Abstract
Sulfate transport capacity was not regulated by cysteine, methionine, or glutathione in Pseudomonas halodurans, but growth on sulfate or thiosulfate suppressed transport. Subsequent sulfur starvation of cultures grown on all sulfur sources except glutathione stimulated uptake. Only methionine failed to regulate sulfate transport in Alteromonas luteo-violaceus, and sulfur starvation of all cultures enhanced transport capacity. During sulfur starvation of sulfate-grown cultures of both bacteria, the increase in transport capacity was mirrored by a decrease in the low-molecular-weight organic sulfur pool. Little metabolism of endogenous inorganic sulfate occurred. Cysteine was probably the major regulatory compound in A. luteo-violaceus, but an intermediate in sulfate reduction, between sulfate and cysteine, controlled sulfate transport in P. halodurans. Kinetic characteristics of sulfate transport in the marine bacteria were similar to those of previously reported nonmarine systems in spite of significant regulatory differences. Sulfate and thiosulfate uptake in P. halodurans responded identically to inhibitors, were coordinately regulated by growth on various sulfur compounds and sulfur starvation, and were mutually competitive inhibitors of transport, suggesting that they were transported by the same mechanism. The affinity of P. halodurans for thiosulfate was much greater than for sulfate.
Full text
PDF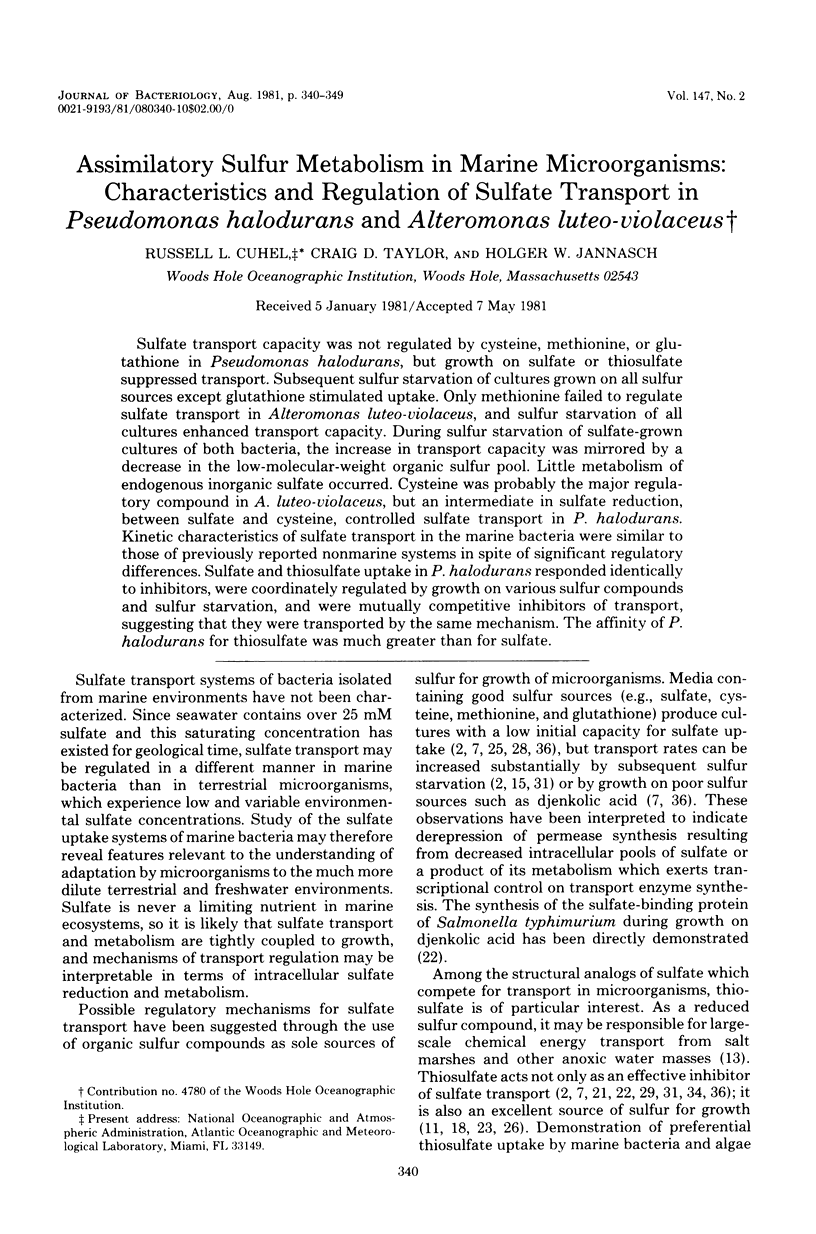
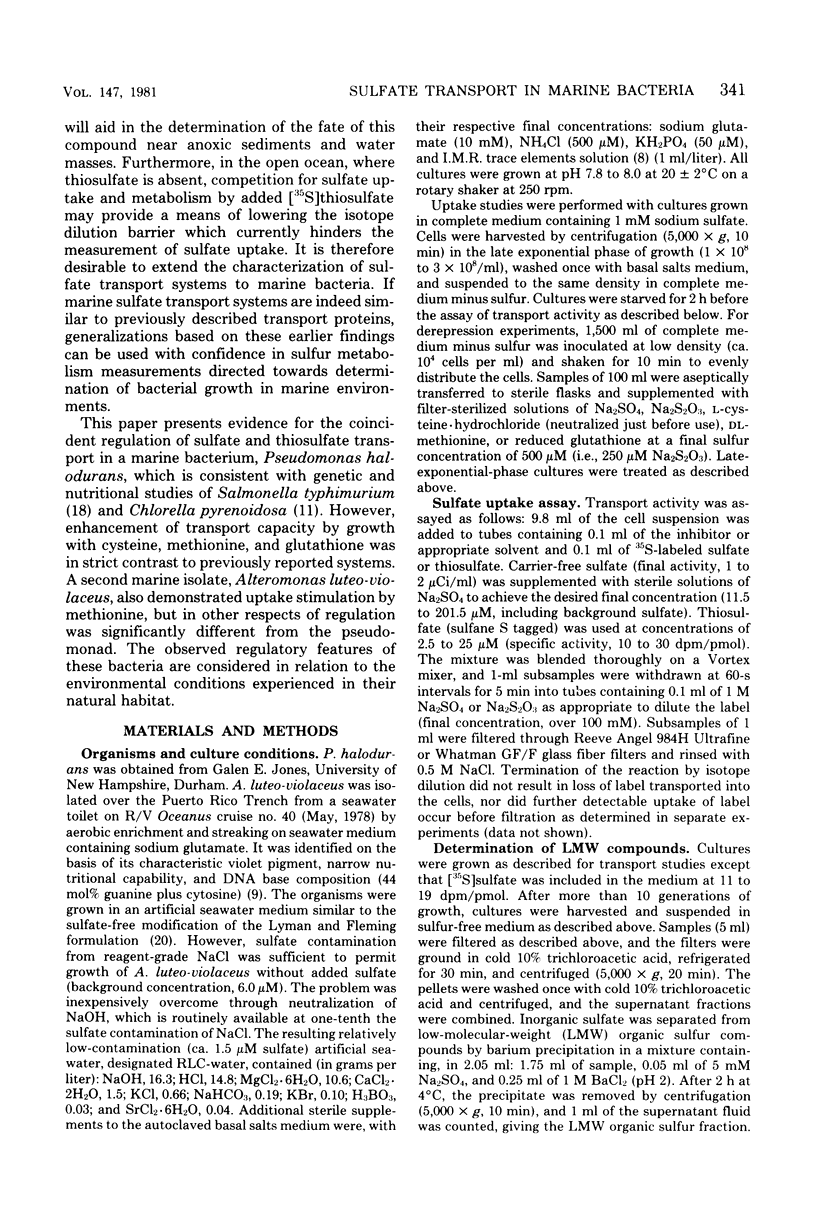
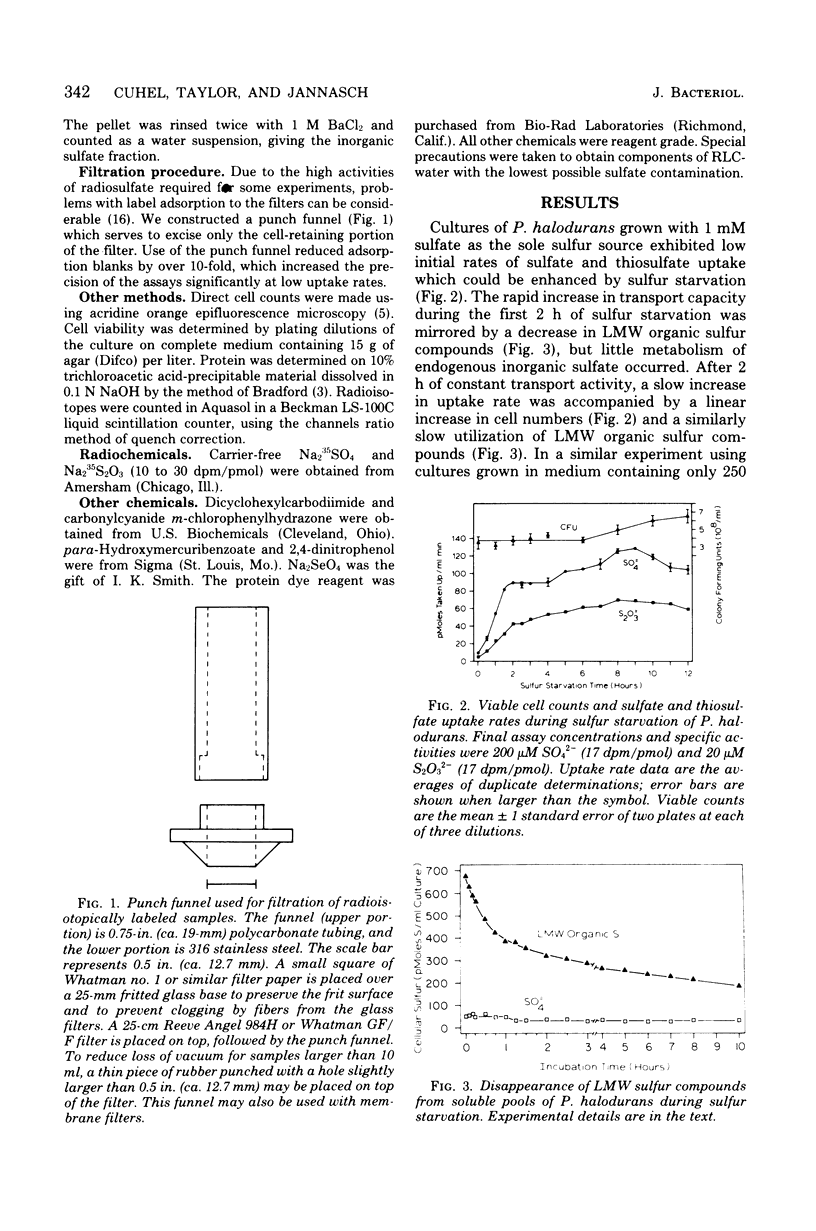
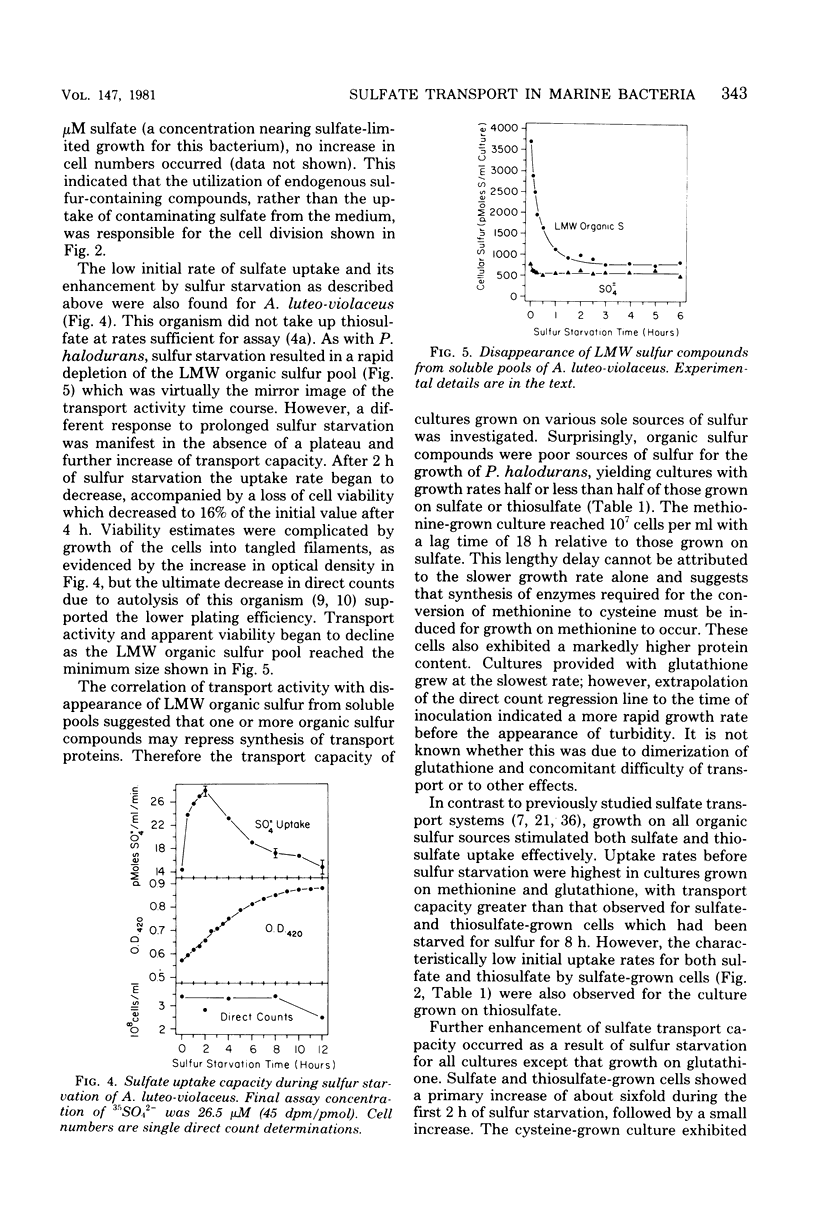
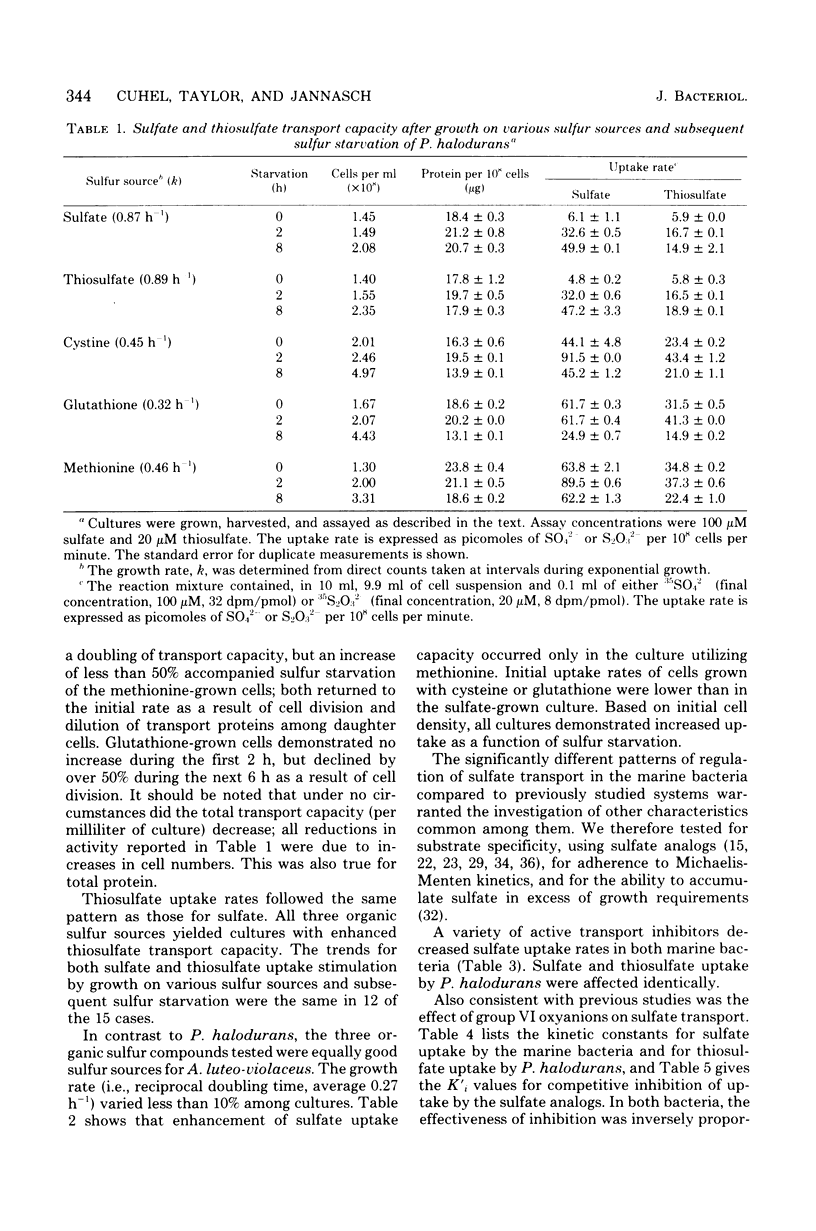
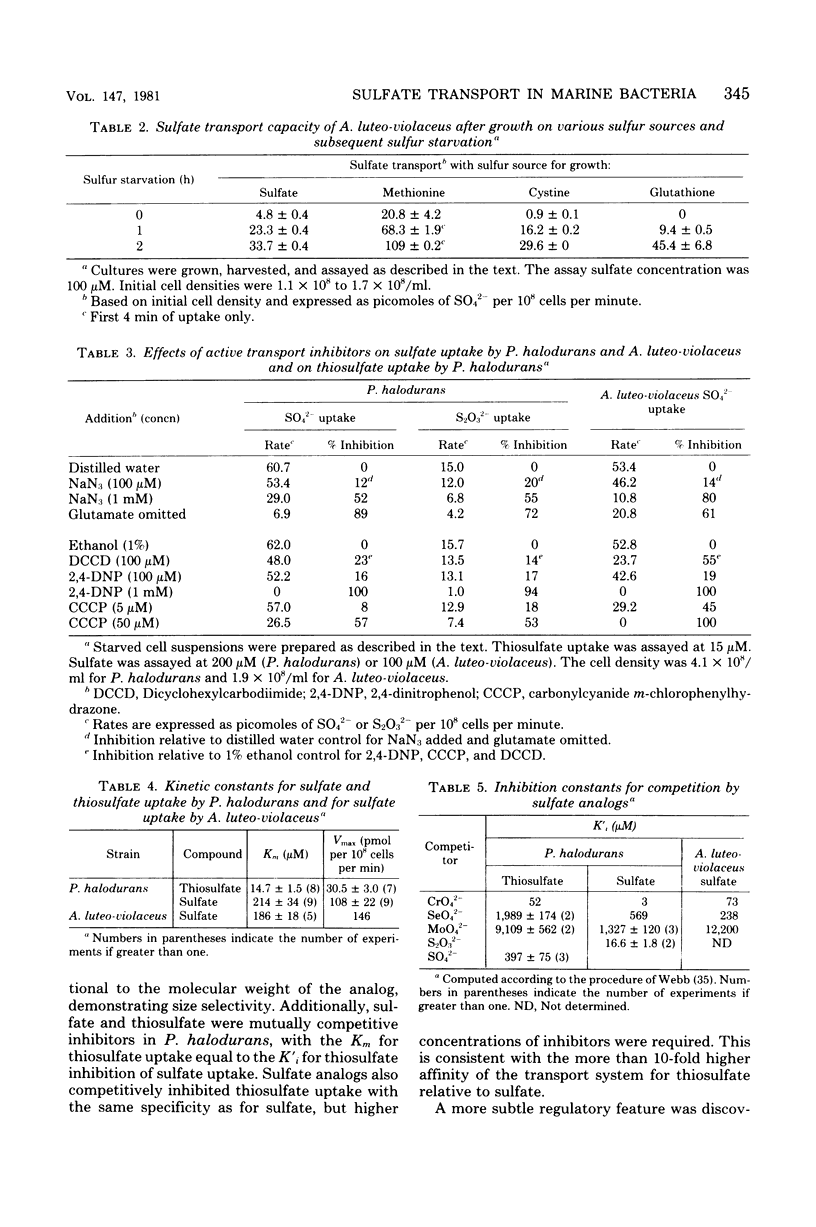
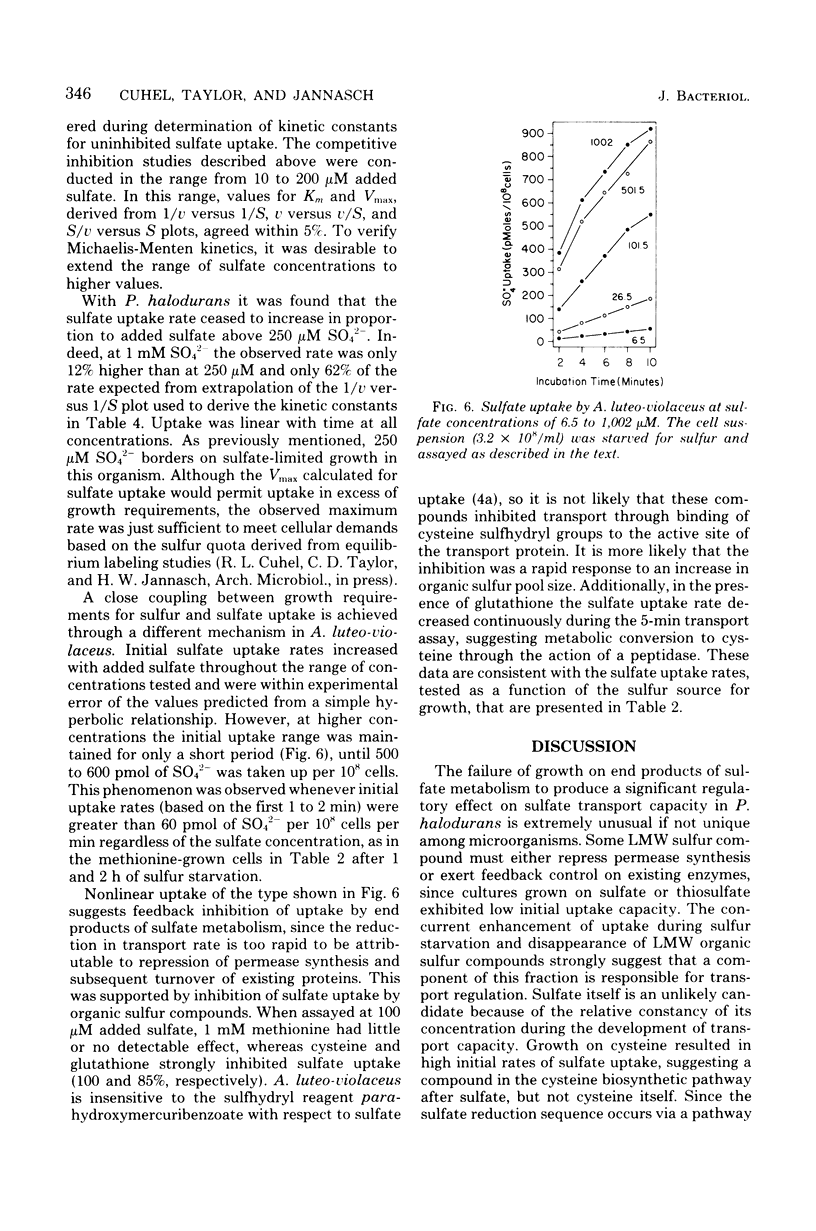
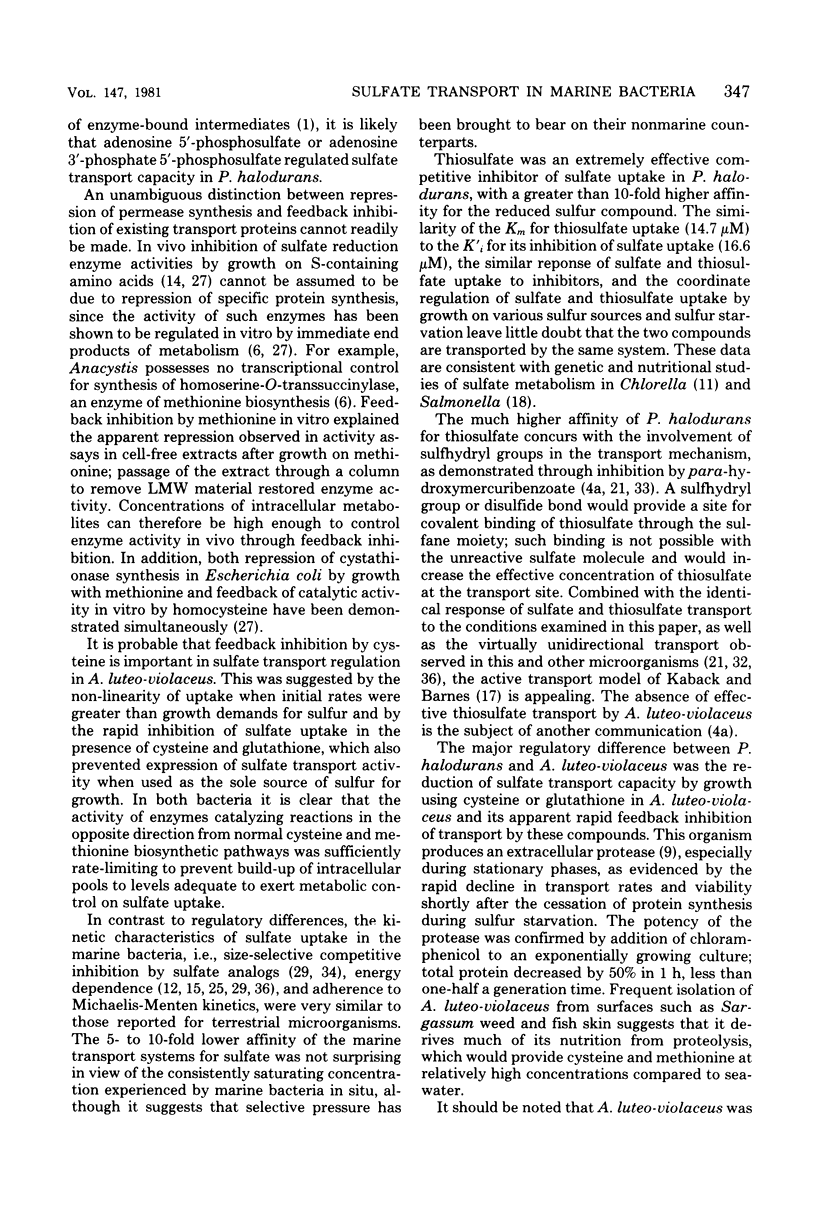
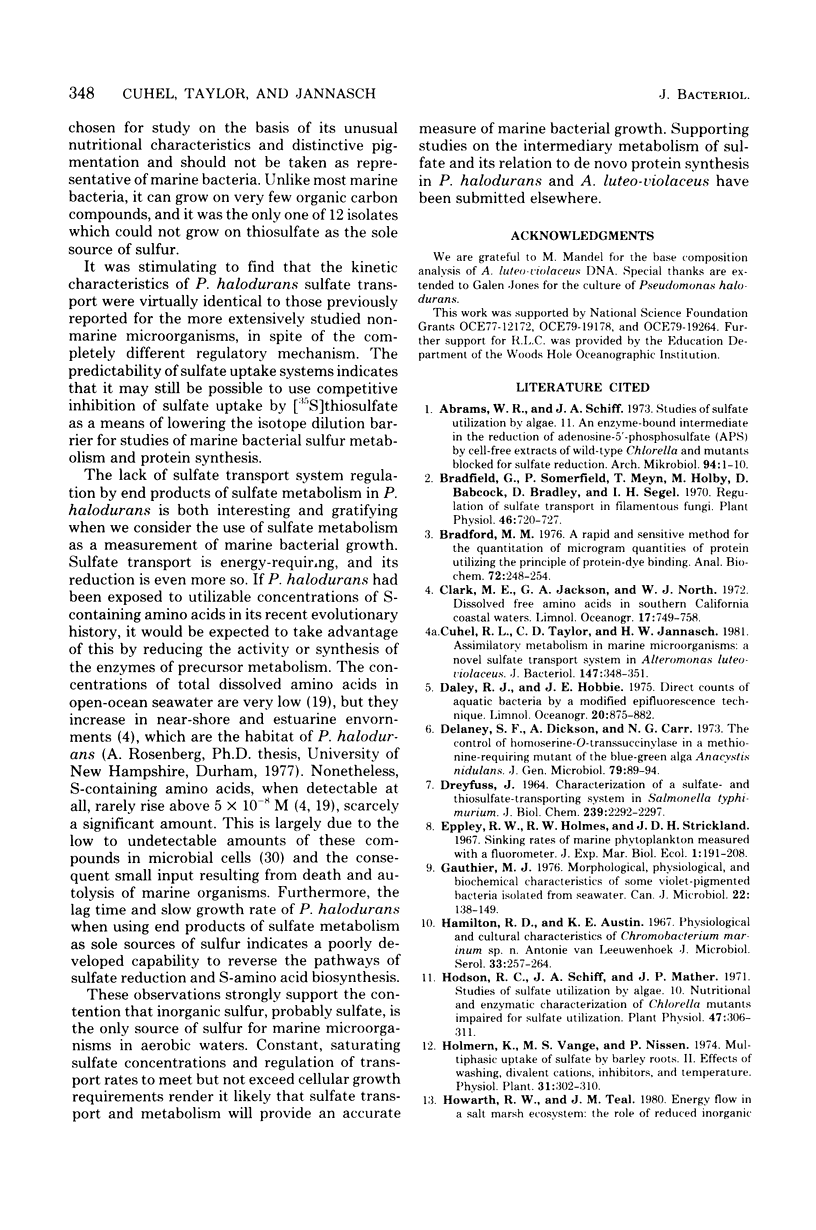
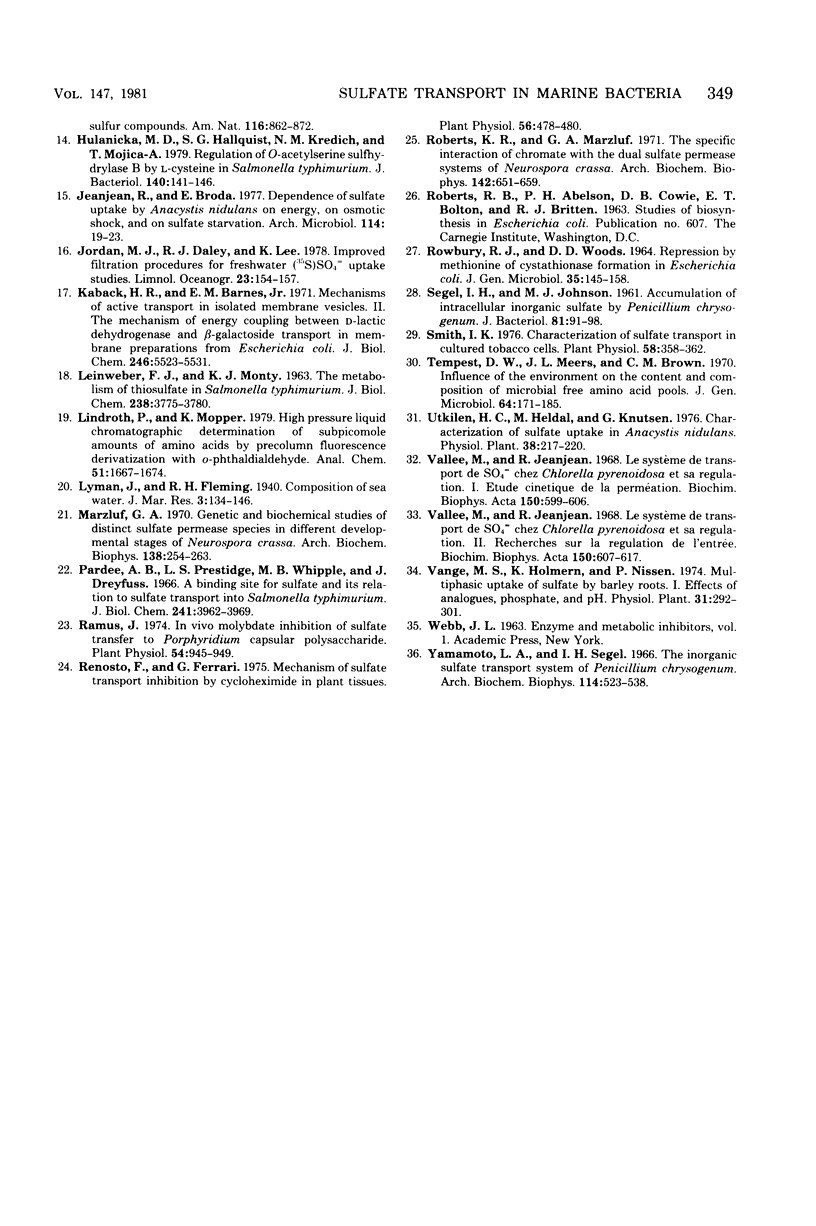
