Abstract
Total synthesis of acetate from CO2 by Clostridium acidiurici during fermentations of hypoxanthine has been shown to involve synthesis of glycine from methylenetetrahydrofolate, CO2, and NH3. The glycine is converted to serine by the addition of methylenetetrahydrofolate, and the resulting serine is converted to pyruvate, which is decarboxylated to form acetate. Since CO2 is converted to methylenetetrahydrofolate, both carbons of the acetate are derived from CO2. The evidence supporting this pathway is based on (i) the demonstration that glycine decarboxylase is present in C. acidiurici, (ii) the fact that glycine is synthesized by crude extracts at a rate which is rapid enough to account for the in vivo synthesis of acetate from CO2, (iii) the fact that methylenetetrahydrofolate is an intermediate in the formation of both carbons of acetate from CO2, and (iv) the fact that the alpha carbon of glycine is the source of the carboxyl group of acetate. Evidence is presented that this synthesis of acetate does not involve carboxylation of a methyl corrinoid enzyme such as occurs in Clostridium thermoaceticum and Clostridium formicoaceticum. Thus, there are two different mechanisms for the total synthesis of acetate from CO2 by clostridia.
Full text
PDF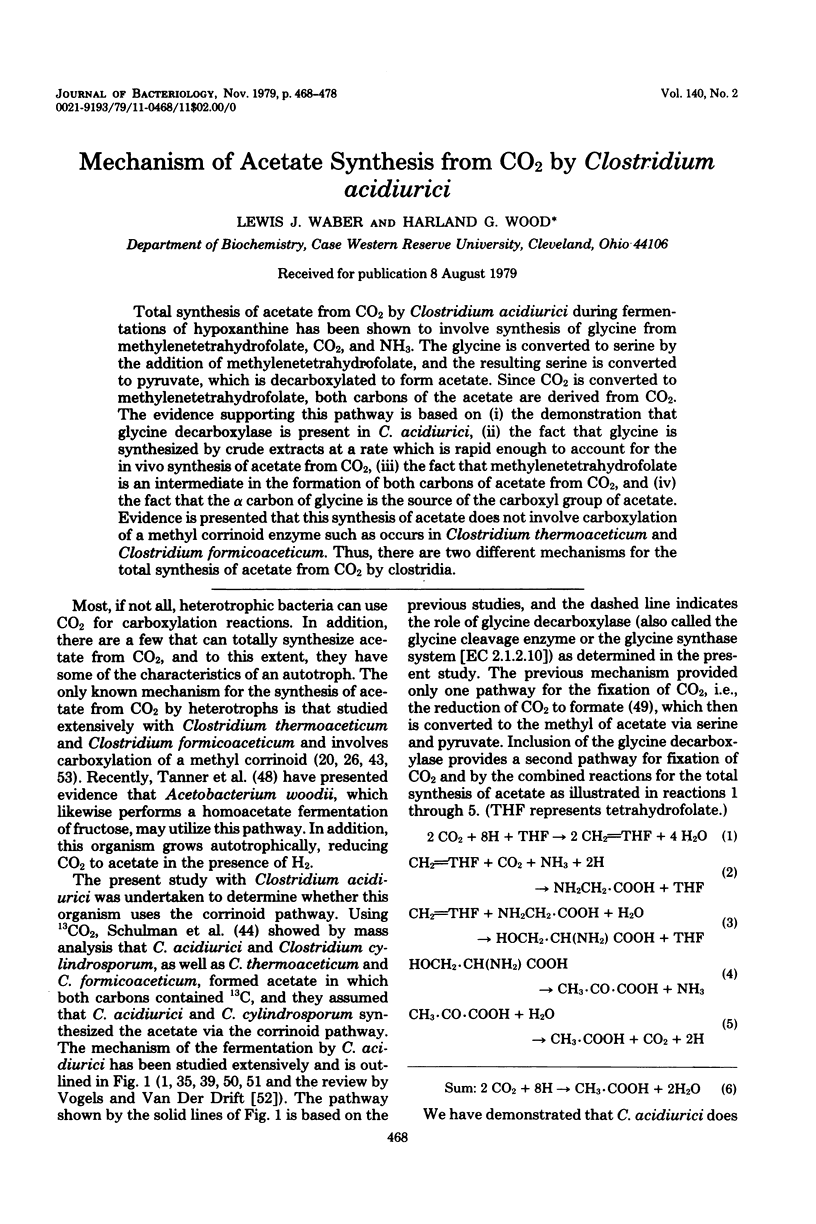
