Abstract
After growth in appropriate media, resting cells of Streptococcus lactis 7962 showed a rapid exchange between external and internal pools of inorganic phosphate. This exchange was not found in other strains of S. lactis (ML3, 133, or K1) or in Streptococcus faecalis. Phosphate exchange in S. lactis 7962 did not require other anions or cations in the assay medium, nor was phosphate influx affected by the membrane potential and pH gradient formed during glycolysis. Thus, the exchange reaction was independent of known ionic drivers (H+, Na+, OH-, etc.). Experiments testing inhibitions of phosphate entry suggested that alternative substrates for exchange included arsenate, as well as the 6-phosphates of glucose, 2-deoxyglucose, fructose, mannose, or glucosamine, and direct studies with 2-deoxyglucose 6-phosphate verified that resting cells could accumulate this sugar phosphate to levels expected for exchange with internal phosphate. Two other observations supported the idea of an exchange between phosphate and sugar phosphate. First, early addition of the heterologous substrate blocked entry of the test compound, whereas later addition caused efflux of preaccumulated material. Second, expression of phosphate exchange and 2-deoxyglucose 6-phosphate transport varied in parallel. Both activities were found at high levels after growth in medium supplemented with rhamnose or arabinose, at intermediate levels with addition of galactose, and at low levels after growth with glucose, fructose, or mannose. We conclude that these findings describe a novel anion antiporter that mediates the exchange of phosphate (arsenate) and sugar 6-phosphates.
Full text
PDF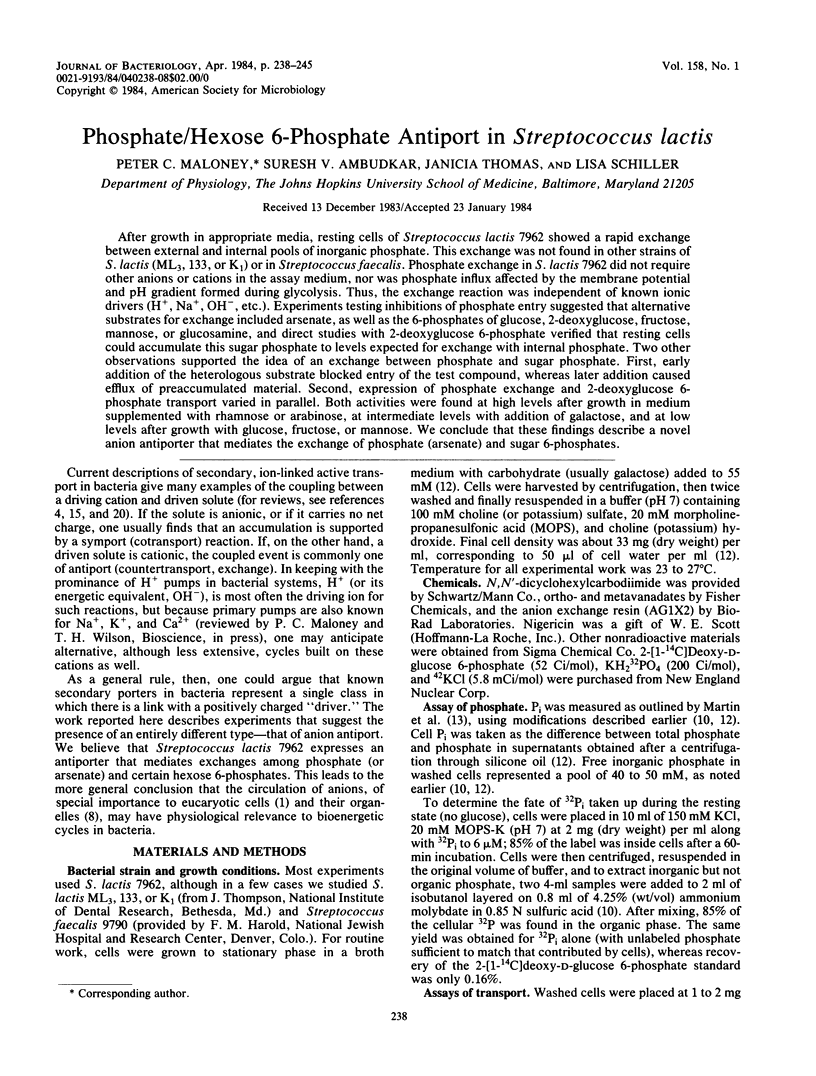
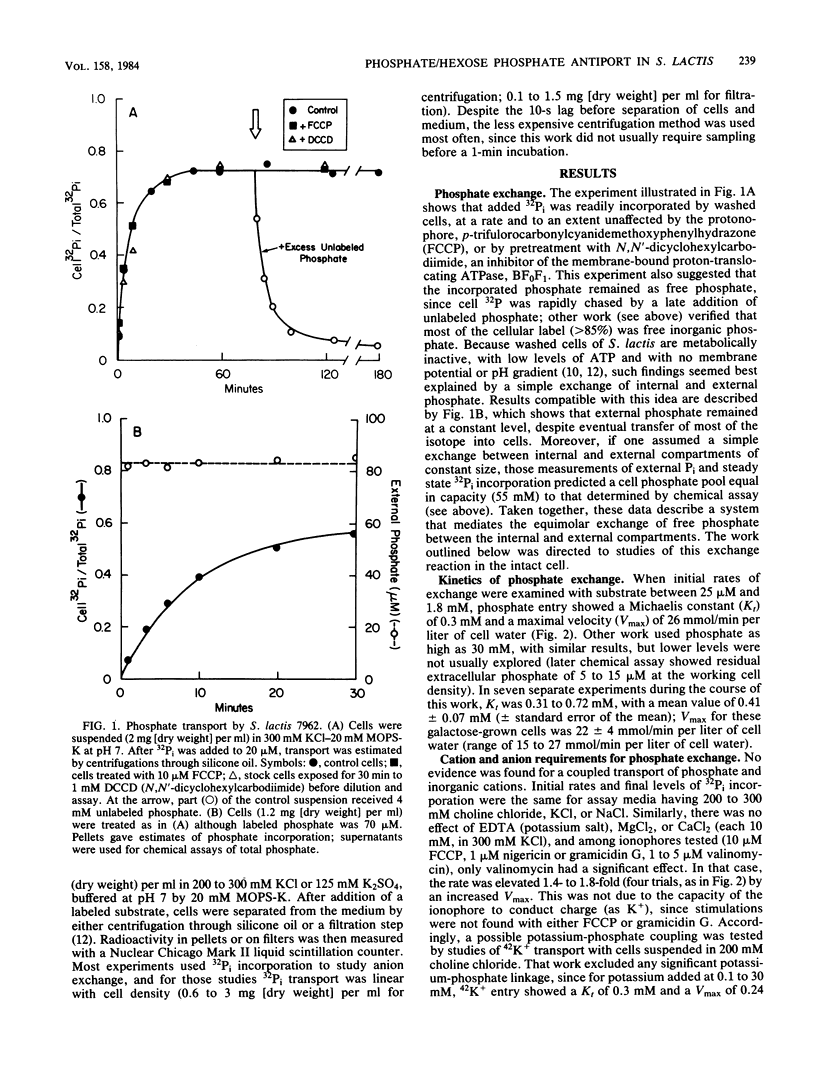
