoris cells that had been grown in a chemostat were starved for lactose. The viability of the culture remained essentially constant in the first hours of starvation and subsequently declined logarithmically. The viability pattern during starvation varied with the previously imposed growth rates. The death rates were 0.029, 0.076, and 0.298 h-1 for cells grown at dilution rates of 0.07, 0.11 and 0.38 h-1, respectively. The proton motive force and the pools of energy-rich phosphorylated intermediates in cells grown at a dilution rate of 0.10 h-1 fell to zero within 2 h of starvation. The culture, however, remained fully viable for at least 20 h, indicating that these energy-rich intermediates are not crucial for survival during long-term lactose starvation. Upon starvation, the intracellular pools of several amino acids depleted with the proton motive force, while large concentration gradients of the amino acids alanine, glycine, aspartate, and glutamate were retained for several hours. A quantitative analysis of the amino acids released indicated that nonspecific protein degradation was not a major cause of the loss in viability. The response of the energy metabolism of starved S. cremoris cells upon refeeding with lactose was monitored. Upon lactose starvation, the glycolytic activity and the rate of proton motive force generation decreased rapidly but the steady-state level of the proton motive force decreased significantly only after several hours. The decreasing steady-state level of the proton motive force and consequently the capacity to accumulate amino acids after the addition of lactose correlated well with the loss of viability. The response of the energy metabolism of starved S. cremoris cells upon refeeding with lactose was monitored. Upon lactose starvation, the glycolytic activity and the rate of proton motive force generation decreased