Selected References
These references are in PubMed. This may not be the complete list of references from this article.
- Abrams W. R., Schiff J. A. Studies of sulfate utilization by algae. II. An enzyme-bound intermediate in the reduction of adenosine-5'-phosphosulfate (APS) by cell-free extracts of wild-type Chlorella and mutants blocked for sulfate reduction. Arch Mikrobiol. 1973 Dec 4;94(1):1–10. [PubMed] [Google Scholar]
- Bradfield G., Somerfield P., Meyn T., Holby M., Babcock D., Bradley D., Segel I. H. Regulation of sulfate transport in filamentous fungi. Plant Physiol. 1970 Nov;46(5):720–727. doi: 10.1104/pp.46.5.720. [DOI] [PMC free article] [PubMed] [Google Scholar]
- Bradford M. M. A rapid and sensitive method for the quantitation of microgram quantities of protein utilizing the principle of protein-dye binding. Anal Biochem. 1976 May 7;72:248–254. doi: 10.1006/abio.1976.9999. [DOI] [PubMed] [Google Scholar]
- DREYFUSS J. CHARACTERIZATION OF A SULFATE- AND THIOSULFATE-TRANSPORTING SYSTEM IN SALMONELLA TYPHIMURIUM. J Biol Chem. 1964 Jul;239:2292–2297. [PubMed] [Google Scholar]
- Gauthier M. J. Morphological, physiological, and biochemical characteristics of some violet-pigmented bacteria isolated from seawater. Can J Microbiol. 1976 Feb;22(2):138–149. doi: 10.1139/m76-019. [DOI] [PubMed] [Google Scholar]
- Hamilton R. D., Austin K. E. Physiological and cultural characteristics of Chromobacterium marinum sp. n. Antonie Van Leeuwenhoek. 1967;33(3):257–264. doi: 10.1007/BF02045571. [DOI] [PubMed] [Google Scholar]
- Hodson R. C., Schiff J. A., Mather J. P. Studies of sulfate utilization by algae: 10. Nutritional and enzymatic characterization of chlorella mutants impaired for sulfate utilization. Plant Physiol. 1971 Feb;47(2):306–311. doi: 10.1104/pp.47.2.306. [DOI] [PMC free article] [PubMed] [Google Scholar]