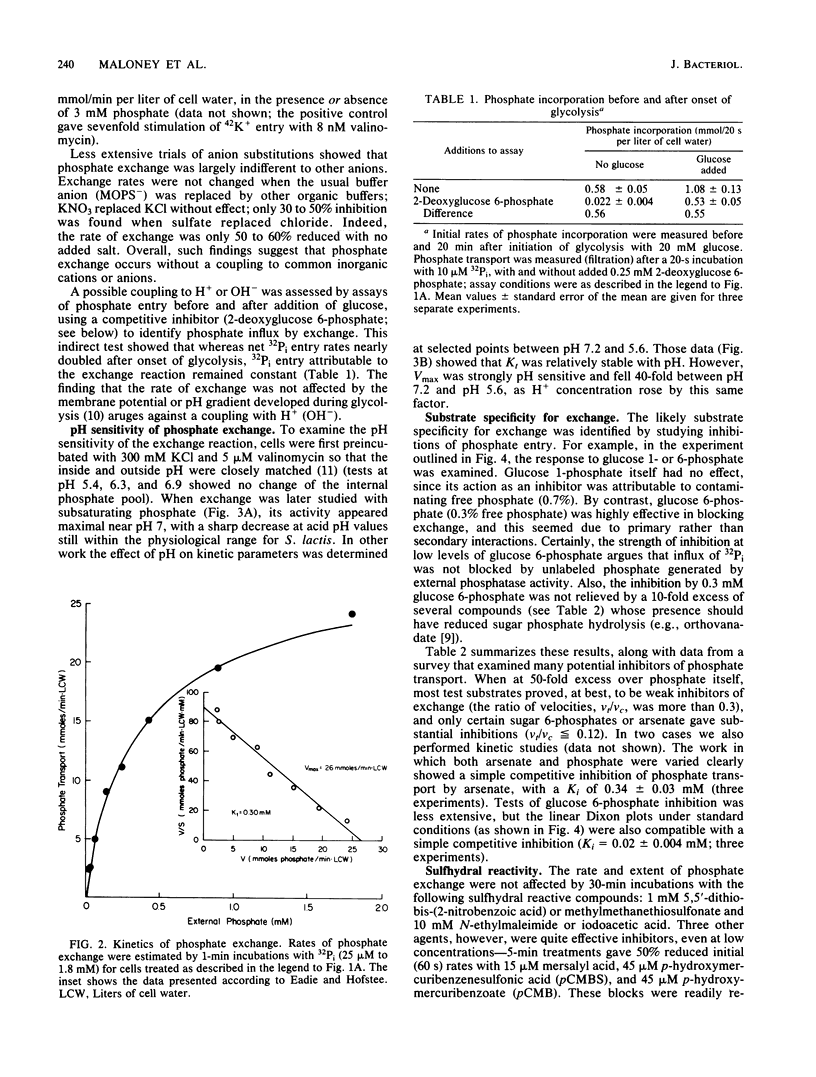
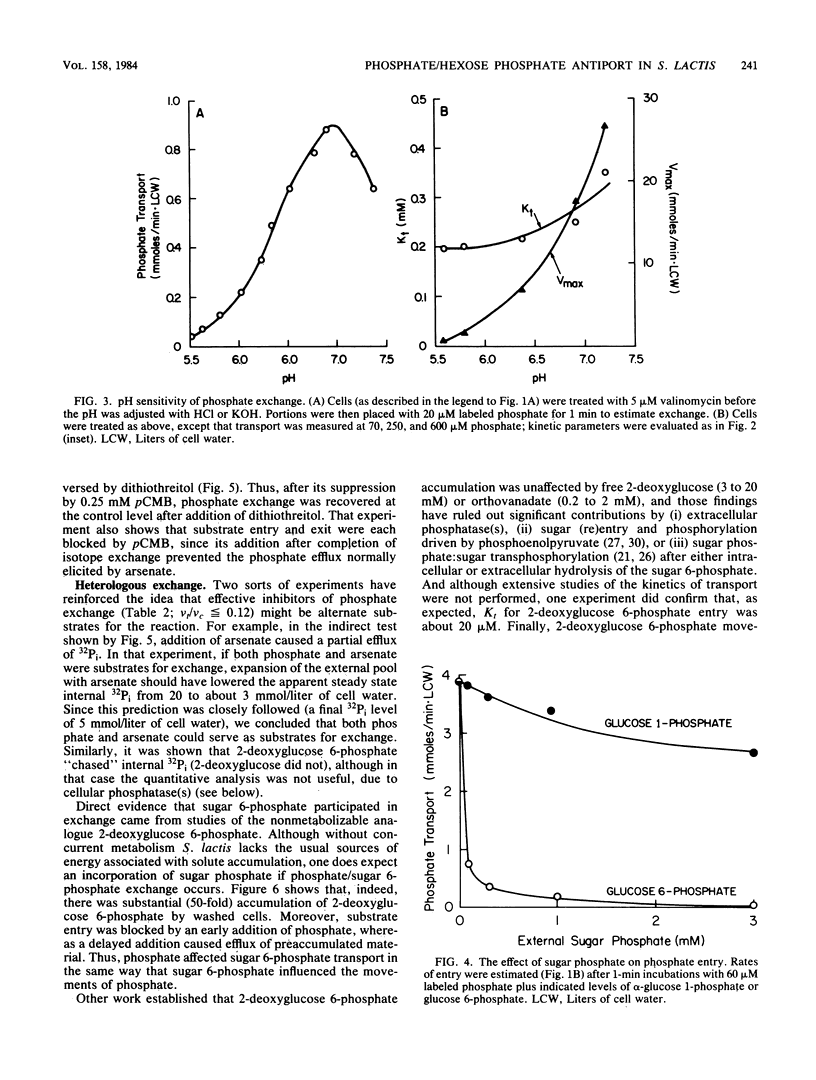
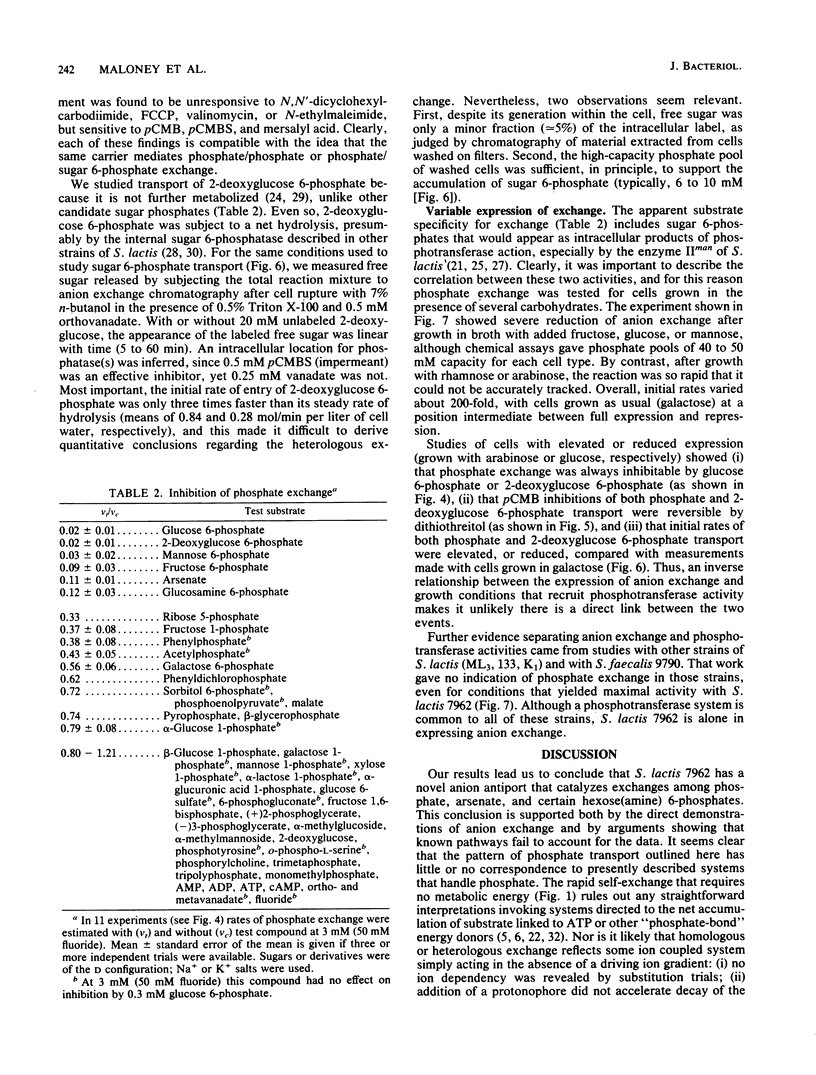
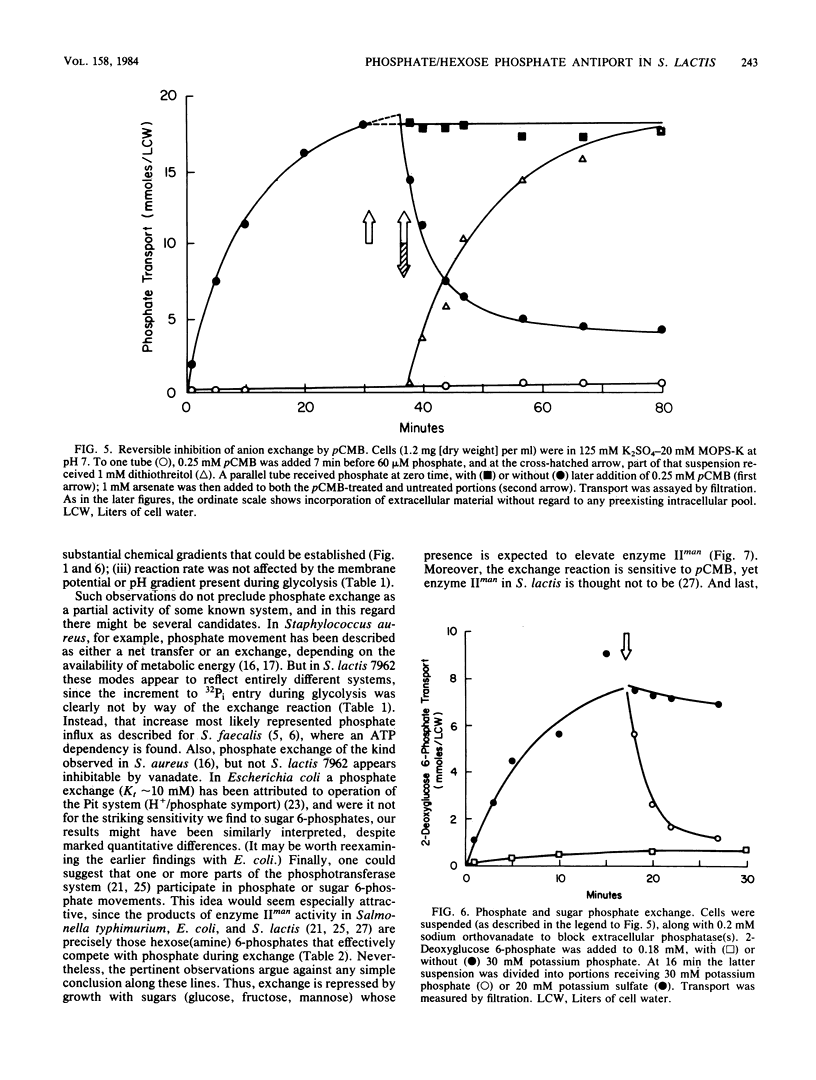
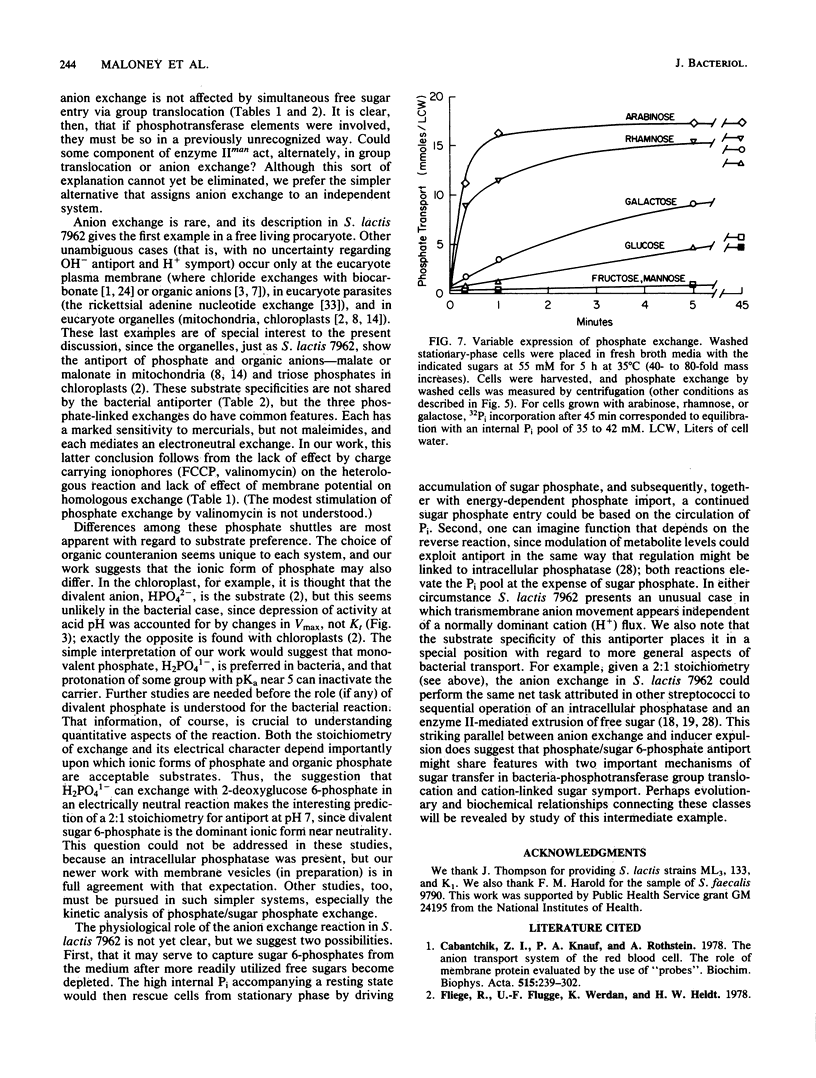
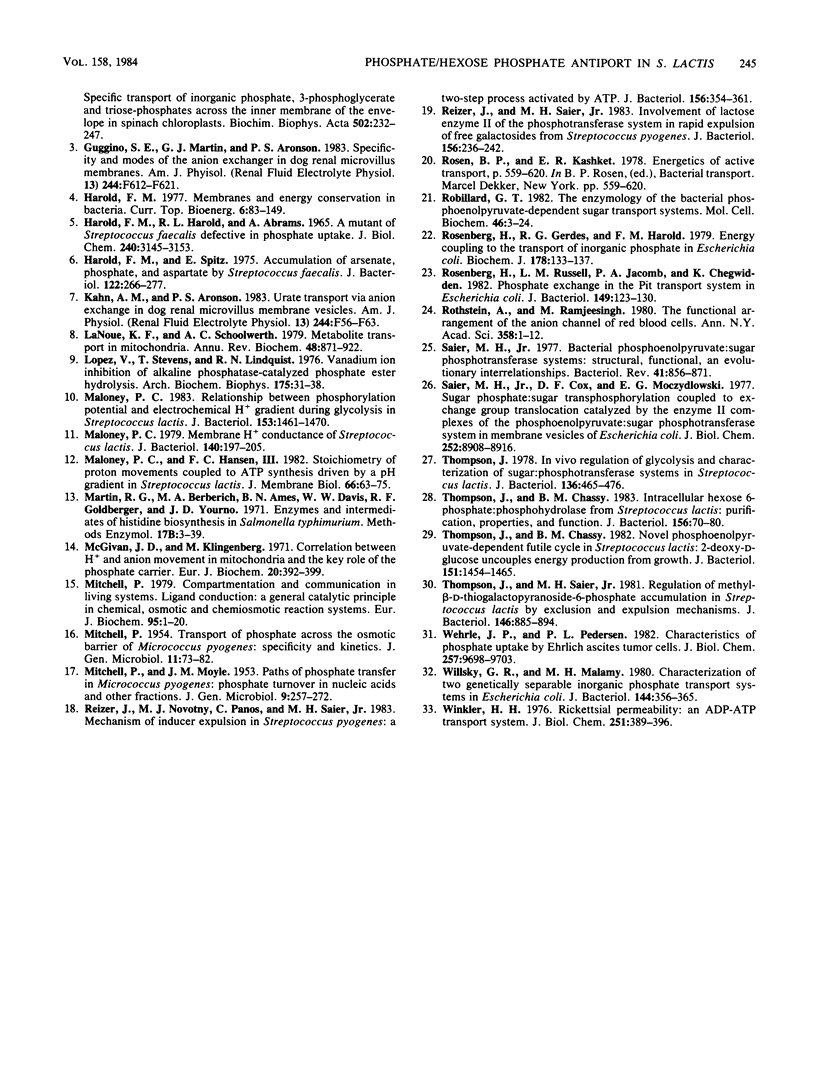
Selected References
These references are in PubMed. This may not be the complete list of references from this article.
