Abstract
The mechanism of methyl-beta-D-thiogalactoside-phosphate (TMG-P) expulsion from Streptococcus pyogenes was studied. The expulsion elicited by glucose was not due to exchange vectorial transphosphorylation between the expelled TMG and the incoming glucose since more beta-galactoside was displaced than glucose taken up, and the stoichiometry between TMG and glucose transport was inconstant. Instead, two distinct and sequential reactions, intracellular dephosphorylation of TMG-P followed by efflux of free TMG, mediated the expulsion. This was shown by temporary accumulation of free TMG effected by competitive inhibition of its efflux and by the aid of arsenate, which arrested dephosphorylation of TMG-P but did not affect efflux of free TMG formed intracellularly before arsenate addition. The competitive inhibition of TMG efflux by its structural analogs suggests that a transport protein facilitates the expulsion. Iodoacetate or fluoride prevented TMG-P dephosphorylation and its expulsion. However, provision of ATP via the arginine deiminase pathway restored these activities in the presence of the glycolytic inhibitors and stimulated expulsion in their absence. Other amino acids tested did not promote this restoration, and canavanine or norvaline severely inhibited it. Arginine without glucose neither elicited the dephosphorylation nor evoked the expulsion of TMG-P. Ionophores or ATPase inhibitors did not prevent the expulsion as elicited by glucose or its restoration by arginine. The results suggest that activation of the dephosphorylation-expulsion mechanism occurs independently of a functional glycolytic pathway, requires ATP provision, and is possibly due to protein phosphorylation controlled by a yet unknown metabolite. The in vivo phosphorylation of a protein (approximate molecular weight - 10,000) under the conditions of expulsion was demonstrated.
Full text
PDF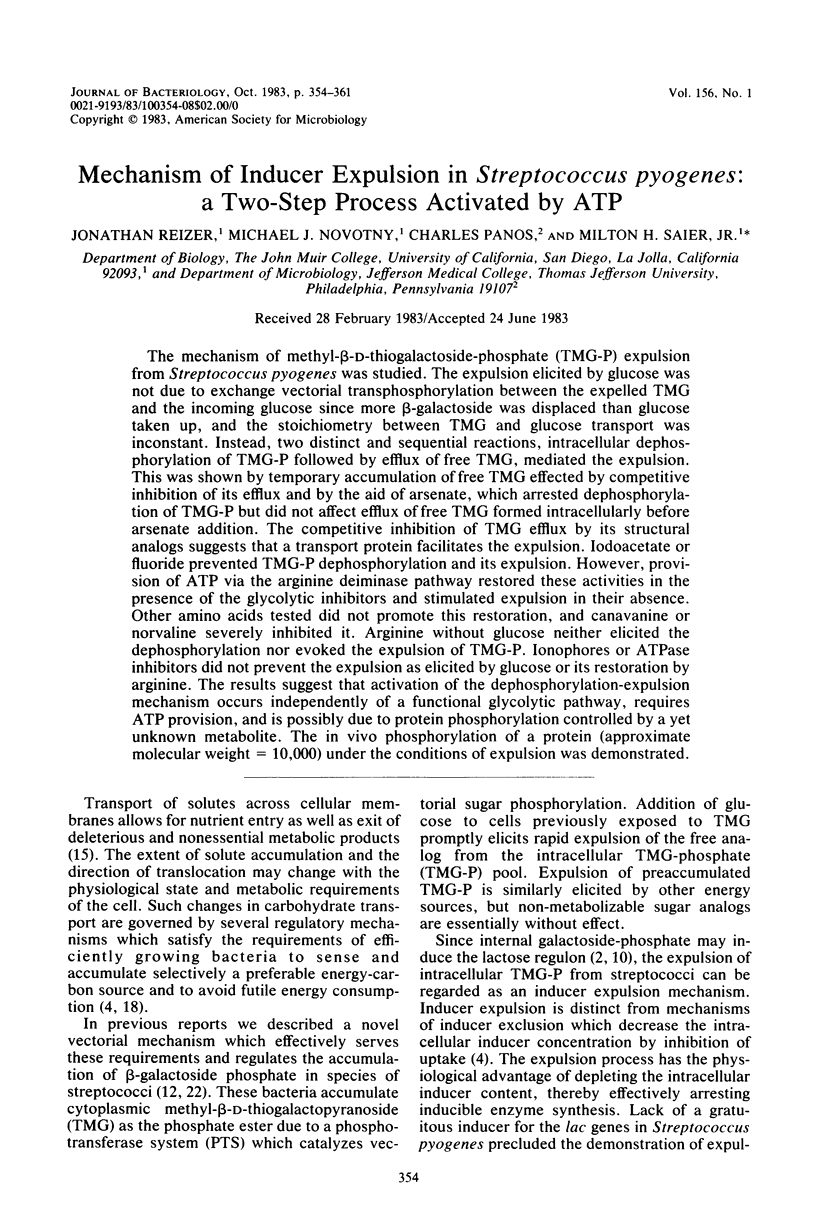