- Hulanicka M. D., Hallquist S. G., Kredich N. M., Mojica-A T. Regulation of O-acetylserine sulfhydrylase B by L-cysteine in Salmonella typhimurium. J Bacteriol. 1979 Oct;140(1):141–146. doi: 10.1128/jb.140.1.141-146.1979. [DOI] [PMC free article] [PubMed] [Google Scholar]
- Jeanjean R., Broda E. Dependence of sulphate uptake by Anacystis nidulans on energy, on osmotic shock and on sulphate stravation. Arch Microbiol. 1977 Jul 26;114(1):19–23. doi: 10.1007/BF00429625. [DOI] [PubMed] [Google Scholar]
- Kaback H. R., Barnes E. M., Jr Mechanisms of active transport in isolated membrane vesicles. II. The mechanism of energy coupling between D-lactic dehydrogenase and beta-galactoside transport in membrane preparations from Escherichia coli. J Biol Chem. 1971 Sep 10;246(17):5523–5531. [PubMed] [Google Scholar]
- LEINWEBER F. J., MONTY K. J. THE METABOLISM OF THIOSULFATE IN SALMONELLA TYPHIMURIUM. J Biol Chem. 1963 Nov;238:3775–3780. [PubMed] [Google Scholar]
- Marzluf G. A. Genetic and biochemical studies of distinct sulfate permease species in different developmental stages of Neurospora crassa. Arch Biochem Biophys. 1970 May;138(1):254–263. doi: 10.1016/0003-9861(70)90306-1. [DOI] [PubMed] [Google Scholar]
- Pardee A. B., Prestidge L. S., Whipple M. B., Dreyfuss J. A binding site for sulfate and its relation to sulfate transport into Salmonella typhimurium. J Biol Chem. 1966 Sep 10;241(17):3962–3969. [PubMed] [Google Scholar]
- ROWBURY R. J., WOODS D. D. REPRESSION BY METHIONINE OF CYSTATHIONASE FORMATION IN ESCHERICHIA COLI. J Gen Microbiol. 1964 Apr;35:145–158. doi: 10.1099/00221287-35-1-145. [DOI] [PubMed] [Google Scholar]
- Ramus J. In vivo molybdate inhibition of sulfate transfer to porphyridium capsular polysaccharide. Plant Physiol. 1974 Dec;54(6):945–949. doi: 10.1104/pp.54.6.945. [DOI] [PMC free article] [PubMed] [Google Scholar]