- Cabantchik Z. I., Knauf P. A., Rothstein A. The anion transport system of the red blood cell. The role of membrane protein evaluated by the use of 'probes'. Biochim Biophys Acta. 1978 Sep 29;515(3):239–302. doi: 10.1016/0304-4157(78)90016-3. [DOI] [PubMed] [Google Scholar]
- Fliege R., Flügge U. I., Werdan K., Heldt H. W. Specific transport of inorganic phosphate, 3-phosphoglycerate and triosephosphates across the inner membrane of the envelope in spinach chloroplasts. Biochim Biophys Acta. 1978 May 10;502(2):232–247. doi: 10.1016/0005-2728(78)90045-2. [DOI] [PubMed] [Google Scholar]
- Guggino S. E., Martin G. J., Aronson P. S. Specificity and modes of the anion exchanger in dog renal microvillus membranes. Am J Physiol. 1983 Jun;244(6):F612–F621. doi: 10.1152/ajprenal.1983.244.6.F612. [DOI] [PubMed] [Google Scholar]
- HAROLD F. M., HAROLD R. L., ABRAMS A. A MUTANT OF STREPTOCOCCUS FAECALIS DEFECTIVE IN PHOSPHATE UPTAKE. J Biol Chem. 1965 Jul;240:3145–3153. [PubMed] [Google Scholar]
- Harold F. M., Spitz E. Accumulation of arsenate, phosphate, and aspartate by Sreptococcus faecalis. J Bacteriol. 1975 Apr;122(1):266–277. doi: 10.1128/jb.122.1.266-277.1975. [DOI] [PMC free article] [PubMed] [Google Scholar]
- Kahn A. M., Aronson P. S. Urate transport via anion exchange in dog renal microvillus membrane vesicles. Am J Physiol. 1983 Jan;244(1):F56–F63. doi: 10.1152/ajprenal.1983.244.1.F56. [DOI] [PubMed] [Google Scholar]
- LaNoue K. F., Schoolwerth A. C. Metabolite transport in mitochondria. Annu Rev Biochem. 1979;48:871–922. doi: 10.1146/annurev.bi.48.070179.004255. [DOI] [PubMed] [Google Scholar]
- Lopez V., Stevens T., Lindquist R. N. Vanadium ion inhibition of alkaline phosphatase-catalyzed phosphate ester hydrolysis. Arch Biochem Biophys. 1976 Jul;175(1):31–38. doi: 10.1016/0003-9861(76)90482-3. [DOI] [PubMed] [Google Scholar]
- MITCHELL P., MOYLE J. M. Paths of phosphate transfer in Micrococcus pyogenes: phosphate turnover in nucleic acids and other fractions. J Gen Microbiol. 1953 Oct;9(2):257–272. doi: 10.1099/00221287-9-2-257. [DOI] [PubMed] [Google Scholar]
- MITCHELL P. Transport of phosphate across the osmotic barrier of Micrococcus pyogenes; specificity and kinetics. J Gen Microbiol. 1954 Aug;11(1):73–82. doi: 10.1099/00221287-11-1-73. [DOI] [PubMed] [Google Scholar]
- Maloney P. C., Hansen F. C., 3rd Stoichiometry of proton movements coupled to ATP synthesis driven by a pH gradient in Streptococcus lactis. J Membr Biol. 1982;66(1):63–75. doi: 10.1007/BF01868482. [DOI] [PubMed] [Google Scholar]
- Maloney P. C. Membrane H+ conductance of Streptococcus lactis. J Bacteriol. 1979 Oct;140(1):197–205. doi: 10.1128/jb.140.1.197-205.1979. [DOI] [PMC free article] [PubMed] [Google Scholar]
- Maloney P. C. Relationship between phosphorylation potential and electrochemical H+ gradient during glycolysis in Streptococcus lactis. J Bacteriol. 1983 Mar;153(3):1461–1470. doi: 10.1128/jb.153.3.1461-1470.1983. [DOI] [PMC free article] [PubMed] [Google Scholar]
- McGivan J. D., Klingenberg M. Correlation between H+ and anion movement in mitochondria and the key role of the phosphate carrier. Eur J Biochem. 1971 Jun 11;20(3):392–399. doi: 10.1111/j.1432-1033.1971.tb01405.x. [DOI] [PubMed] [Google Scholar]
- Mitchell P. The Ninth Sir Hans Krebs Lecture. Compartmentation and communication in living systems. Ligand conduction: a general catalytic principle in chemical, osmotic and chemiosmotic reaction systems. Eur J Biochem. 1979 Mar 15;95(1):1–20. doi: 10.1111/j.1432-1033.1979.tb12934.x. [DOI] [PubMed] [Google Scholar]