rapidly but the steady-state level of the proton motive force decreased significantly only after several hours. The decreasing steady-state level of the proton motive force and consequently the capacity to accumulate amino acids after the addition of lactose correlated well with the loss of viability. It is concluded that a regulatory loss of glycolytic capacity has pivotal role in the survival of S. cremoris under the conditions used.
Full text
PDF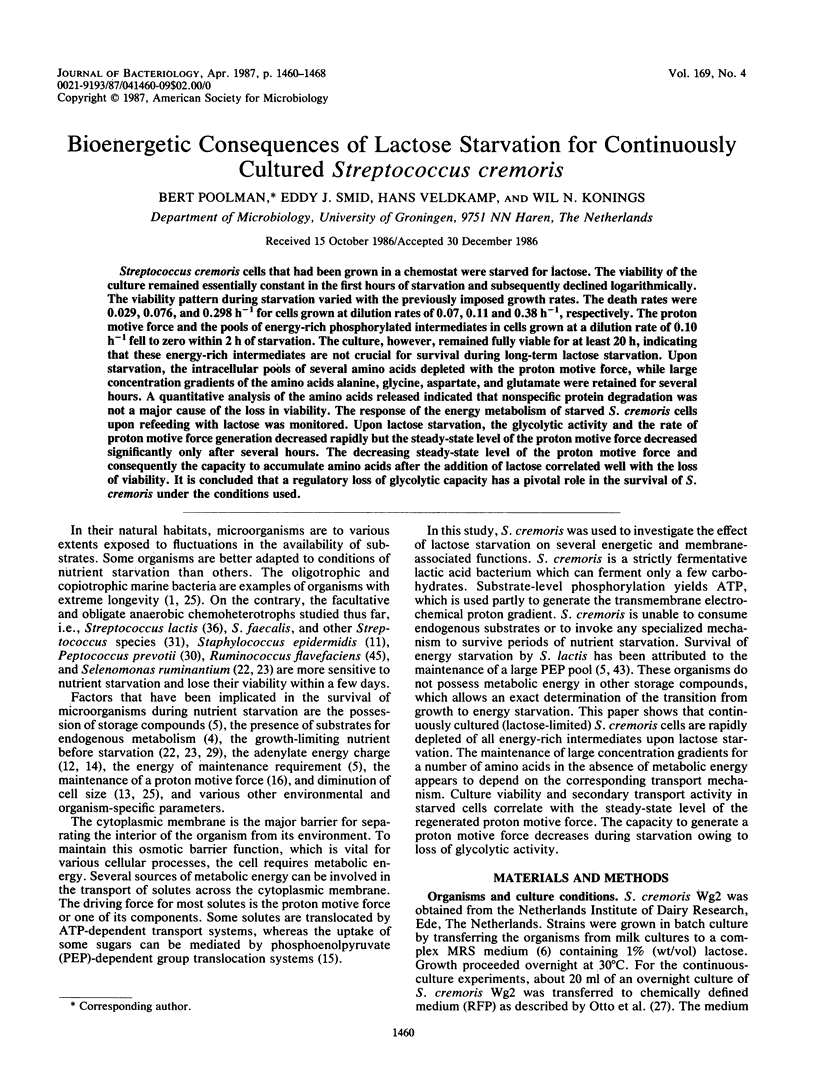
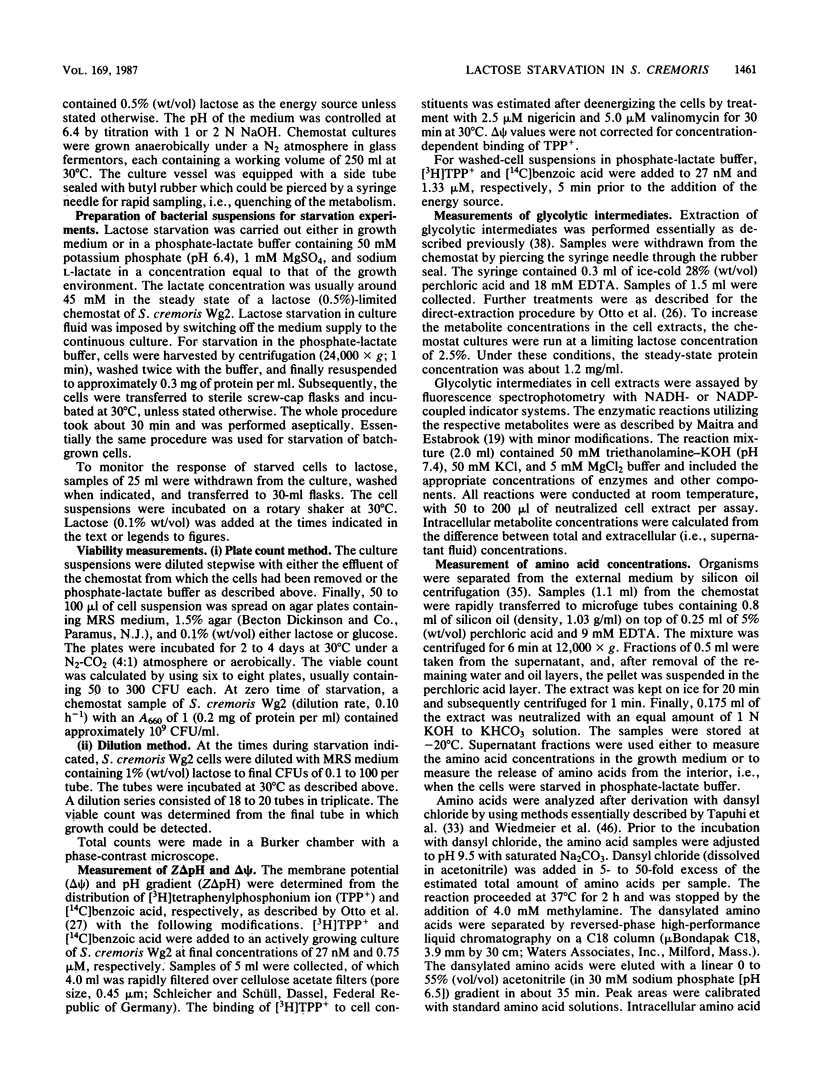
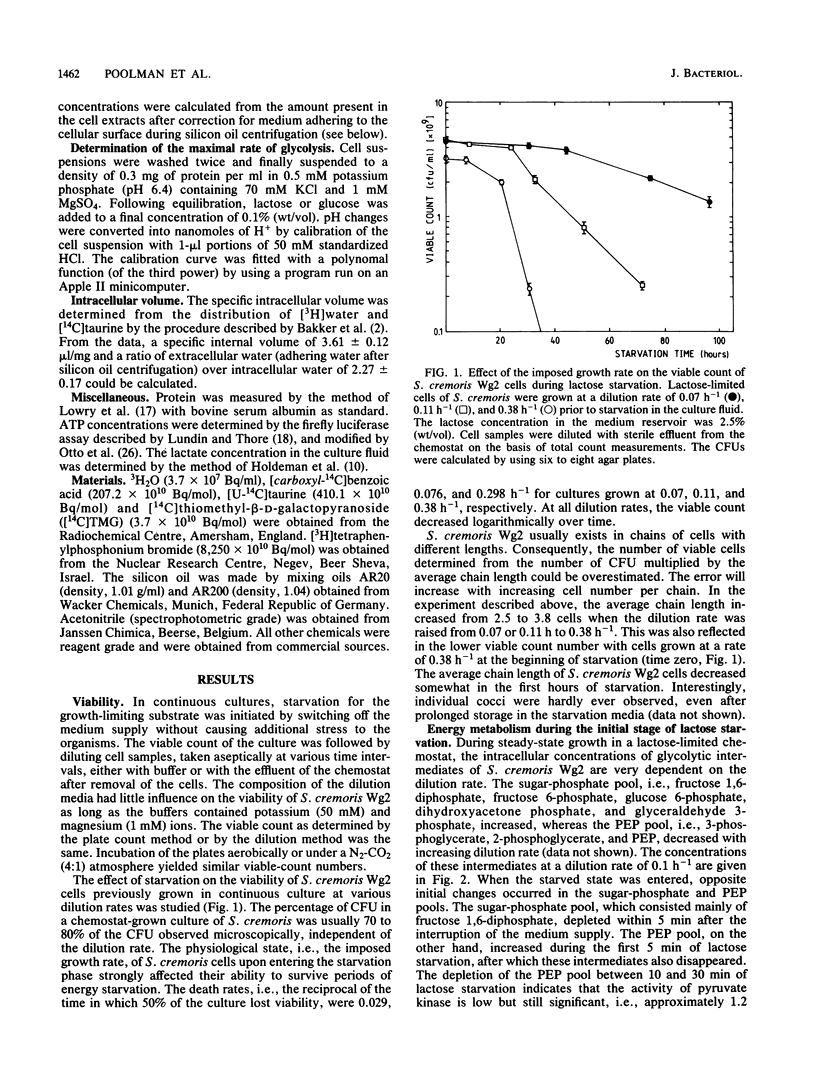
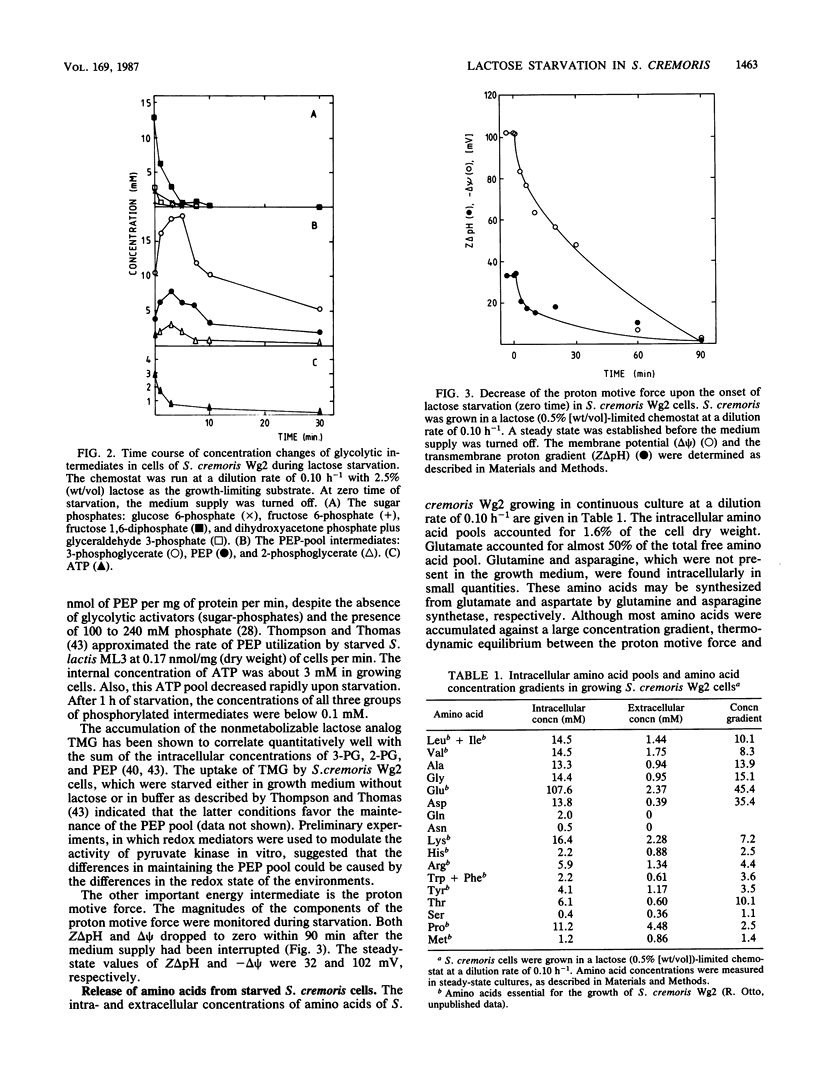
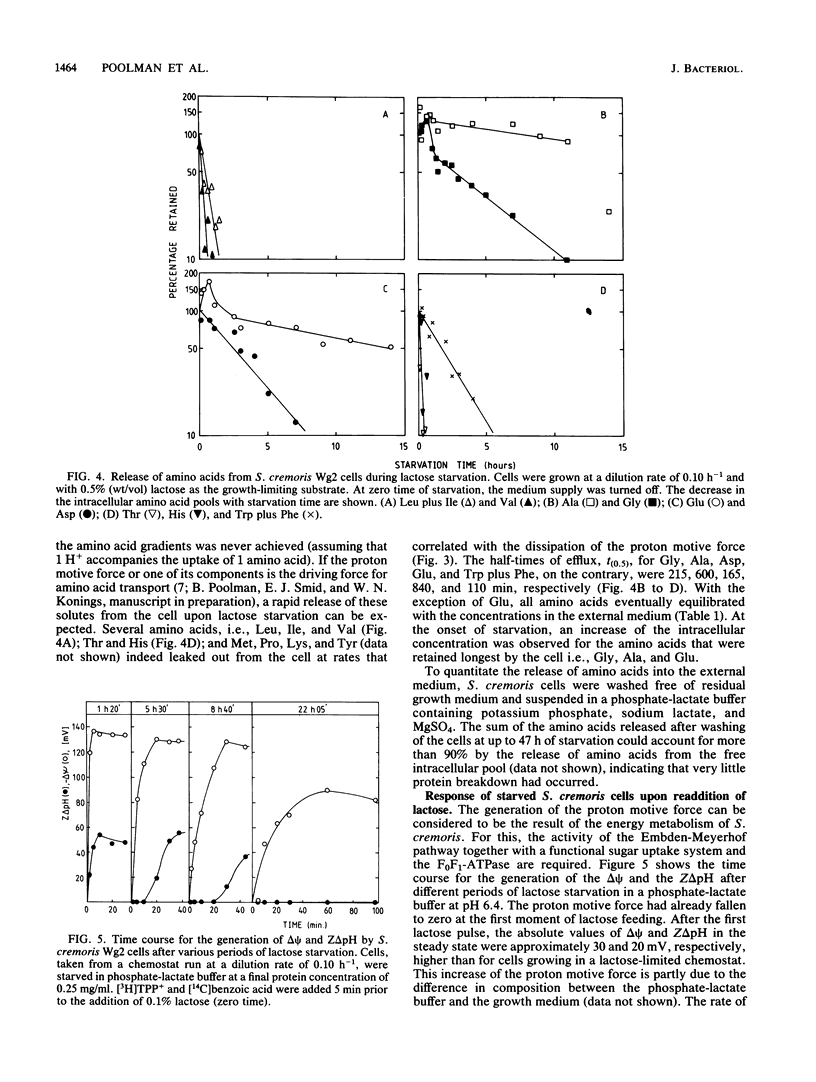
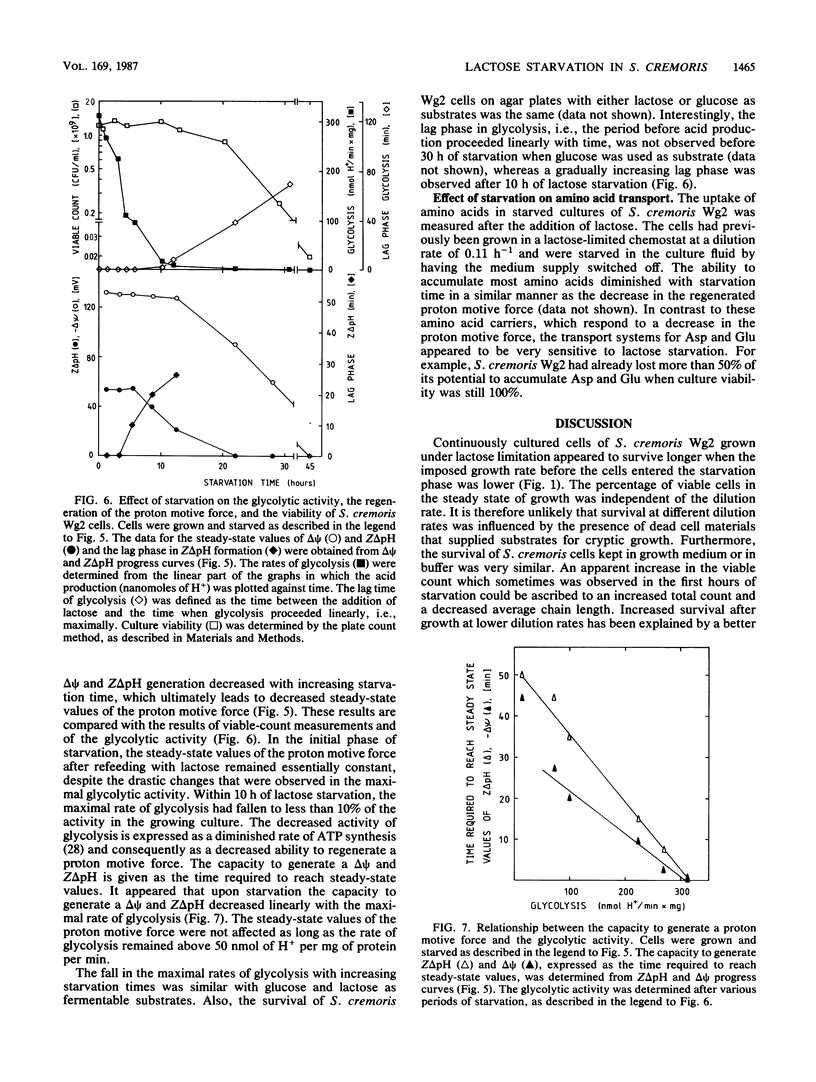
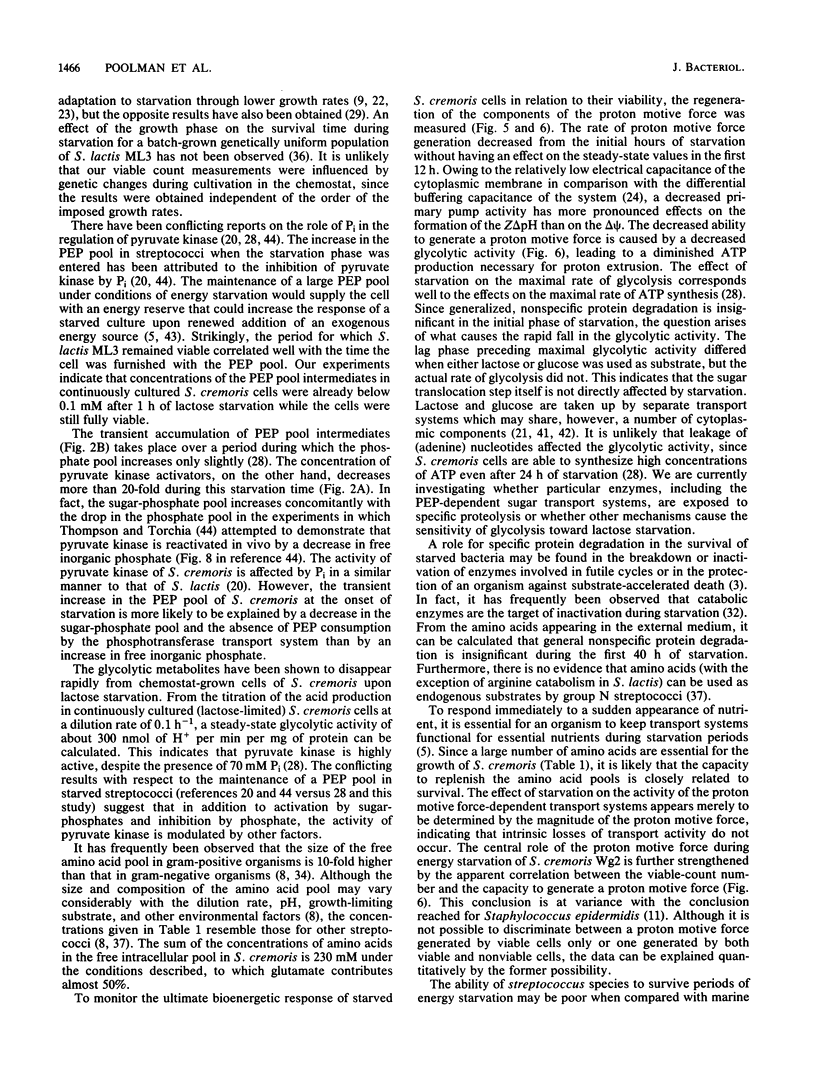
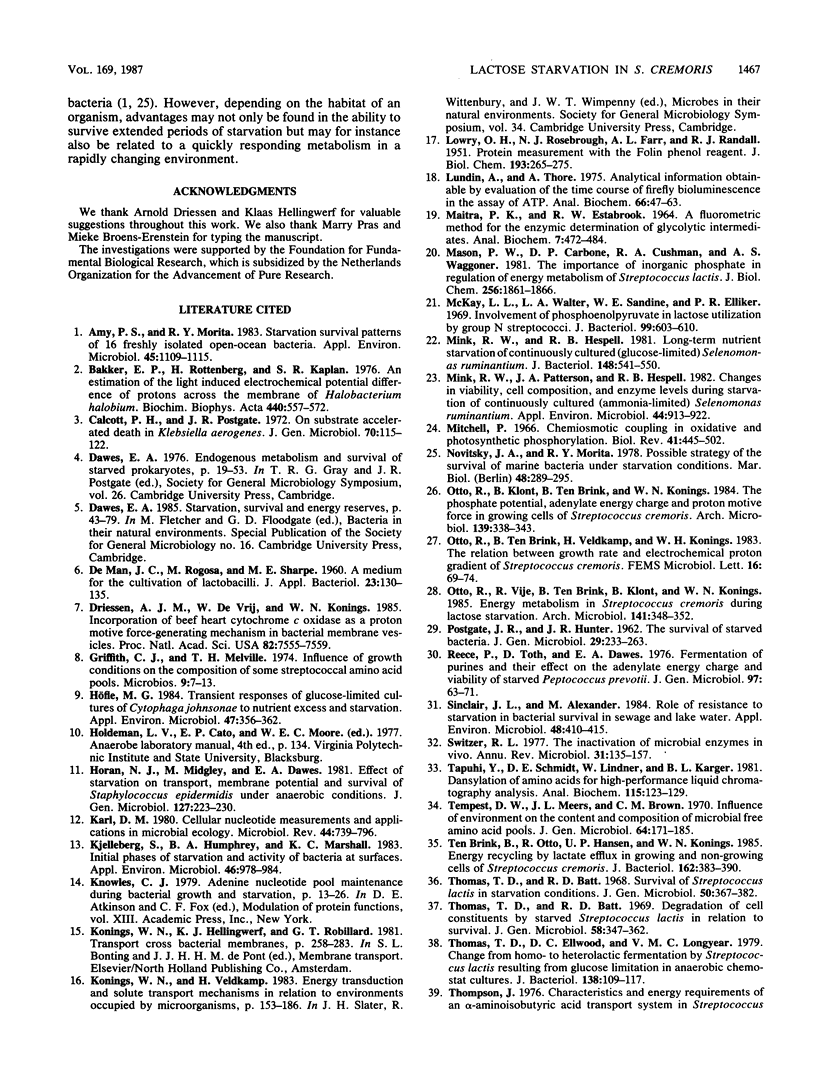
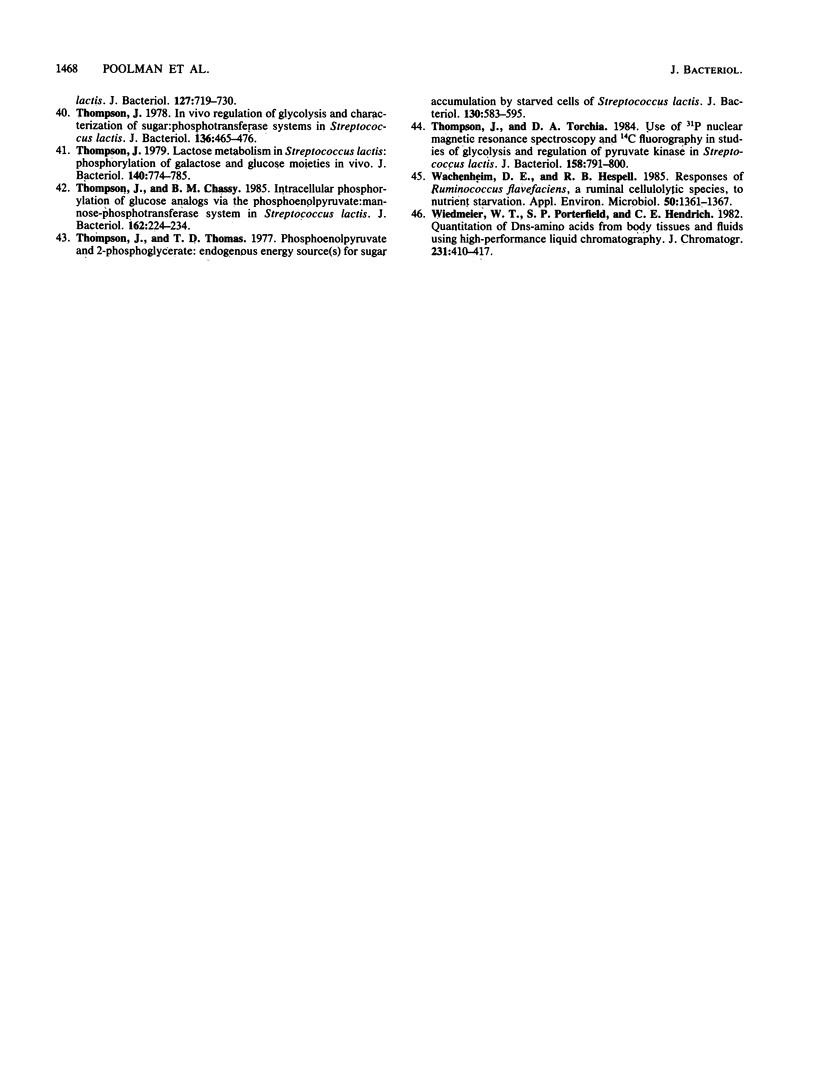
Selected References
These references are in PubMed. This may not be the complete list of references from this article.