- Renosto F., Ferrari G. Mechanism of sulfate transport inhibition by cycloheximide in plant tissues. Plant Physiol. 1975 Oct;56(4):478–480. doi: 10.1104/pp.56.4.478. [DOI] [PMC free article] [PubMed] [Google Scholar]
- Roberts K. R., Marzluf G. A. The specific interaction of chromate with the dual sulfate permease systems of Neurospora crassa. Arch Biochem Biophys. 1971 Feb;142(2):651–659. doi: 10.1016/0003-9861(71)90531-5. [DOI] [PubMed] [Google Scholar]
- SEGEL I. H., JOHNSON M. J. Accumulation of intracellular inorganic sulfate by Penicillium chrysogenum. J Bacteriol. 1961 Jan;81:91–98. doi: 10.1128/jb.81.1.91-98.1961. [DOI] [PMC free article] [PubMed] [Google Scholar]
- Smith I. K. Characterization of sulfate transport in cultured tobacco cells. Plant Physiol. 1976 Sep;58(3):358–362. doi: 10.1104/pp.58.3.358. [DOI] [PMC free article] [PubMed] [Google Scholar]
- Tempest D. W., Meers J. L., Brown C. M. Influence of environment on the content and composition of microbial free amino acid pools. J Gen Microbiol. 1970 Dec;64(2):171–185. doi: 10.1099/00221287-64-2-171. [DOI] [PubMed] [Google Scholar]
- Vallée M., Jeanjean R. Le système de transport de SO42--chez Chlorella pyrenoidosa et sa régulation. II. Recherches sur la régulation de l'entrée. Biochim Biophys Acta. 1968 Jun 11;150(4):607–617. doi: 10.1016/0005-2736(68)90050-3. [DOI] [PubMed] [Google Scholar]
- Vallée M., Jeanjean R. Le système de transport de SO4= chez Chlorella pyrenoidosa et sa régulation. I. Etude cinétique de la perméation. Biochim Biophys Acta. 1968 Jun 11;150(4):599–606. doi: 10.1016/0005-2736(68)90049-7. [DOI] [PubMed] [Google Scholar]
- Yamamoto L. A., Segel I. H. The inorganic sulfate transport system of Penicillium chrysogenum. Arch Biochem Biophys. 1966 Jun;114(3):523–538. doi: 10.1016/0003-9861(66)90376-6. [DOI] [PubMed] [Google Scholar]