Abstract
Changes in dilution rate did not elicit large and systematic changes in cellular cyclic AMP levels in Escherichia coli grown in a chemostat under carbon or phosphate limitation. However, the technical difficulties of measuring low levels of cellular cyclic AMP in the presence of a large background of extracellular cyclic AMP precluded firm conclusions in this point. The net rate of cyclic AMP synthesis increased exponentially with increasing dilution rate through either the entire range of dilution rates examined (phosphate limitation) or a substantial part of the range (lactose and glucose limitations). Thus, it is probable that growth rate regulates the synthesis of adenylate cyclase. The maximum rate of net cyclic AMP synthesis was greater under lactose than under glucose limitation, which is consistent with the notion that the uptake of phosphotransferase sugars is more inhibitory to adenylate cyclase than the uptake of other carbon substrates. Phosphate-limited cultures exhibited the lowest rate of net cyclic AMP synthesis, which could be due to the role of phosphorylated metabolites in the regulation of adenylate cyclase activity. Under all growth conditions examined, greater than 99.9% of the cyclic AMP synthesized was found in the culture medium. The function of this excretion, which consumed up to 9% of the total energy available to the cell and which evidently resulted from elaborate regulatory mechanisms, remains entirely unknown.
Full text
PDF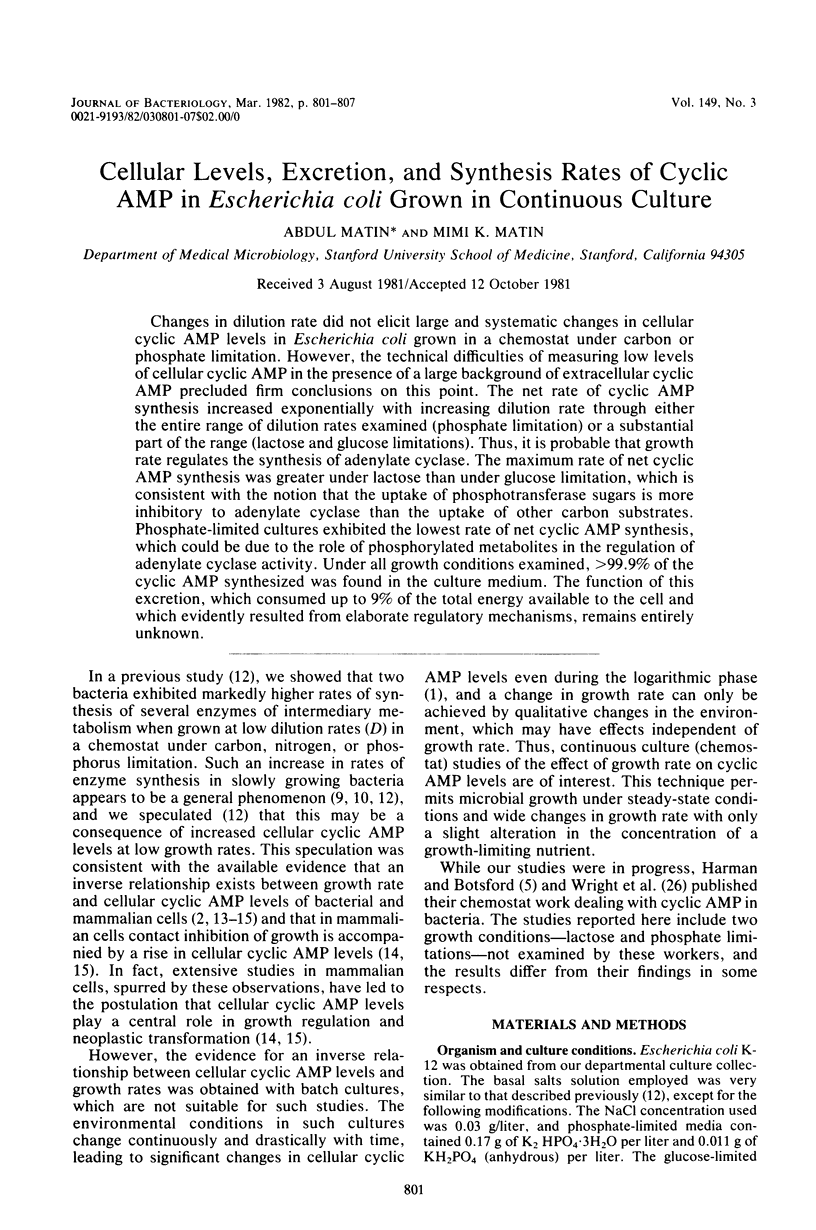
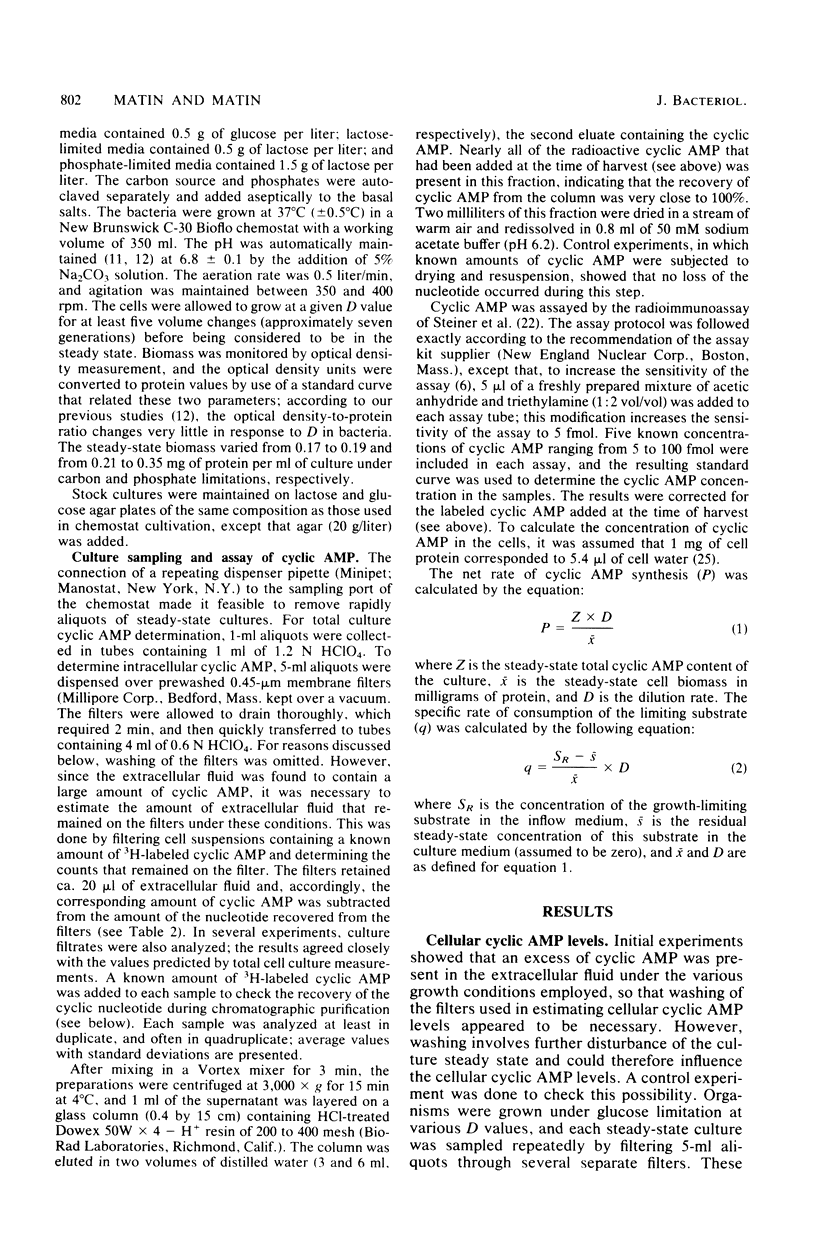
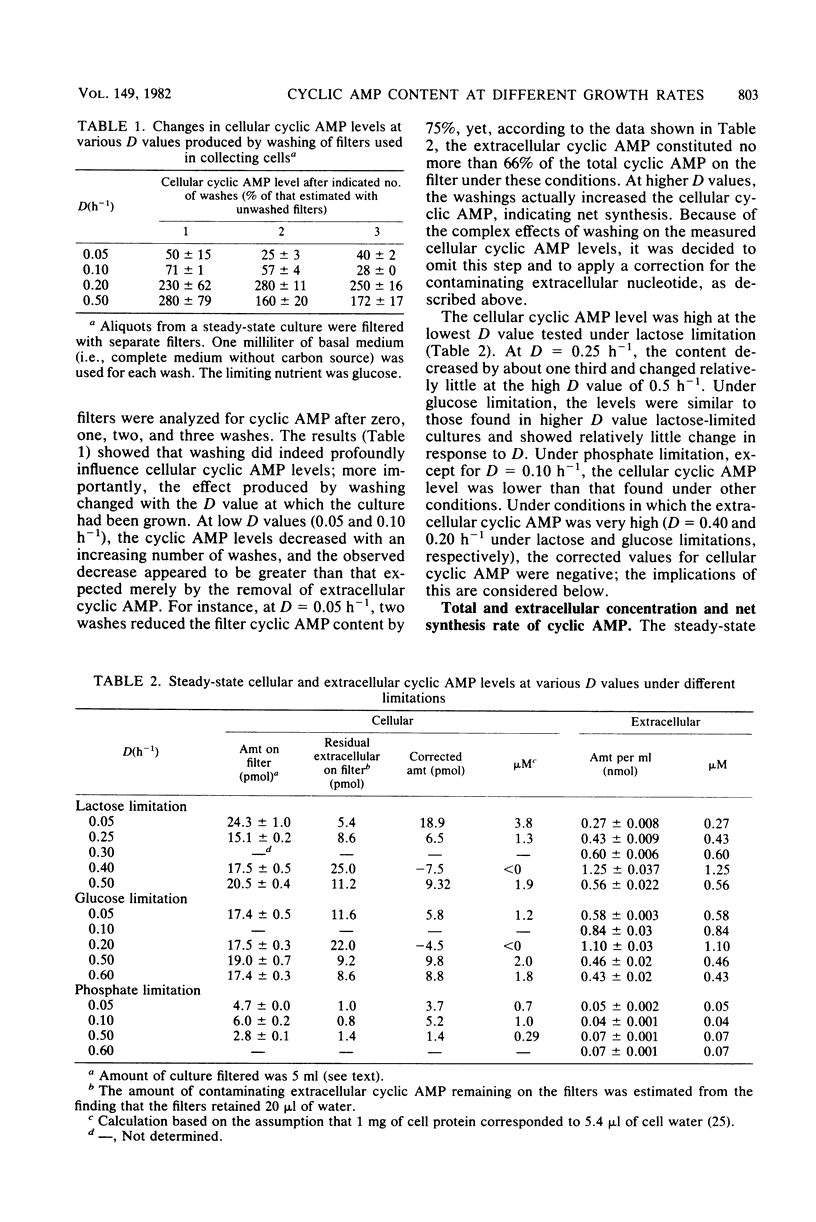
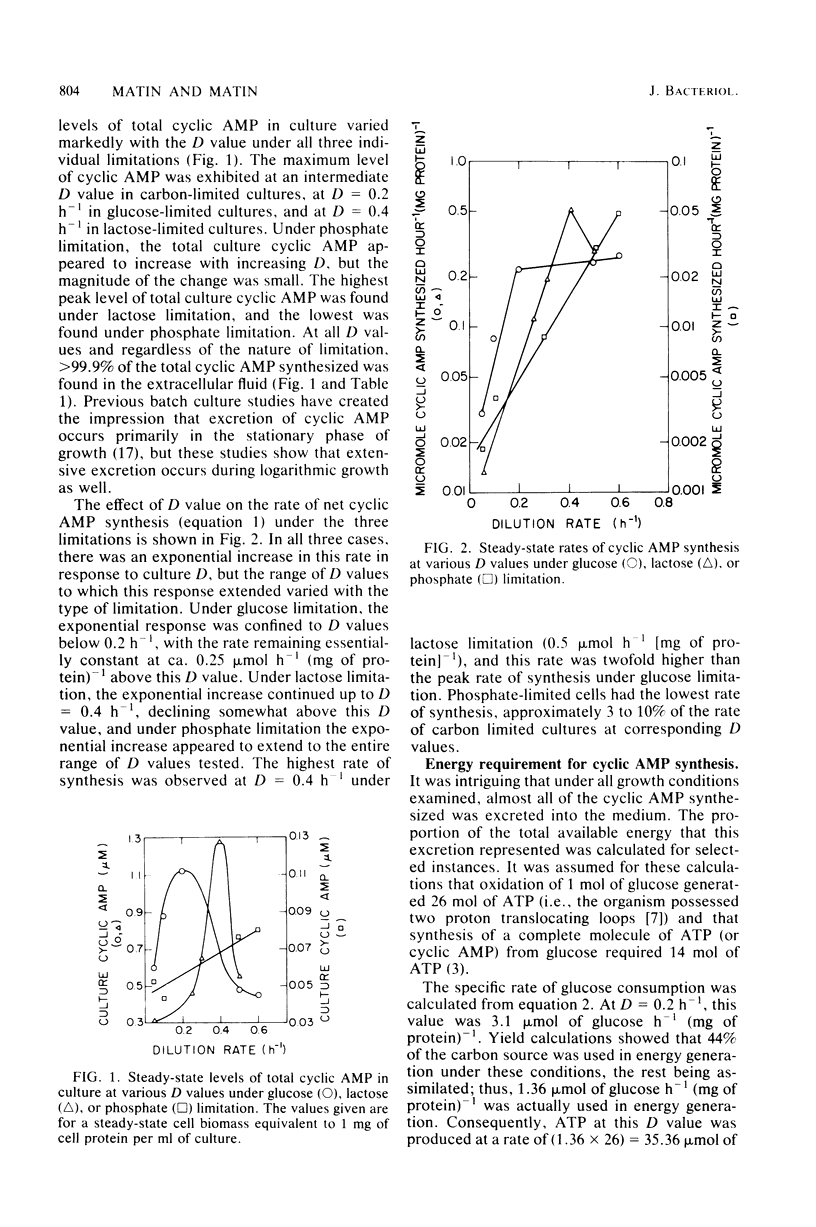
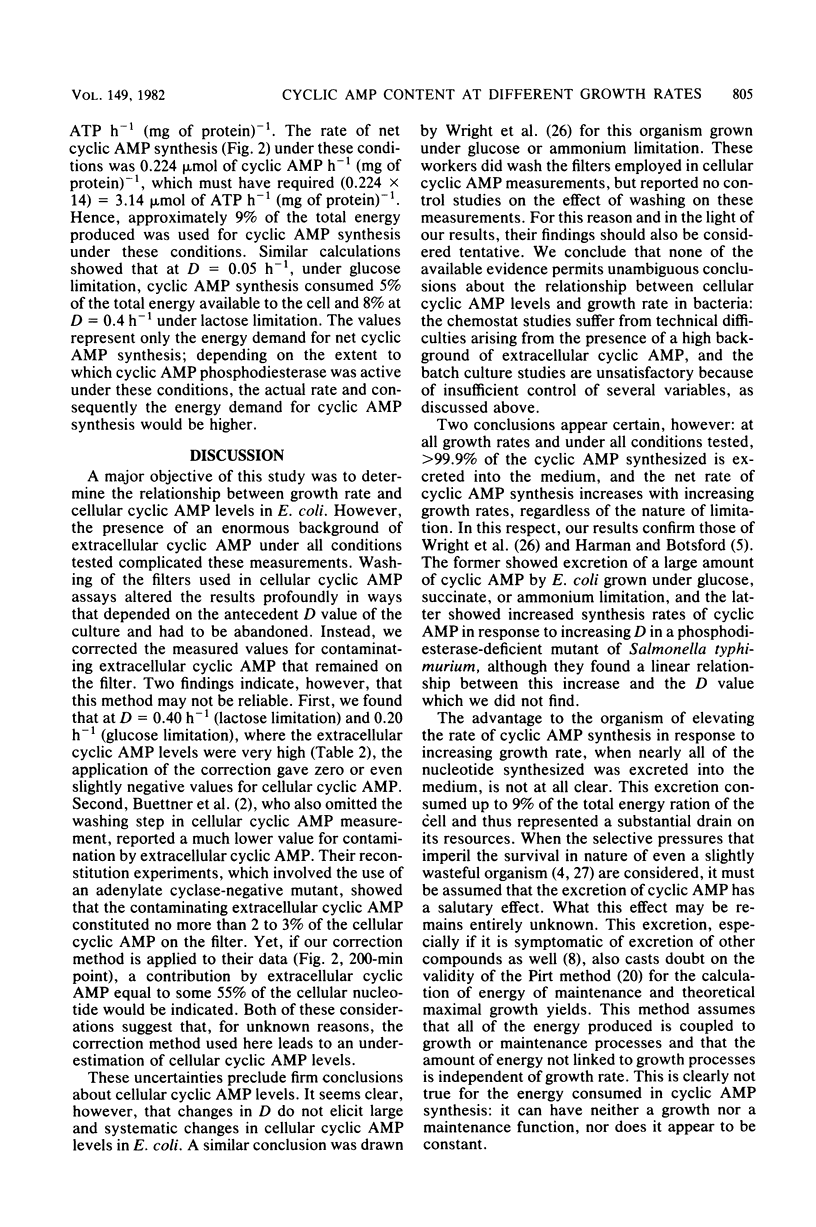
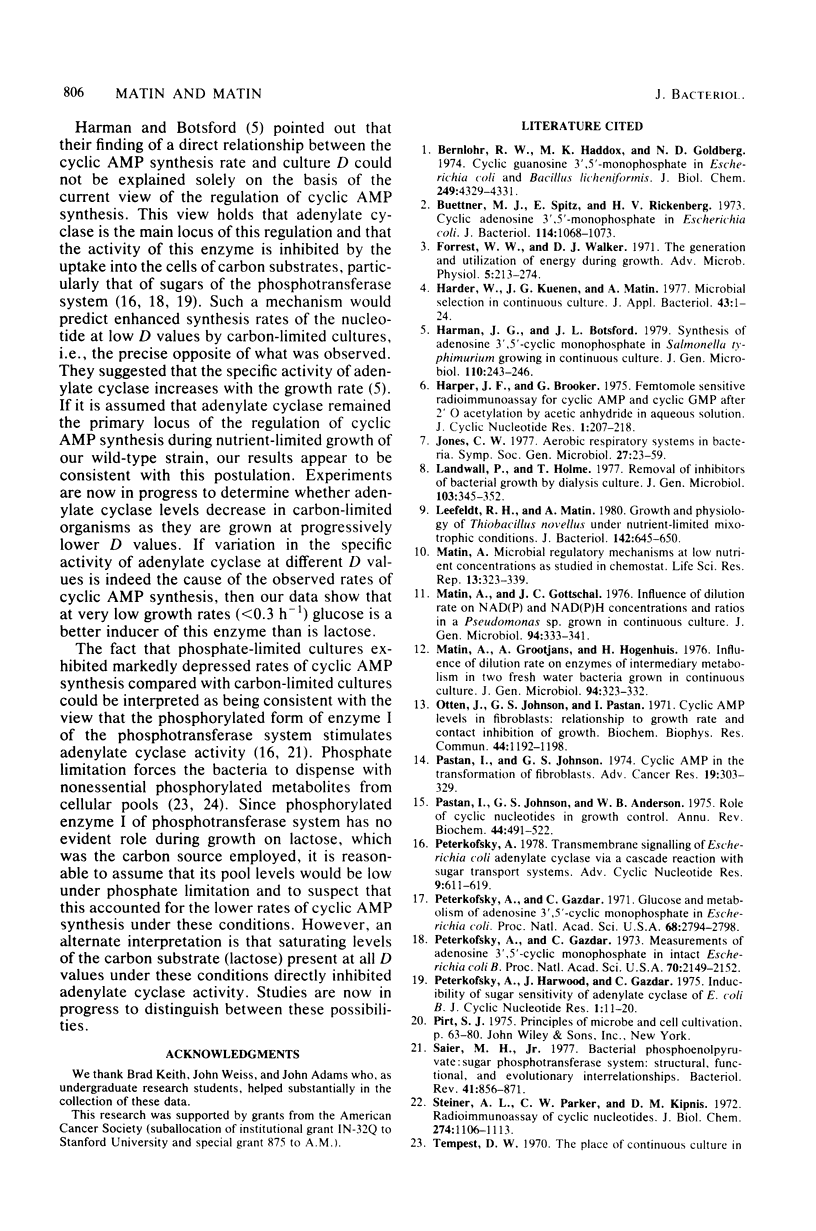