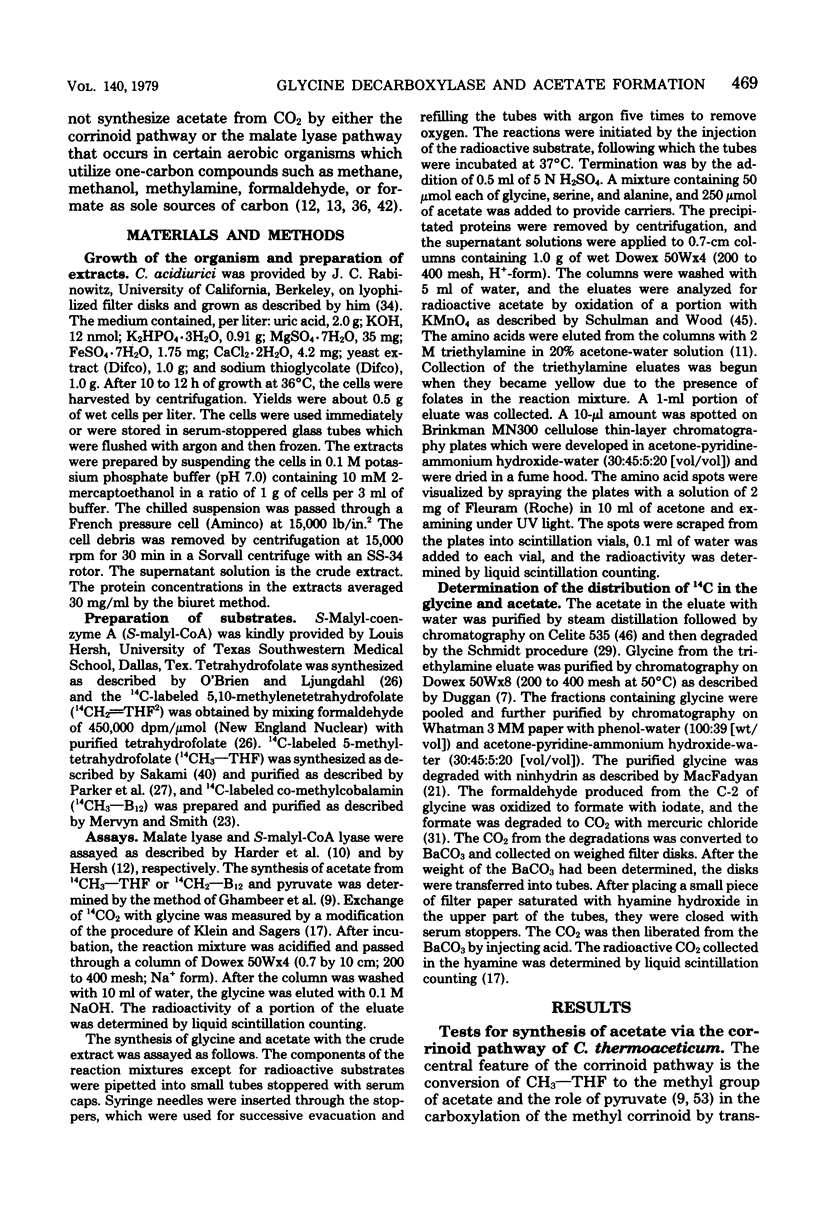
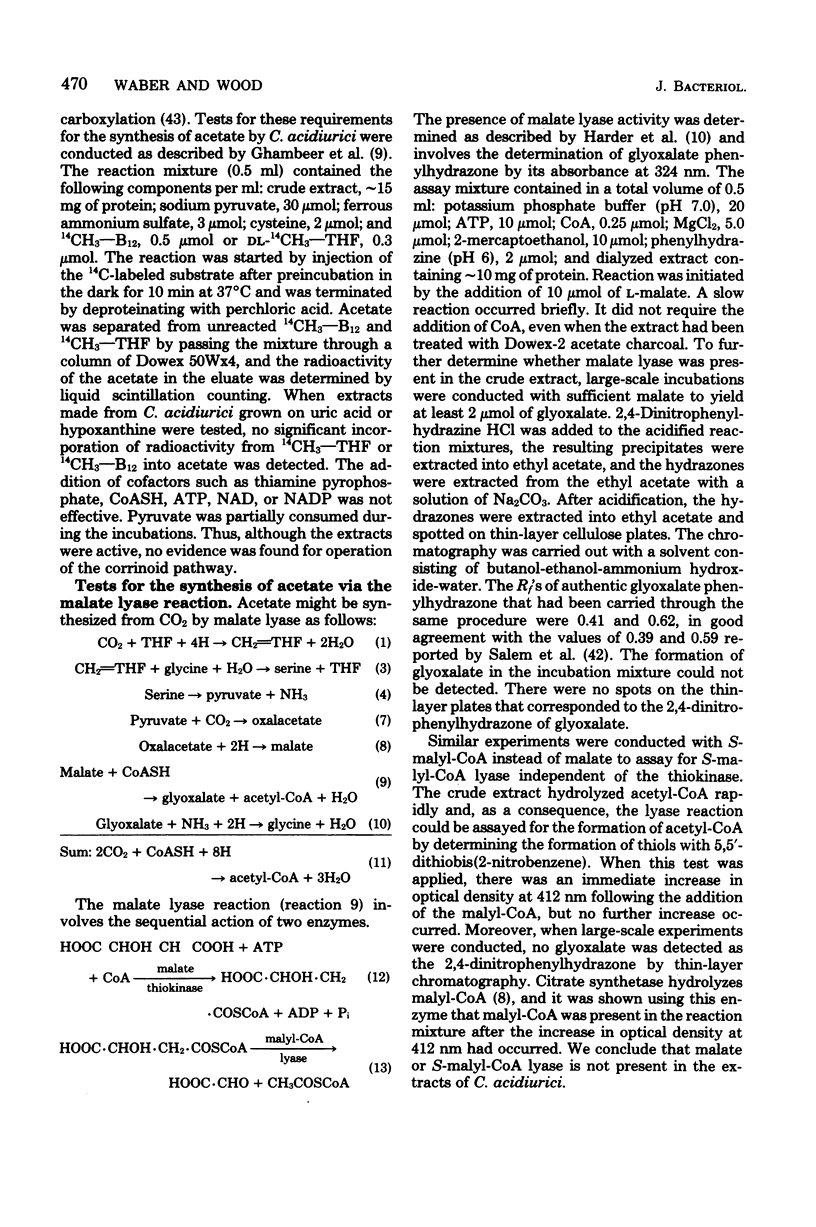
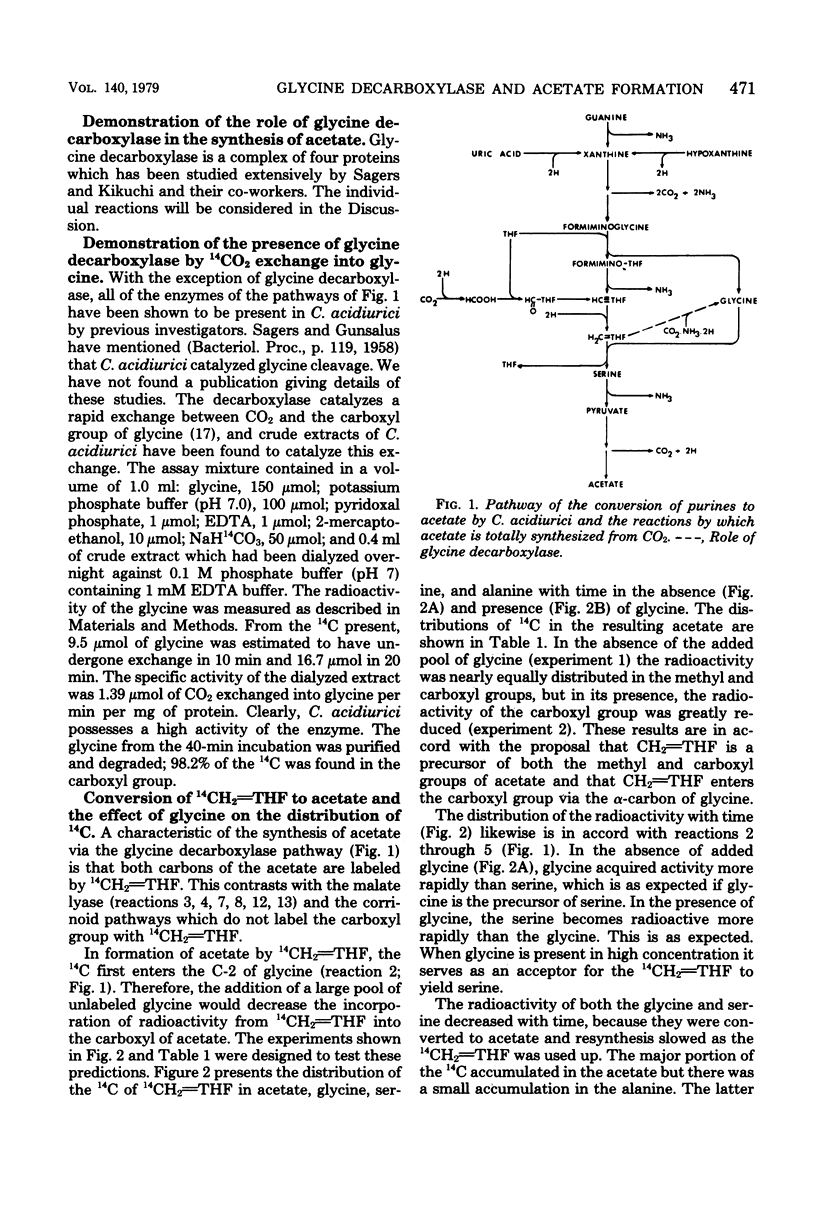
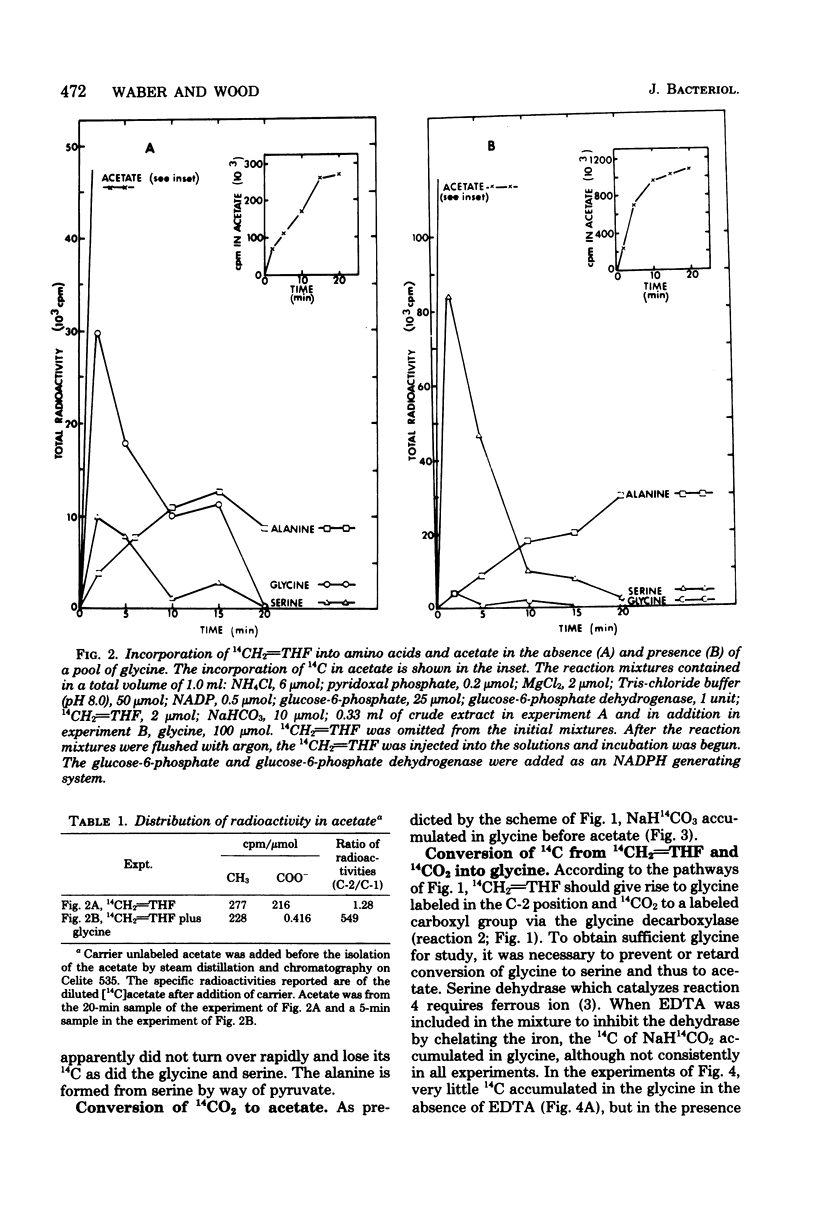
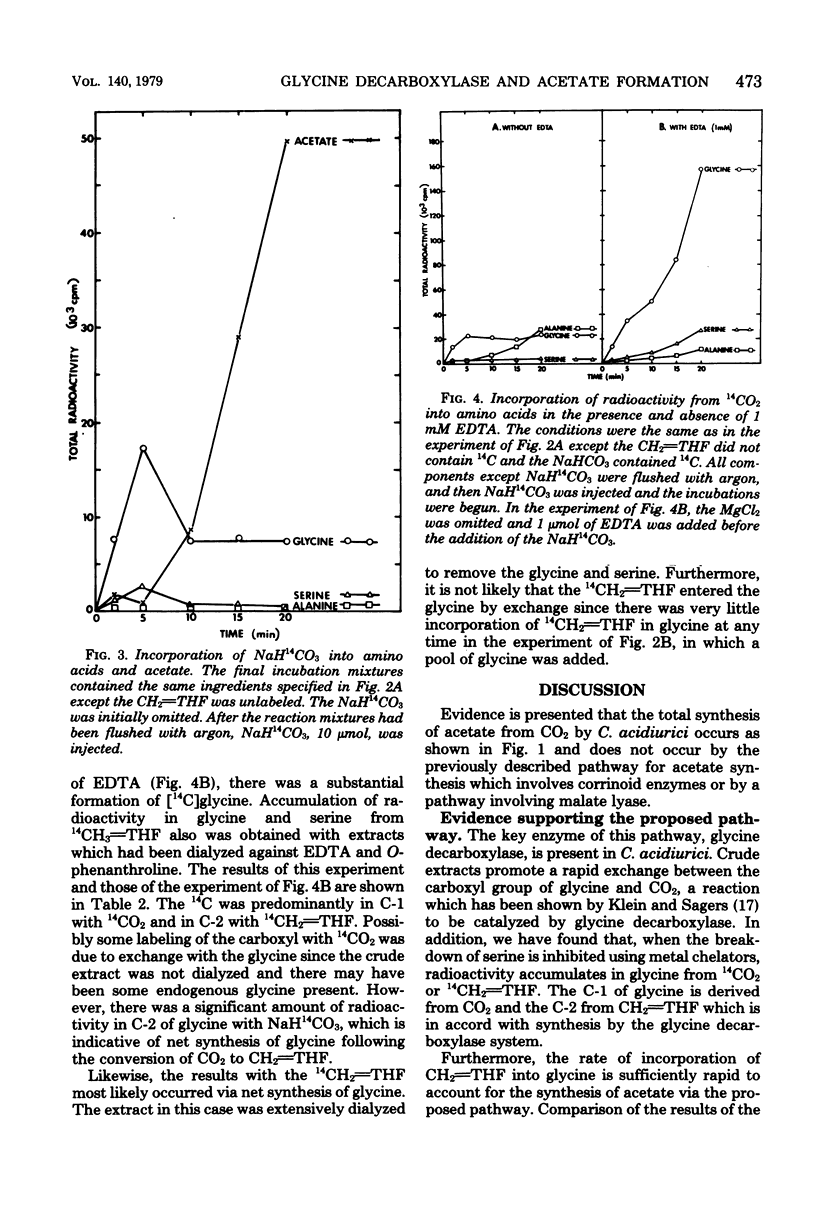
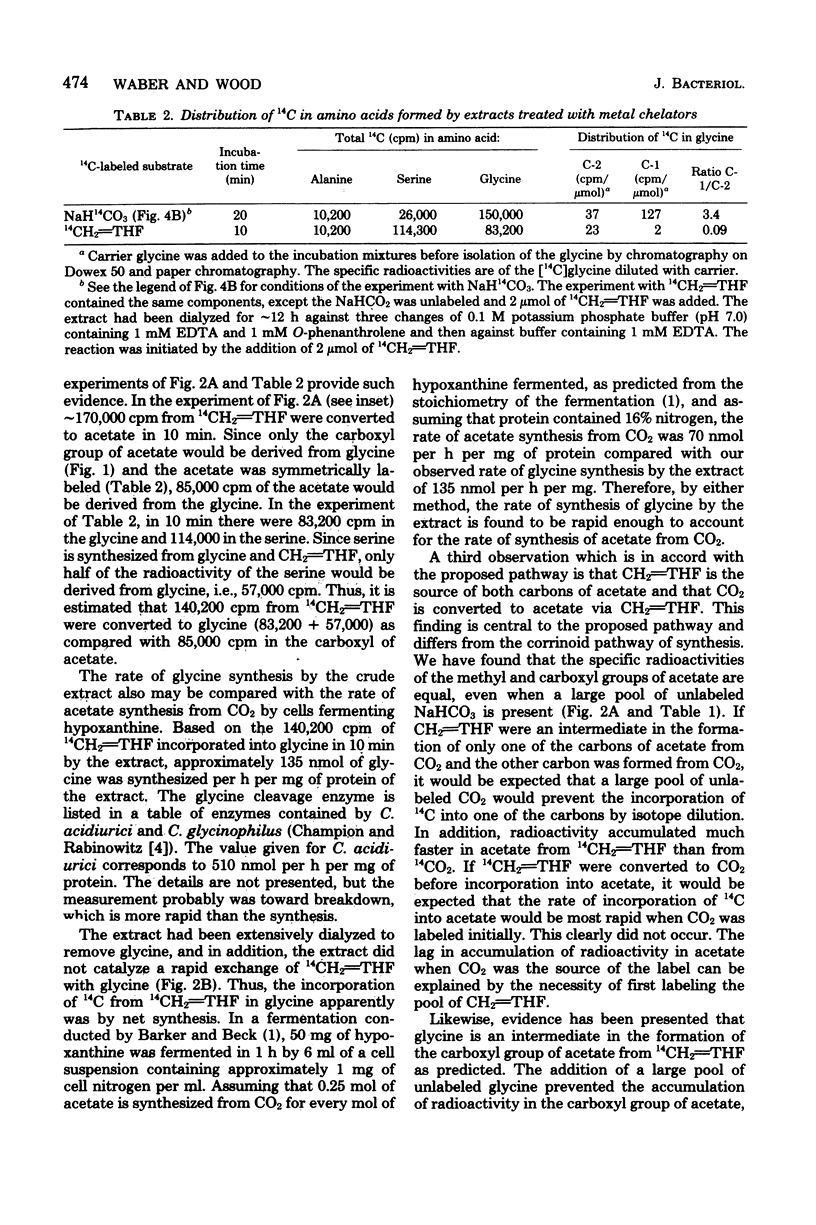