- Amy P. S., Morita R. Y. Starvation-survival patterns of sixteen freshly isolated open-ocean bacteria. Appl Environ Microbiol. 1983 Mar;45(3):1109–1115. doi: 10.1128/aem.45.3.1109-1115.1983. [DOI] [PMC free article] [PubMed] [Google Scholar]
- Bakker E. P., Rottenberg H., Caplan S. R. An estimation of the light-induced electrochemical potential difference of protons across the membrane of Halobacterium halobium. Biochim Biophys Acta. 1976 Sep 13;440(3):557–572. doi: 10.1016/0005-2728(76)90042-6. [DOI] [PubMed] [Google Scholar]
- Calcott P. H., Postgate J. R. On substrate-accelerated death in Klebsiella aerogenes. J Gen Microbiol. 1972 Apr;70(1):115–122. doi: 10.1099/00221287-70-1-115. [DOI] [PubMed] [Google Scholar]
- Driessen A. J., de Vrij W., Konings W. N. Incorporation of beef heart cytochrome c oxidase as a proton-motive force-generating mechanism in bacterial membrane vesicles. Proc Natl Acad Sci U S A. 1985 Nov;82(22):7555–7559. doi: 10.1073/pnas.82.22.7555. [DOI] [PMC free article] [PubMed] [Google Scholar]
- Griffith C. J., Melville T. H. Influence of growth conditions on the composition of some streptococcal amino acid pools. Microbios. 1974 Jan;9(33):7–13. [PubMed] [Google Scholar]
- Horan N. J., Midgley M., Dawes E. A. Effect of starvation on transport, membrane potential and survival of Staphylococcus epidermidis under anaerobic conditions. J Gen Microbiol. 1981 Dec;127(2):223–230. doi: 10.1099/00221287-127-2-223. [DOI] [PubMed] [Google Scholar]
- Höfle M. G. Transient Responses of Glucose-Limited Cultures of Cytophaga johnsonae to Nutrient Excess and Starvation. Appl Environ Microbiol. 1984 Feb;47(2):356–362. doi: 10.1128/aem.47.2.356-362.1984. [DOI] [PMC free article] [PubMed] [Google Scholar]
- Karl D. M. Cellular nucleotide measurements and applications in microbial ecology. Microbiol Rev. 1980 Dec;44(4):739–796. doi: 10.1128/mr.44.4.739-796.1980. [DOI] [PMC free article] [PubMed] [Google Scholar]
- Kjelleberg S., Humphrey B. A., Marshall K. C. Initial phases of starvation and activity of bacteria at surfaces. Appl Environ Microbiol. 1983 Nov;46(5):978–984. doi: 10.1128/aem.46.5.978-984.1983. [DOI] [PMC free article] [PubMed] [Google Scholar]
- LOWRY O. H., ROSEBROUGH N. J., FARR A. L., RANDALL R. J. Protein measurement with the Folin phenol reagent. J Biol Chem. 1951 Nov;193(1):265–275. [PubMed] [Google Scholar]
- Lundin A., Thore A. Analytical information obtainable by evaluation of the time course of firefly bioluminescence in the assay of ATP. Anal Biochem. 1975 May 26;66(1):47–63. doi: 10.1016/0003-2697(75)90723-x. [DOI] [PubMed] [Google Scholar]
- MAITRA P. K., ESTABROOK R. W. A FLUOROMETRIC METHOD FOR THE ENZYMIC DETERMINATION OF GLYCOLYTIC INTERMEDIATES. Anal Biochem. 1964 Apr;7:472–484. doi: 10.1016/0003-2697(64)90156-3. [DOI] [PubMed] [Google Scholar]
- Mason P. W., Carbone D. P., Cushman R. A., Waggoner A. S. The importance of inorganic phosphate in regulation of energy metabolism of Streptococcus lactis. J Biol Chem. 1981 Feb 25;256(4):1861–1866. [PubMed] [Google Scholar]
- McKay L. L., Walter L. A., Sandine W. E., Elliker P. R. Involvement of phosphoenolpyruvate in lactose utilization by group N streptococci. J Bacteriol. 1969 Aug;99(2):603–610. doi: 10.1128/jb.99.2.603-610.1969. [DOI] [PMC free article] [PubMed] [Google Scholar]
- Mink R. W., Hespell R. B. Long-term nutrient starvation of continuously cultured (glucose-limited) Selenomonas ruminantium. J Bacteriol. 1981 Nov;148(2):541–550. doi: 10.1128/jb.148.2.541-550.1981. [DOI] [PMC free article] [PubMed] [Google Scholar]
- Mink R. W., Patterson J. A., Hespell R. B. Changes in Viability, Cell Composition, and Enzyme Levels During Starvation of Continuously Cultured (Ammonia-Limited) Selenomonas ruminantium. Appl Environ Microbiol. 1982 Oct;44(4):913–922. doi: 10.1128/aem.44.4.913-922.1982. [DOI] [PMC free article] [PubMed] [Google Scholar]
- Mitchell P. Chemiosmotic coupling in oxidative and photosynthetic phosphorylation. Biol Rev Camb Philos Soc. 1966 Aug;41(3):445–502. doi: 10.1111/j.1469-185x.1966.tb01501.x. [DOI] [PubMed] [Google Scholar]