- Reizer J., Novotny M. J., Panos C., Saier M. H., Jr Mechanism of inducer expulsion in Streptococcus pyogenes: a two-step process activated by ATP. J Bacteriol. 1983 Oct;156(1):354–361. doi: 10.1128/jb.156.1.354-361.1983. [DOI] [PMC free article] [PubMed] [Google Scholar]
- Reizer J., Saier M. H., Jr Involvement of lactose enzyme II of the phosphotransferase system in rapid expulsion of free galactosides from Streptococcus pyogenes. J Bacteriol. 1983 Oct;156(1):236–242. doi: 10.1128/jb.156.1.236-242.1983. [DOI] [PMC free article] [PubMed] [Google Scholar]
- Robillard G. T. The enzymology of the bacterial phosphoenolpyruvate-dependent sugar transport systems. Mol Cell Biochem. 1982 Jul 7;46(1):3–24. doi: 10.1007/BF00215577. [DOI] [PubMed] [Google Scholar]
- Rosenberg H., Gerdes R. G., Harold F. M. Energy coupling to the transport of inorganic phosphate in Escherichia coli K12. Biochem J. 1979 Jan 15;178(1):133–137. doi: 10.1042/bj1780133. [DOI] [PMC free article] [PubMed] [Google Scholar]
- Rosenberg H., Russell L. M., Jacomb P. A., Chegwidden K. Phosphate exchange in the pit transport system in Escherichia coli. J Bacteriol. 1982 Jan;149(1):123–130. doi: 10.1128/jb.149.1.123-130.1982. [DOI] [PMC free article] [PubMed] [Google Scholar]
- Rothstein A., Ramjeesingh M. The functional arrangement of the anion channel of red blood cells. Ann N Y Acad Sci. 1980;358:1–12. doi: 10.1111/j.1749-6632.1980.tb15381.x. [DOI] [PubMed] [Google Scholar]
- Saier M. H., Jr Bacterial phosphoenolpyruvate: sugar phosphotransferase systems: structural, functional, and evolutionary interrelationships. Bacteriol Rev. 1977 Dec;41(4):856–871. doi: 10.1128/br.41.4.856-871.1977. [DOI] [PMC free article] [PubMed] [Google Scholar]
- Saier M. H., Jr, Cox D. F., Moczydlowski E. G. Sugar phosphate:sugar transphosphorylation coupled to exchange group translocation catalyzed by the enzyme II complexes of the phosphoenolpyruvate:sugar phosphotransferase system in membrane vesicles of Escherichia coli. J Biol Chem. 1977 Dec 25;252(24):8908–8916. [PubMed] [Google Scholar]
- Thompson J., Chassy B. M. Intracellular hexose-6-phosphate:phosphohydrolase from Streptococcus lactis: purification, properties, and function. J Bacteriol. 1983 Oct;156(1):70–80. doi: 10.1128/jb.156.1.70-80.1983. [DOI] [PMC free article] [PubMed] [Google Scholar]
- Thompson J., Chassy B. M. Novel phosphoenolpyruvate-dependent futile cycle in Streptococcus lactis: 2-deoxy-D-glucose uncouples energy production from growth. J Bacteriol. 1982 Sep;151(3):1454–1465. doi: 10.1128/jb.151.3.1454-1465.1982. [DOI] [PMC free article] [PubMed] [Google Scholar]
- Thompson J. In vivo regulation of glycolysis and characterization of sugar: phosphotransferase systems in Streptococcus lactis. J Bacteriol. 1978 Nov;136(2):465–476. doi: 10.1128/jb.136.2.465-476.1978. [DOI] [PMC free article] [PubMed] [Google Scholar]
- Thompson J., Saier M. H., Jr Regulation of methyl-beta-d-thiogalactopyranoside-6-phosphate accumulation in Streptococcus lactis by exclusion and expulsion mechanisms. J Bacteriol. 1981 Jun;146(3):885–894. doi: 10.1128/jb.146.3.885-894.1981. [DOI] [PMC free article] [PubMed] [Google Scholar]
- Wehrle J. P., Pedersen P. L. Characteristics of phosphate uptake by Ehrlich ascites tumor cells. J Biol Chem. 1982 Aug 25;257(16):9698–9703. [PubMed] [Google Scholar]
- Willsky G. R., Malamy M. H. Characterization of two genetically separable inorganic phosphate transport systems in Escherichia coli. J Bacteriol. 1980 Oct;144(1):356–365. doi: 10.1128/jb.144.1.356-365.1980. [DOI] [PMC free article] [PubMed] [Google Scholar]
- Winkler H. H. Rickettsial permeability. An ADP-ATP transport system. J Biol Chem. 1976 Jan 25;251(2):389–396. [PubMed] [Google Scholar]