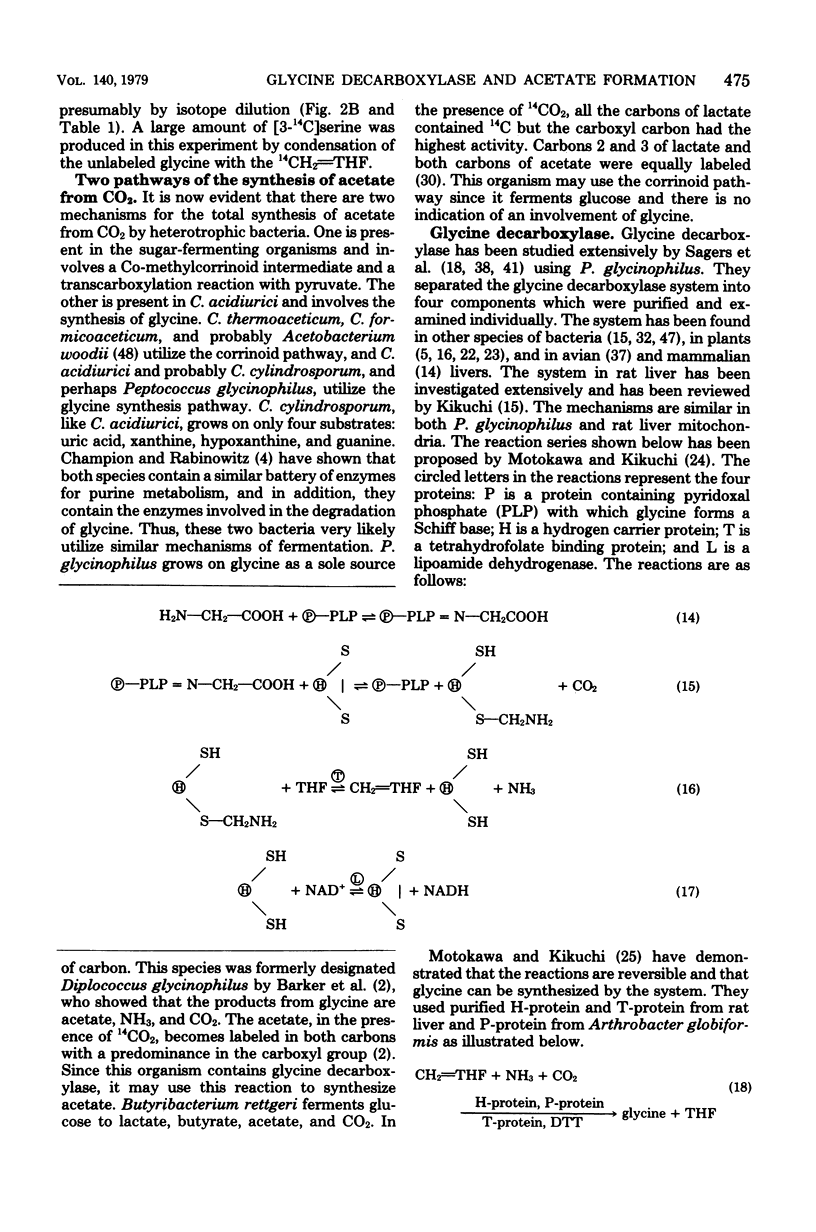
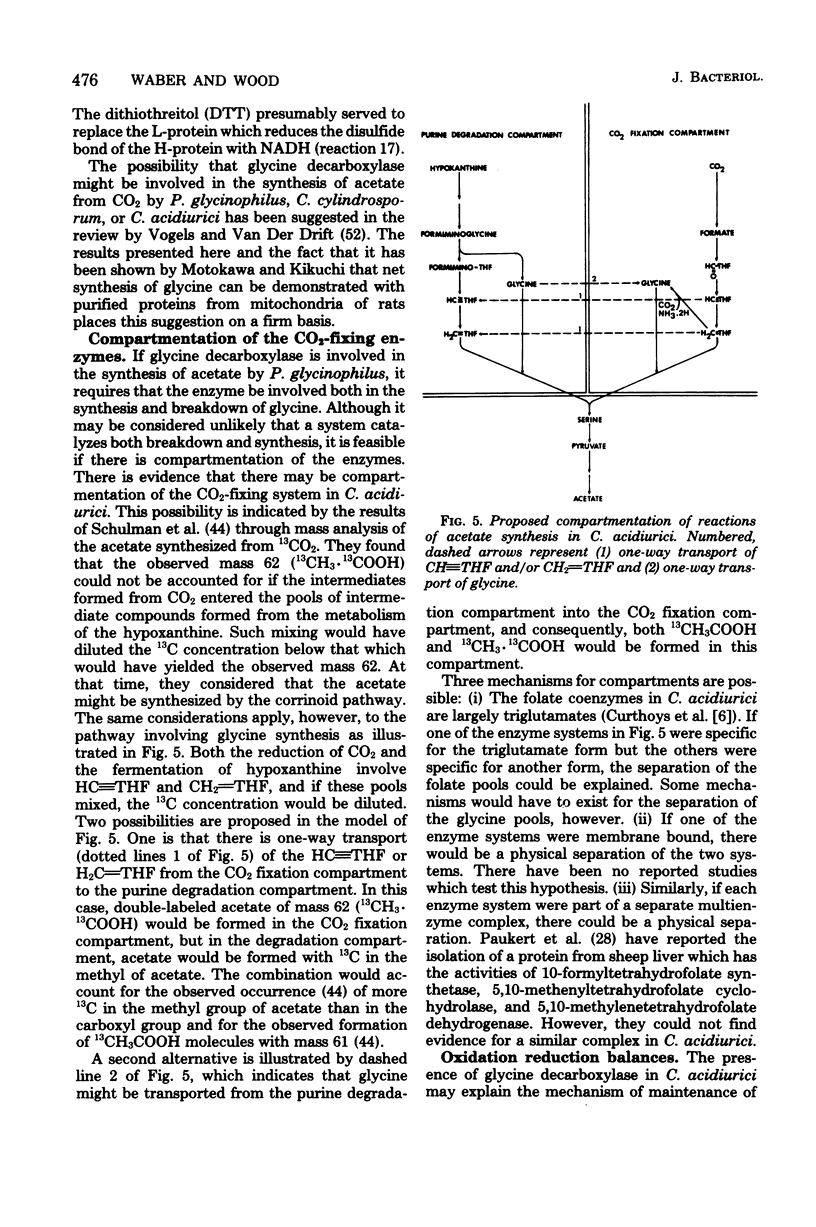
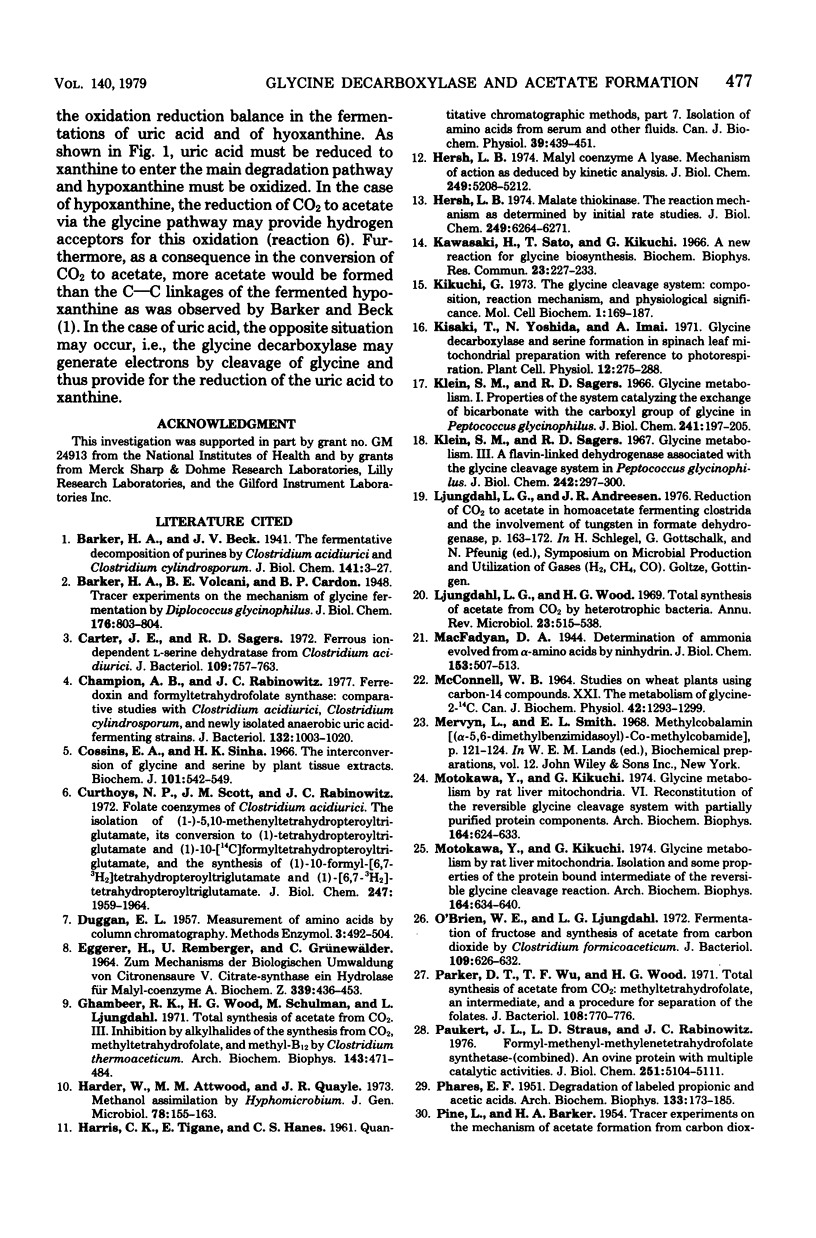
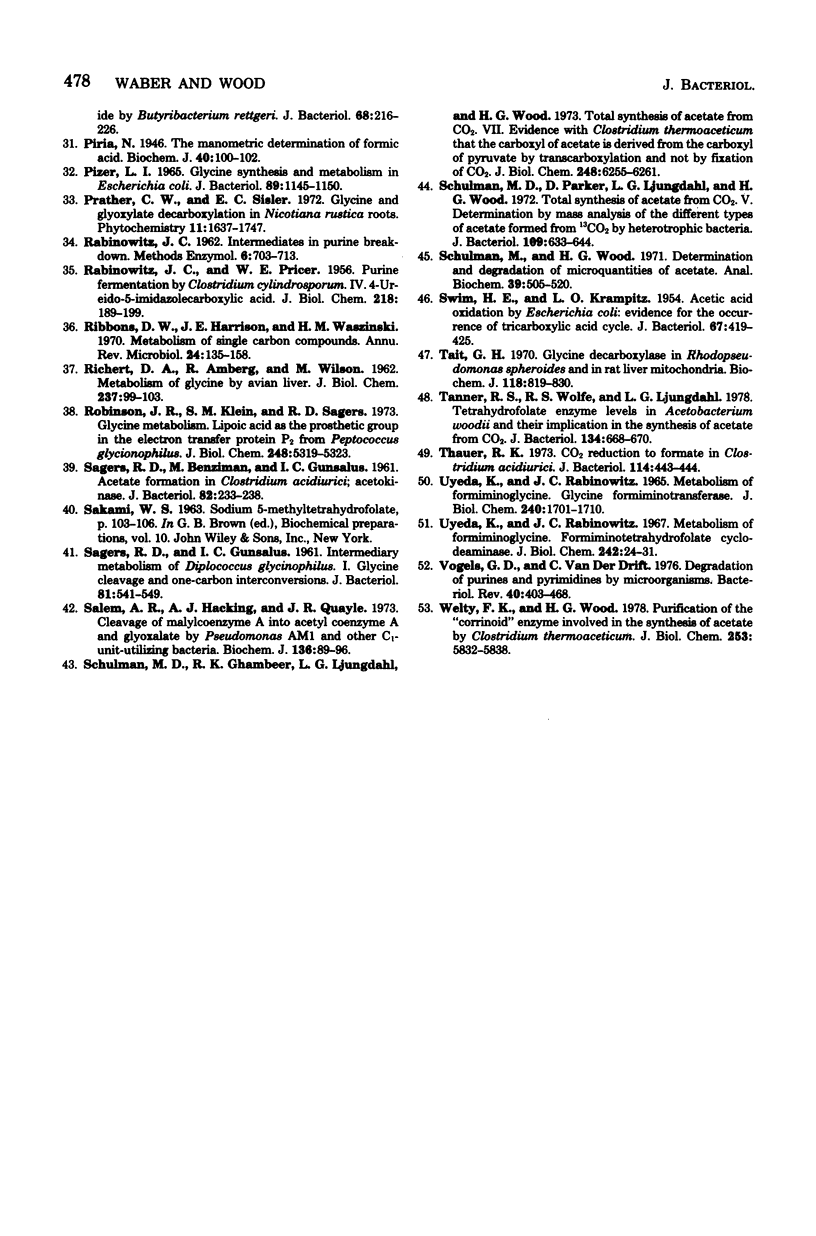
Selected References
These references are in PubMed. This may not be the complete list of references from this article.