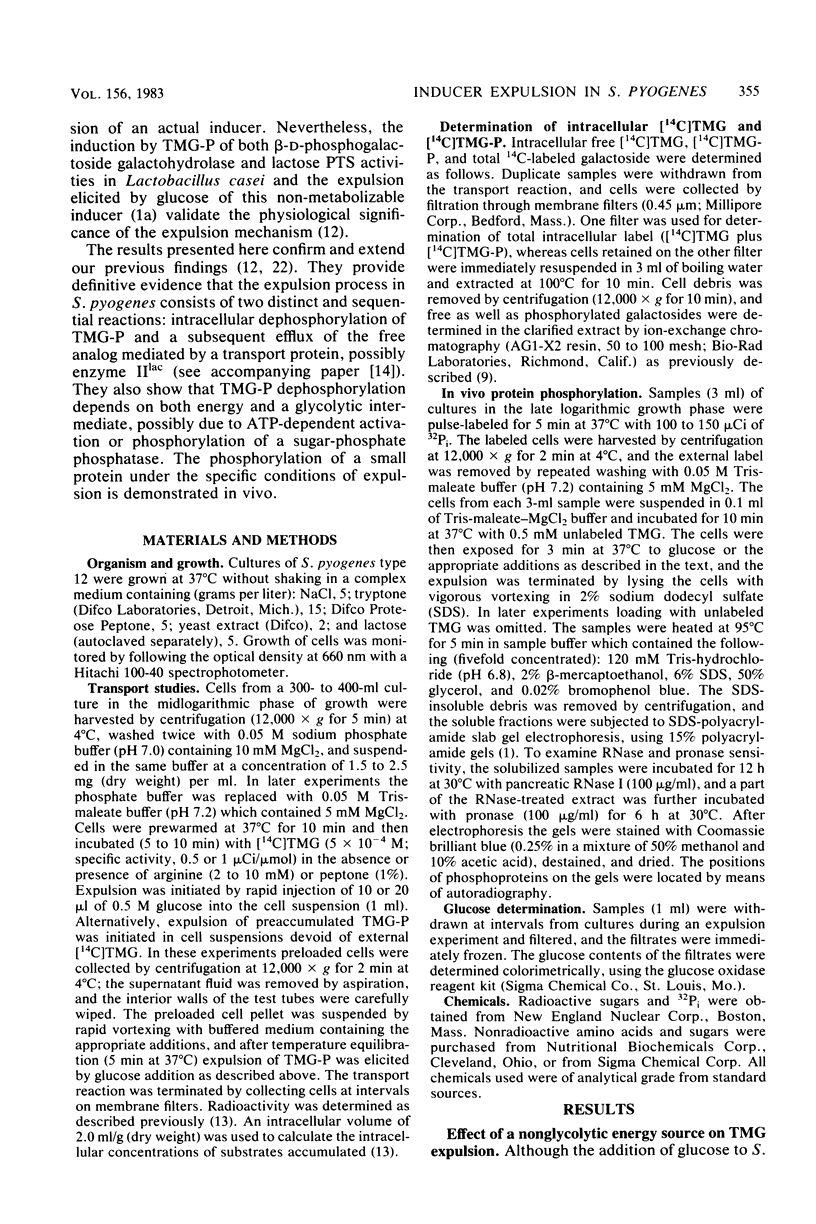
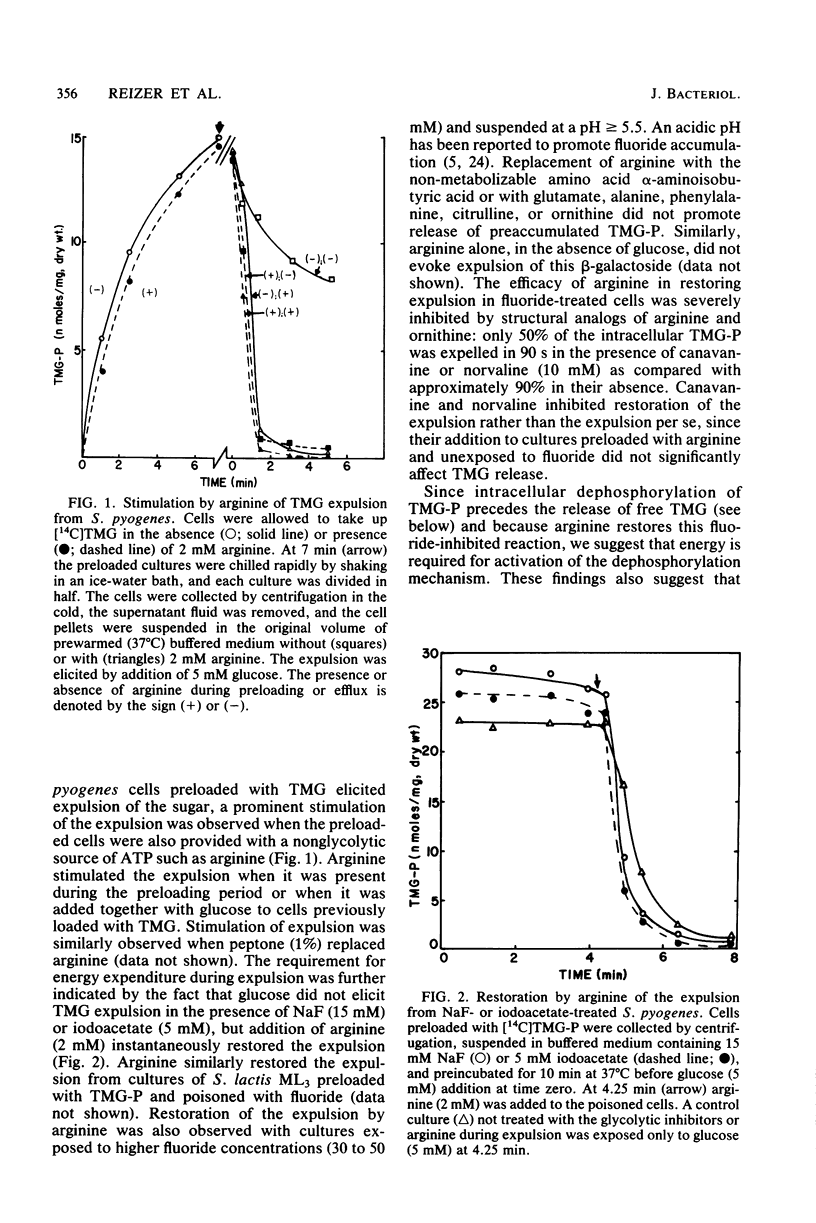
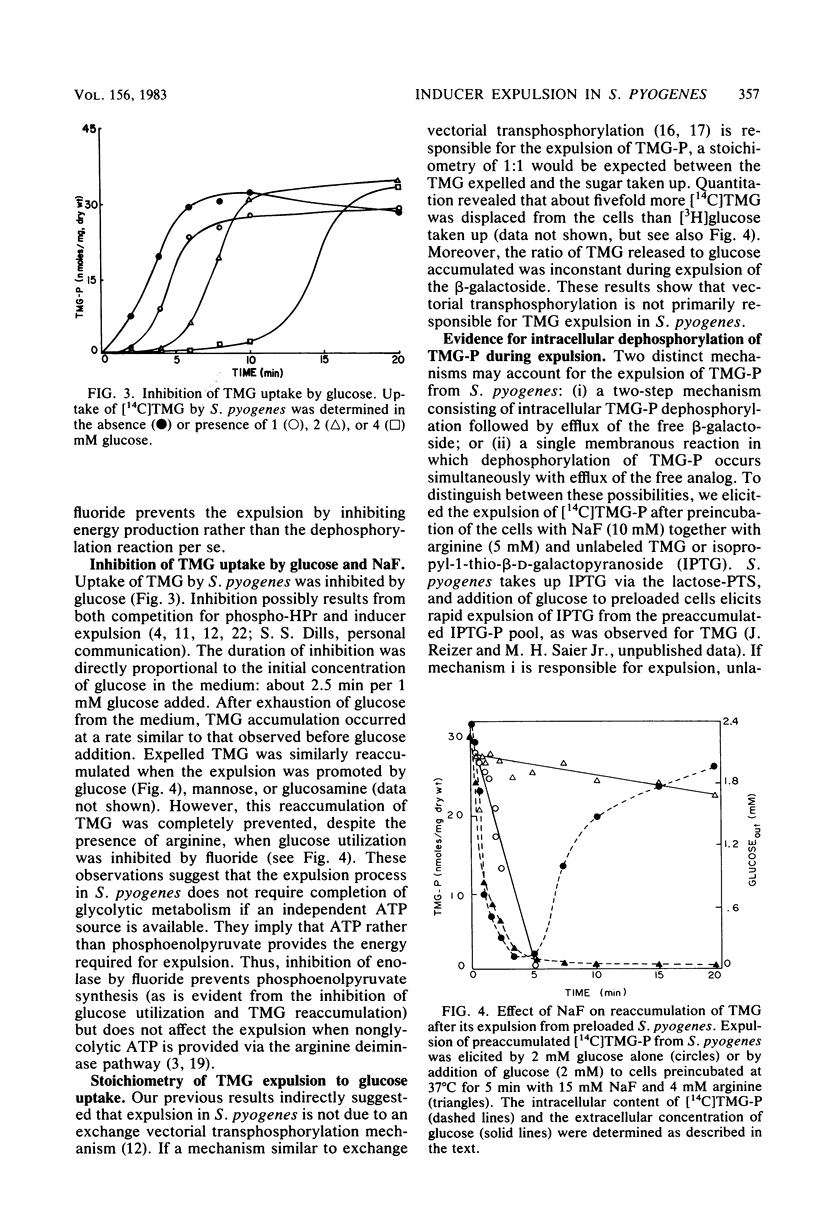
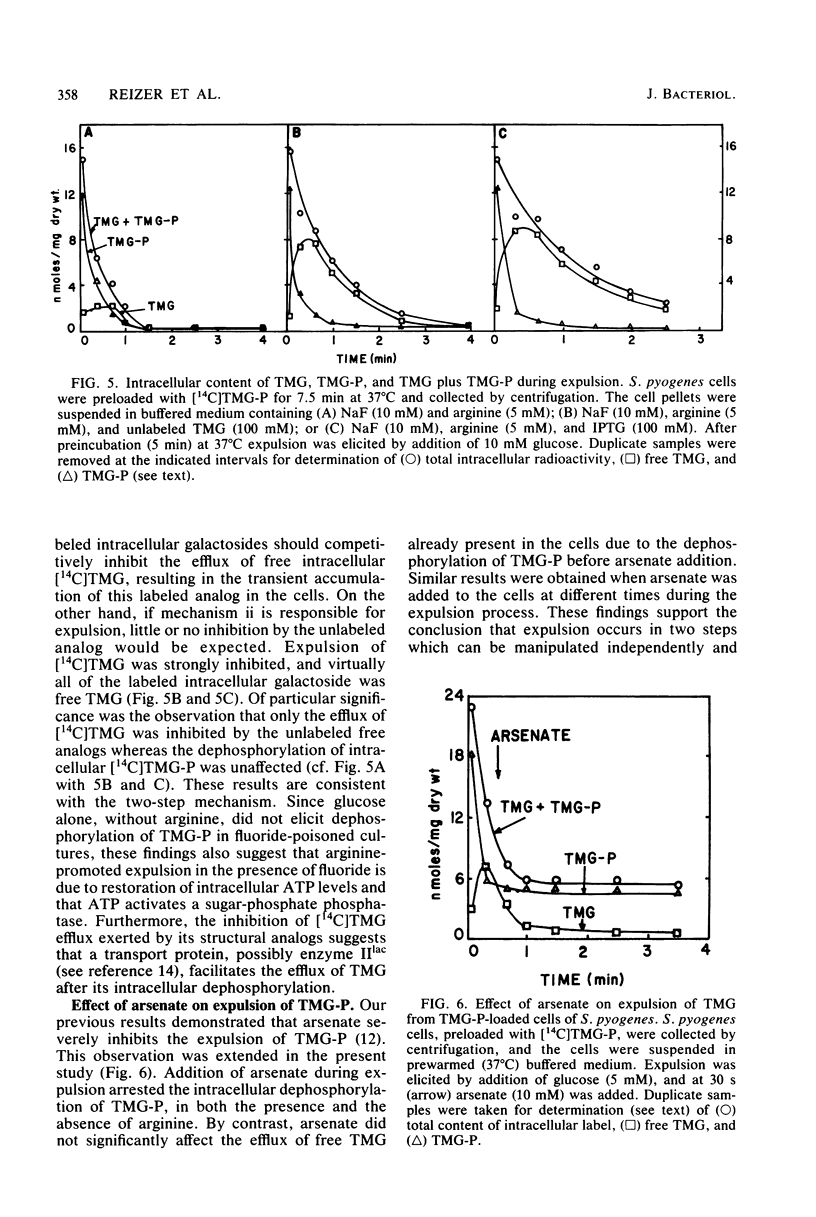
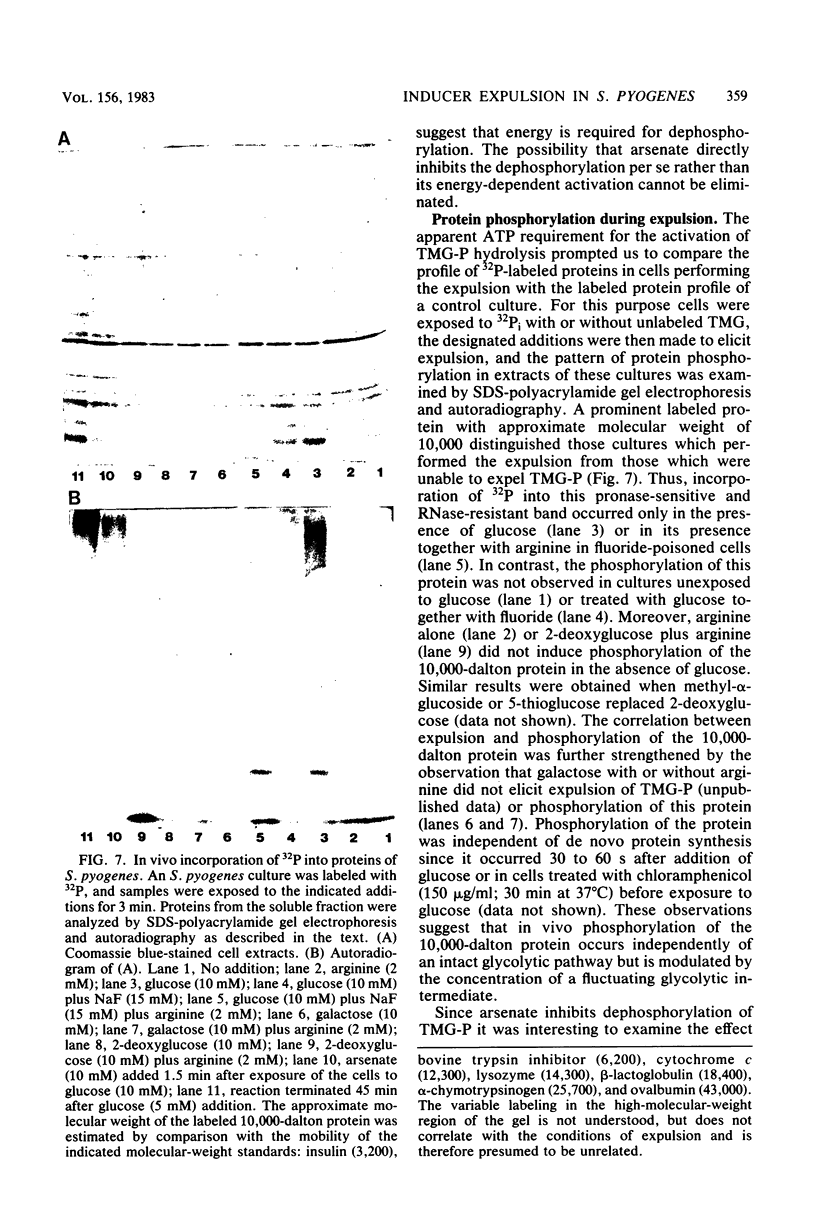
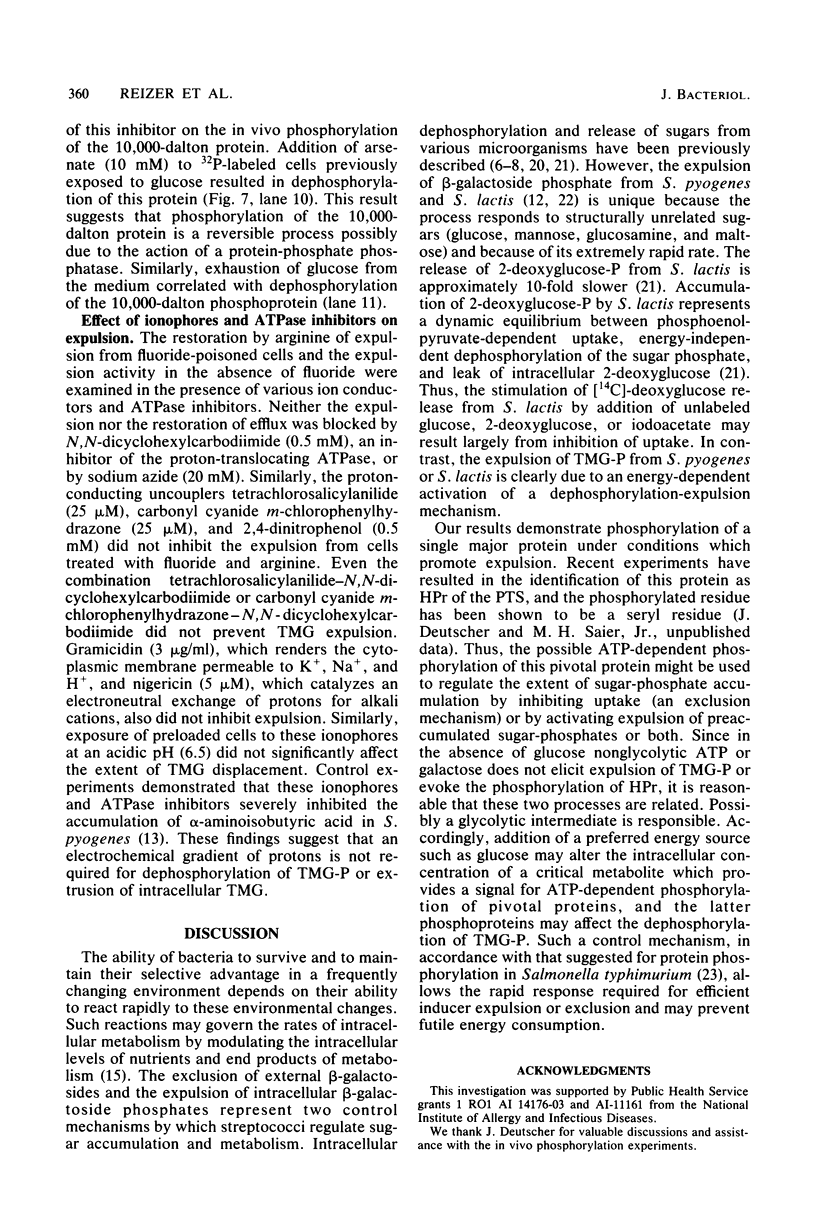
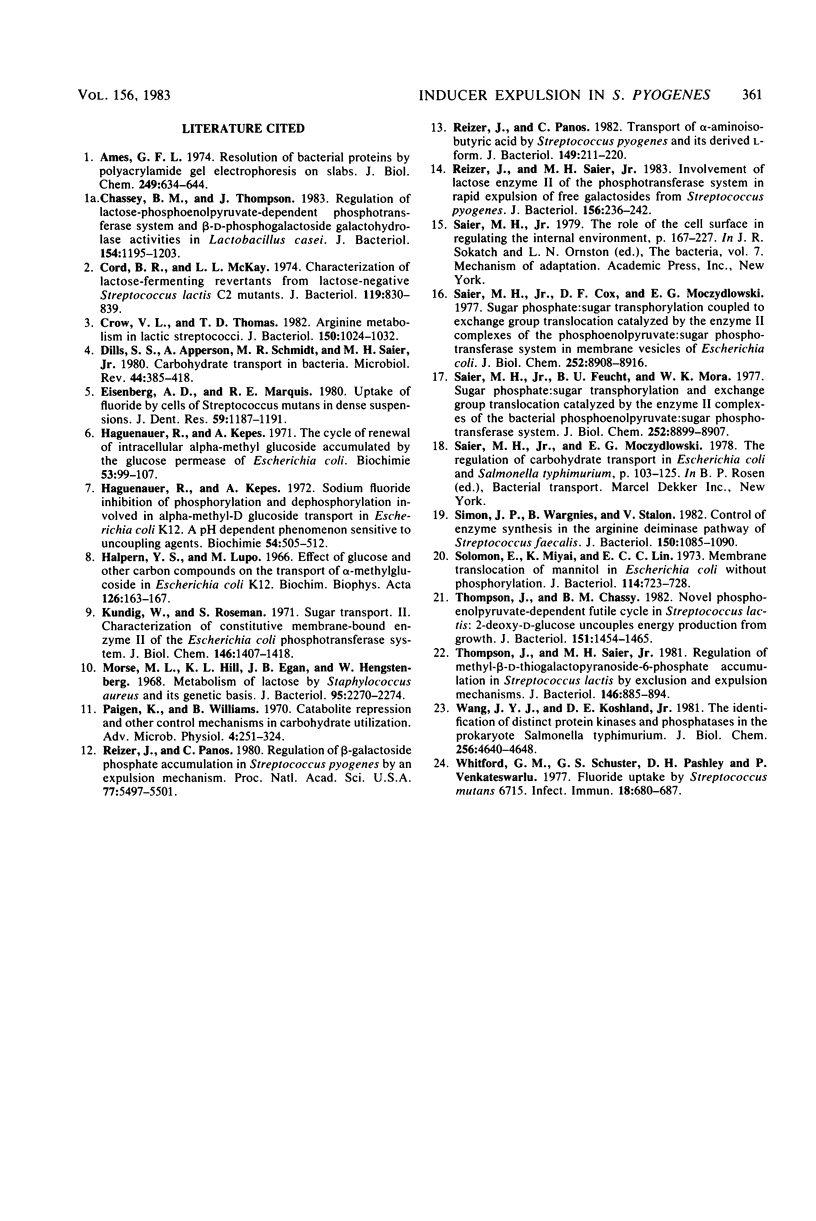
Images in this article
Selected References
These references are in PubMed. This may not be the complete list of references from this article.
- Ames G. F. Resolution of bacterial proteins by polyacrylamide gel electrophoresis on slabs. Membrane, soluble, and periplasmic fractions. J Biol Chem. 1974 Jan 25;249(2):634–644. [PubMed] [Google Scholar]
- Chassy B. M., Thompson J. Regulation of lactose-phosphoenolpyruvate-dependent phosphotransferase system and beta-D-phosphogalactoside galactohydrolase activities in Lactobacillus casei. J Bacteriol. 1983 Jun;154(3):1195–1203. doi: 10.1128/jb.154.3.1195-1203.1983. [DOI] [PMC free article] [PubMed] [Google Scholar]