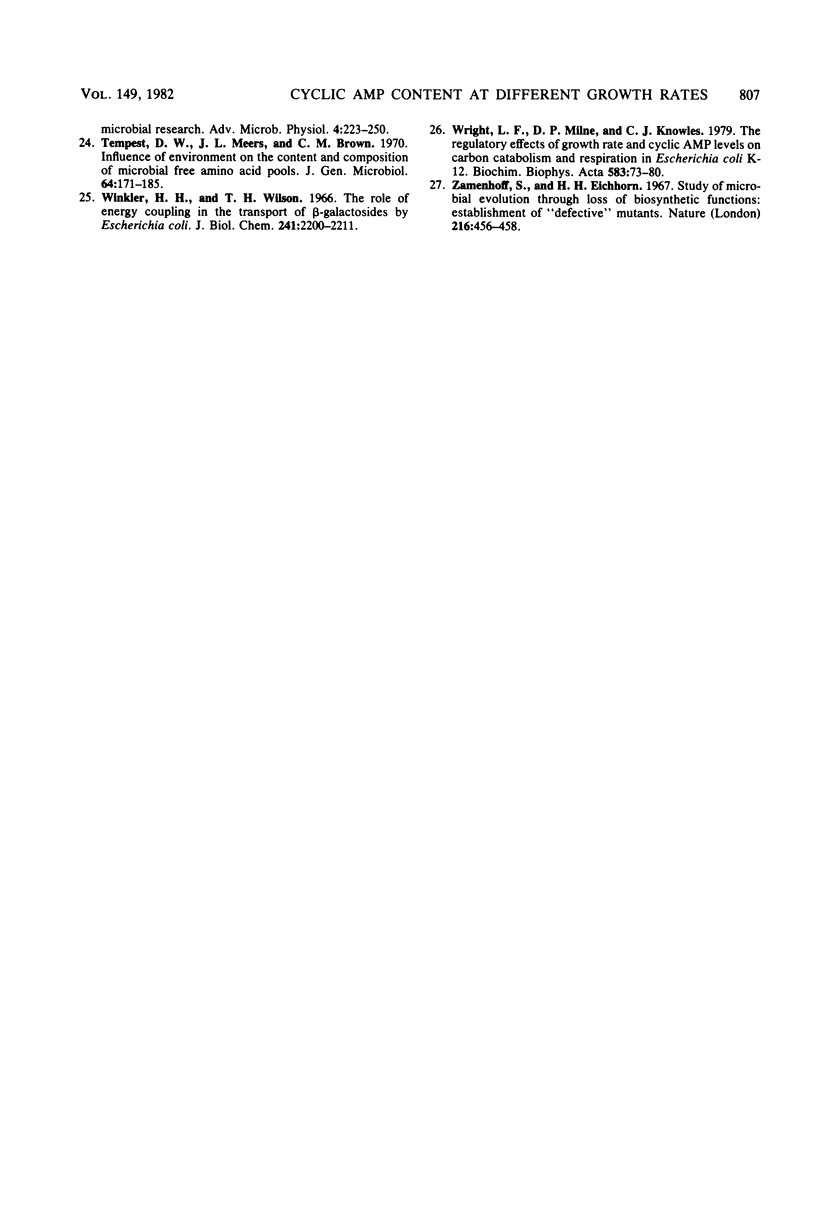
Selected References
These references are in PubMed. This may not be the complete list of references from this article.
- Bernlohr R. W., Haddox M. K., Goldberg N. D. Cyclic guanosine 3':5'-monophosphate in Escherichia coli and Bacillus lichenformis. J Biol Chem. 1974 Jul 10;249(13):4329–4331. [PubMed] [Google Scholar]
- Buettner M. J., Spitz E., Rickenberg H. V. Cyclic adenosine 3',5'-monophosphate in Escherichia coli. J Bacteriol. 1973 Jun;114(3):1068–1073. doi: 10.1128/jb.114.3.1068-1073.1973. [DOI] [PMC free article] [PubMed] [Google Scholar]
- Forrest W. W., Walker D. J. The generation and utilization of energy during growth. Adv Microb Physiol. 1971;5:213–274. doi: 10.1016/s0065-2911(08)60408-7. [DOI] [PubMed] [Google Scholar]