- POSTGATE J. R., HUNTER J. R. The survival of starved bacteria. J Gen Microbiol. 1962 Oct;29:233–263. doi: 10.1099/00221287-29-2-233. [DOI] [PubMed] [Google Scholar]
- Reece P., Toth D., Dawes E. A. Fermentation of purines and their effect on the adenylate energy charge and viability of starved Peptococcus prévotii. J Gen Microbiol. 1976 Nov;97(1):63–71. doi: 10.1099/00221287-97-1-63. [DOI] [PubMed] [Google Scholar]
- Sinclair J. L., Alexander M. Role of resistance to starvation in bacterial survival in sewage and lake water. Appl Environ Microbiol. 1984 Aug;48(2):410–415. doi: 10.1128/aem.48.2.410-415.1984. [DOI] [PMC free article] [PubMed] [Google Scholar]
- Switzer R. L. The inactivation of microbial enzymes in vivo. Annu Rev Microbiol. 1977;31:135–157. doi: 10.1146/annurev.mi.31.100177.001031. [DOI] [PubMed] [Google Scholar]
- Tapuhi Y., Schmidt D. E., Lindner W., Karger B. L. Dansylation of amino acids for high-performance liquid chromatography analysis. Anal Biochem. 1981 Jul 15;115(1):123–129. doi: 10.1016/0003-2697(81)90534-0. [DOI] [PubMed] [Google Scholar]
- Tempest D. W., Meers J. L., Brown C. M. Influence of environment on the content and composition of microbial free amino acid pools. J Gen Microbiol. 1970 Dec;64(2):171–185. doi: 10.1099/00221287-64-2-171. [DOI] [PubMed] [Google Scholar]
- Thomas T. D., Batt R. D. Degradation of cell constituents by starved Streptococcus lactis in relation to survival. J Gen Microbiol. 1969 Nov;58(3):347–362. doi: 10.1099/00221287-58-3-347. [DOI] [PubMed] [Google Scholar]
- Thomas T. D., Batt R. D. Survival of Streptococcus lactis in starvation conditions. J Gen Microbiol. 1968 Mar;50(3):367–382. doi: 10.1099/00221287-50-3-367. [DOI] [PubMed] [Google Scholar]
- Thomas T. D., Ellwood D. C., Longyear V. M. Change from homo- to heterolactic fermentation by Streptococcus lactis resulting from glucose limitation in anaerobic chemostat cultures. J Bacteriol. 1979 Apr;138(1):109–117. doi: 10.1128/jb.138.1.109-117.1979. [DOI] [PMC free article] [PubMed] [Google Scholar]
- Thompson J., Chassy B. M. Intracellular phosphorylation of glucose analogs via the phosphoenolpyruvate: mannose-phosphotransferase system in Streptococcus lactis. J Bacteriol. 1985 Apr;162(1):224–234. doi: 10.1128/jb.162.1.224-234.1985. [DOI] [PMC free article] [PubMed] [Google Scholar]
- Thompson J. In vivo regulation of glycolysis and characterization of sugar: phosphotransferase systems in Streptococcus lactis. J Bacteriol. 1978 Nov;136(2):465–476. doi: 10.1128/jb.136.2.465-476.1978. [DOI] [PMC free article] [PubMed] [Google Scholar]
- Thompson J. Lactose metabolism in Streptococcus lactis: phosphorylation of galactose and glucose moieties in vivo. J Bacteriol. 1979 Dec;140(3):774–785. doi: 10.1128/jb.140.3.774-785.1979. [DOI] [PMC free article] [PubMed] [Google Scholar]
- Thompson J., Thomas T. D. Phosphoenolpyruvate and 2-phosphoglycerate: endogenous energy source(s) for sugar accumulation by starved cells of Streptococcus lactis. J Bacteriol. 1977 May;130(2):583–595. doi: 10.1128/jb.130.2.583-595.1977. [DOI] [PMC free article] [PubMed] [Google Scholar]
- Thompson J., Torchia D. A. Use of 31P nuclear magnetic resonance spectroscopy and 14C fluorography in studies of glycolysis and regulation of pyruvate kinase in Streptococcus lactis. J Bacteriol. 1984 Jun;158(3):791–800. doi: 10.1128/jb.158.3.791-800.1984. [DOI] [PMC free article] [PubMed] [Google Scholar]
- Wachenheim D. E., Hespell R. B. Responses of Ruminococcus flavefaciens, a Ruminal Cellulolytic Species, to Nutrient Starvation. Appl Environ Microbiol. 1985 Dec;50(6):1361–1367. doi: 10.1128/aem.50.6.1361-1367.1985. [DOI] [PMC free article] [PubMed] [Google Scholar]
- Wiedmeier V. T., Porterfield S. P., Hendrich C. E. Quantitation of Dns-amino acids from body tissues and fluids using high-performance liquid chromatography. J Chromatogr. 1982 Sep 10;231(2):410–417. doi: 10.1016/s0378-4347(00)81865-4. [DOI] [PubMed] [Google Scholar]
- ten Brink B., Otto R., Hansen U. P., Konings W. N. Energy recycling by lactate efflux in growing and nongrowing cells of Streptococcus cremoris. J Bacteriol. 1985 Apr;162(1):383–390. doi: 10.1128/jb.162.1.383-390.1985. [DOI] [PMC free article] [PubMed] [Google Scholar]