- Cords B. R., McKay L. L. Characterization of lactose-fermenting revertants from lactose-negative Streptococcus lactis C2 mutants. J Bacteriol. 1974 Sep;119(3):830–839. doi: 10.1128/jb.119.3.830-839.1974. [DOI] [PMC free article] [PubMed] [Google Scholar]
- Crow V. L., Thomas T. D. Arginine metabolism in lactic streptococci. J Bacteriol. 1982 Jun;150(3):1024–1032. doi: 10.1128/jb.150.3.1024-1032.1982. [DOI] [PMC free article] [PubMed] [Google Scholar]
- Dills S. S., Apperson A., Schmidt M. R., Saier M. H., Jr Carbohydrate transport in bacteria. Microbiol Rev. 1980 Sep;44(3):385–418. doi: 10.1128/mr.44.3.385-418.1980. [DOI] [PMC free article] [PubMed] [Google Scholar]
- Eisenberg A. D., Marquis R. E. Uptake of fluoride by cells of Streptococcus mutans in dense suspensions. J Dent Res. 1980 Jul;59(7):1187–1191. doi: 10.1177/00220345800590072801. [DOI] [PubMed] [Google Scholar]
- Haguenauer R., Kepes A. NaF inhibition of phosphorylation and dephosphorylation involved in -methyl-D glucoside transport in E. coli K 12. A pH dependant phenomenon sensitive to uncoupling agents. Biochimie. 1972;54(4):505–512. doi: 10.1016/s0300-9084(72)80235-9. [DOI] [PubMed] [Google Scholar]
- Halpern Y. S., Lupo M. Effect of glucose and other carbon compounds on the transport of alpha-methylglucoside in Escherichia coli K12. Biochim Biophys Acta. 1966 Sep 5;126(1):163–167. doi: 10.1016/0926-6585(66)90046-x. [DOI] [PubMed] [Google Scholar]
- Kundig W., Roseman S. Sugar transport. II. Characterization of constitutive membrane-bound enzymes II of the Escherichia coli phosphotransferase system. J Biol Chem. 1971 Mar 10;246(5):1407–1418. [PubMed] [Google Scholar]
- Morse M. L., Hill K. L., Egan J. B., Hengstenberg W. Metabolism of lactose by Staphylococcus aureus and its genetic basis. J Bacteriol. 1968 Jun;95(6):2270–2274. doi: 10.1128/jb.95.6.2270-2274.1968. [DOI] [PMC free article] [PubMed] [Google Scholar]