- Harder W., Kuenen J. G. A review. Microbial selection in continuous culture. J Appl Bacteriol. 1977 Aug;43(1):1–24. doi: 10.1111/j.1365-2672.1977.tb00717.x. [DOI] [PubMed] [Google Scholar]
- Harman J. G., Botsford J. L. Synthesis of adenosine 3':5'-cyclic monophosphate in Salmonella typhimurium growing in continuous culture. J Gen Microbiol. 1979 Jan;110(1):243–246. doi: 10.1099/00221287-110-1-243. [DOI] [PubMed] [Google Scholar]
- Harper J. F., Brooker G. Femtomole sensitive radioimmunoassay for cyclic AMP and cyclic GMP after 2'0 acetylation by acetic anhydride in aqueous solution. J Cyclic Nucleotide Res. 1975;1(4):207–218. [PubMed] [Google Scholar]
- Landwall P., Holme T. Removal of inhibitors of bacterial growth by dialysis culture. J Gen Microbiol. 1977 Dec;103(2):345–352. doi: 10.1099/00221287-103-2-345. [DOI] [PubMed] [Google Scholar]
- Leefeldt R. H., Matin A. Growth and physiology of Thiobacillus novellus under nutrient-limited mixotrophic conditions. J Bacteriol. 1980 May;142(2):645–650. doi: 10.1128/jb.142.2.645-650.1980. [DOI] [PMC free article] [PubMed] [Google Scholar]
- Matin A., Gottschal J. C. Influence of dilution rate on NAD(P) and NAD(P)H concentrations and ratios in a Pseudomonas sp. grown in continuous culture. J Gen Microbiol. 1976 Jun;94(2):333–341. doi: 10.1099/00221287-94-2-333. [DOI] [PubMed] [Google Scholar]
- Matin A., Grootjans A., Hogenhuis H. Influence of dilution rate on enzymes of intermediary metabolism in two freshwater bacteria grown in continuous culture. J Gen Microbiol. 1976 Jun;94(2):323–332. doi: 10.1099/00221287-94-2-323. [DOI] [PubMed] [Google Scholar]
- Otten J., Johnson G. S., Pastan I. Cyclic AMP levels in fibroblasts: relationship to growth rate and contact inhibition of growth. Biochem Biophys Res Commun. 1971 Sep;44(5):1192–1198. doi: 10.1016/s0006-291x(71)80212-7. [DOI] [PubMed] [Google Scholar]