- Reizer J., Panos C. Regulation of beta-galactoside phosphate accumulation in Streptococcus pyogenes by an expulsion mechanism. Proc Natl Acad Sci U S A. 1980 Sep;77(9):5497–5501. doi: 10.1073/pnas.77.9.5497. [DOI] [PMC free article] [PubMed] [Google Scholar]
- Reizer J., Panos C. Transport of alpha-aminoisobutyric acid by Streptococcus pyogenes and its derived L-form. J Bacteriol. 1982 Jan;149(1):211–220. doi: 10.1128/jb.149.1.211-220.1982. [DOI] [PMC free article] [PubMed] [Google Scholar]
- Reizer J., Saier M. H., Jr Involvement of lactose enzyme II of the phosphotransferase system in rapid expulsion of free galactosides from Streptococcus pyogenes. J Bacteriol. 1983 Oct;156(1):236–242. doi: 10.1128/jb.156.1.236-242.1983. [DOI] [PMC free article] [PubMed] [Google Scholar]
- Saier M. H., Jr, Cox D. F., Moczydlowski E. G. Sugar phosphate:sugar transphosphorylation coupled to exchange group translocation catalyzed by the enzyme II complexes of the phosphoenolpyruvate:sugar phosphotransferase system in membrane vesicles of Escherichia coli. J Biol Chem. 1977 Dec 25;252(24):8908–8916. [PubMed] [Google Scholar]