- Pastan I. H., Johnson G. S., Anderson W. B. Role of cyclic nucleotides in growth control. Annu Rev Biochem. 1975;44:491–522. doi: 10.1146/annurev.bi.44.070175.002423. [DOI] [PubMed] [Google Scholar]
- Pastan I., Johnson G. S. Cyclic AMP and the transformation of fibroblasts. Adv Cancer Res. 1974;19(0):303–329. doi: 10.1016/s0065-230x(08)60057-3. [DOI] [PubMed] [Google Scholar]
- Peterkofsky A., Gazdar C. Glucose and the metabolism of adenosine 3':5'-cyclic monophosphate in Escherichia coli. Proc Natl Acad Sci U S A. 1971 Nov;68(11):2794–2798. doi: 10.1073/pnas.68.11.2794. [DOI] [PMC free article] [PubMed] [Google Scholar]
- Peterkofsky A., Gazdar C. Measurements of rates of adenosine 3':5'-cyclic monophosphate synthesis in intact Escherichia coli B. Proc Natl Acad Sci U S A. 1973 Jul;70(7):2149–2152. doi: 10.1073/pnas.70.7.2149. [DOI] [PMC free article] [PubMed] [Google Scholar]
- Peterkofsky A. Transmembrane signaling to Escherichia coli adenylate cyclase via a cascade reaction with sugar transport systems. Adv Cyclic Nucleotide Res. 1978;9:611–619. [PubMed] [Google Scholar]
- Saier M. H., Jr Bacterial phosphoenolpyruvate: sugar phosphotransferase systems: structural, functional, and evolutionary interrelationships. Bacteriol Rev. 1977 Dec;41(4):856–871. doi: 10.1128/br.41.4.856-871.1977. [DOI] [PMC free article] [PubMed] [Google Scholar]
- Steiner A. L., Parker C. W., Kipnis D. M. Radioimmunoassay for cyclic nucleotides. I. Preparation of antibodies and iodinated cyclic nucleotides. J Biol Chem. 1972 Feb 25;247(4):1106–1113. [PubMed] [Google Scholar]
- Tempest D. W., Meers J. L., Brown C. M. Influence of environment on the content and composition of microbial free amino acid pools. J Gen Microbiol. 1970 Dec;64(2):171–185. doi: 10.1099/00221287-64-2-171. [DOI] [PubMed] [Google Scholar]
- Winkler H. H., Wilson T. H. The role of energy coupling in the transport of beta-galactosides by Escherichia coli. J Biol Chem. 1966 May 25;241(10):2200–2211. [PubMed] [Google Scholar]
- Wright L. F., Milne D. P., Knowles C. J. The regulatory effects of growth rate and cyclic AMP levels on carbon catabolism and respiration in Escherichia coli K-12. Biochim Biophys Acta. 1979 Feb 19;583(1):73–80. doi: 10.1016/0304-4165(79)90311-8. [DOI] [PubMed] [Google Scholar]
- Zamenhof S., Eichhorn H. H. Study of microbial evolution through loss of biosynthetic functions: establishment of "defective" mutants. Nature. 1967 Nov 4;216(5114):456–458. doi: 10.1038/216456a0. [DOI] [PubMed] [Google Scholar]