- Saier M. H., Jr, Feucht B. U., Mora W. K. Sugar phosphate: sugar transphosphorylation and exchange group translocation catalyzed by the enzyme 11 complexes of the bacterial phosphoenolpyruvate: sugar phosphotransferase system. J Biol Chem. 1977 Dec 25;252(24):8899–8907. [PubMed] [Google Scholar]
- Simon J. P., Wargnies B., Stalon V. Control of enzyme synthesis in the arginine deiminase pathway of Streptococcus faecalis. J Bacteriol. 1982 Jun;150(3):1085–1090. doi: 10.1128/jb.150.3.1085-1090.1982. [DOI] [PMC free article] [PubMed] [Google Scholar]
- Solomon E., Miyal K., Lin E. C. Membrane translocation of mannitol in Escherichia coli without phosphorylation. J Bacteriol. 1973 May;114(2):723–728. doi: 10.1128/jb.114.2.723-728.1973. [DOI] [PMC free article] [PubMed] [Google Scholar]
- Thompson J., Chassy B. M. Novel phosphoenolpyruvate-dependent futile cycle in Streptococcus lactis: 2-deoxy-D-glucose uncouples energy production from growth. J Bacteriol. 1982 Sep;151(3):1454–1465. doi: 10.1128/jb.151.3.1454-1465.1982. [DOI] [PMC free article] [PubMed] [Google Scholar]
- Thompson J., Saier M. H., Jr Regulation of methyl-beta-d-thiogalactopyranoside-6-phosphate accumulation in Streptococcus lactis by exclusion and expulsion mechanisms. J Bacteriol. 1981 Jun;146(3):885–894. doi: 10.1128/jb.146.3.885-894.1981. [DOI] [PMC free article] [PubMed] [Google Scholar]
- Wang J. Y., Koshland D. E., Jr The identification of distinct protein kinases and phosphatases in the prokaryote Salmonella typhimurium. J Biol Chem. 1981 May 10;256(9):4640–4648. [PubMed] [Google Scholar]
- Whitford G. M., Schuster G. S., Pashley D. H., Venkateswarlu P. Fluoride uptake by Streptococcus mutans 6715. Infect Immun. 1977 Dec;18(3):680–687. doi: 10.1128/iai.18.3.680-687.1977. [DOI] [PMC free article] [PubMed] [Google Scholar]