- Carter J. E., Sagers R. D. Ferrous ion-dependent L-serine dehydratase from Clostridium acidiurici. J Bacteriol. 1972 Feb;109(2):757–763. doi: 10.1128/jb.109.2.757-763.1972. [DOI] [PMC free article] [PubMed] [Google Scholar]
- Champion A. B., Rabinowitz J. C. Ferredoxin and formyltetrahydrofolate synthetase: comparative studies with Clostridium acidiurici, Clostridium cylindrosporum, and newly isolated anaerobic uric acid-fermenting strains. J Bacteriol. 1977 Dec;132(3):1003–1020. doi: 10.1128/jb.132.3.1003-1020.1977. [DOI] [PMC free article] [PubMed] [Google Scholar]
- Cossins E. A., Sinha S. K. The interconversion of glycine and serine by plant tissue extracts. Biochem J. 1966 Nov;101(2):542–549. doi: 10.1042/bj1010542. [DOI] [PMC free article] [PubMed] [Google Scholar]
- Curthoys N. P., Scott J. M., Rabinowitz J. C. Folate coenzymes of Clostridium acidi-urici. The isolation of (l)-5,10-methenyltetrahydropteroyltriglutamate, its conversion to (l)-tetrahydropteroyltriglutamate and (l)-10-( 14 C)formyltetrahydropteroyltriglutamate, and the synthesis of (l)-10-formyl-(6,7- 3 H 2 )tetrahydropteroyltriglutamate and (l)-(6,7- 3 H 2 )tetrahydropteroyltriglutamate. J Biol Chem. 1972 Apr 10;247(7):1959–1964. [PubMed] [Google Scholar]
- EGGERER H., REMBERGER U., GRUENEWAELDER C. ZUM MECHANISMUS DER BIOLOGISCHEN UMWANDLUNG VON CITRONENSAEURE. V. CITRAT-SYNTHASE, EINE HYDROLASE FUER MALYL-COENZYM A. Biochem Z. 1964 May 22;339:436–453. [PubMed] [Google Scholar]
- Ghambeer R. K., Wood H. G., Schulman M., Ljungdahl L. Total synthesis of acetate from CO2. 3. Inhibition by alkylhalides of the synthesis from CO2, methyltetrahydrofolate, and methyl-B12 by Clostridium thermoaceticum. Arch Biochem Biophys. 1971 Apr;143(2):471–484. doi: 10.1016/0003-9861(71)90232-3. [DOI] [PubMed] [Google Scholar]
- HARRIS C. K., TIGANE E., HANES C. S. Quantitative chromatographic methods. 7. Isolation of amino acids from serum and other fluids. Can J Biochem Physiol. 1961 Feb;39:439–451. doi: 10.1139/o61-042. [DOI] [PubMed] [Google Scholar]
- Hersh L. B. Malate thiokinase. The reaction mechanism as determined by initial rate studies. J Biol Chem. 1974 Oct 10;249(19):6264–6271. [PubMed] [Google Scholar]
- Hersh L. B. Malyl coenzyme A lyase. Mechanism of action as deduced by kinetic analysis. J Biol Chem. 1974 Aug 25;249(16):5208–5212. [PubMed] [Google Scholar]
- Kawasaki H., Sato T., Kikuchi G. A new reaction for glycine biosynthesis. Biochem Biophys Res Commun. 1966 May 3;23(3):227–233. doi: 10.1016/0006-291x(66)90532-8. [DOI] [PubMed] [Google Scholar]
- Kikuchi G. The glycine cleavage system: composition, reaction mechanism, and physiological significance. Mol Cell Biochem. 1973 Jun 27;1(2):169–187. doi: 10.1007/BF01659328. [DOI] [PubMed] [Google Scholar]
- Klein S. M., Sagers R. D. Glycine metabolism. 3. A flavin-linked dehydrogenase associated with the glycine cleavage system in Peptococcus glycinophilus. J Biol Chem. 1967 Jan 25;242(2):297–300. [PubMed] [Google Scholar]
- Klein S. M., Sagers R. D. Glycine metabolism. I. Properties of the system catalyzing the exchange of bicarbonate with the carboxyl group of glycine in Peptococcus glycinophilus. J Biol Chem. 1966 Jan 10;241(1):197–205. [PubMed] [Google Scholar]
- Ljungdahl L. G. Total synthesis of acetate from CO2 by heterotrophic bacteria. Annu Rev Microbiol. 1969;23:515–538. doi: 10.1146/annurev.mi.23.100169.002503. [DOI] [PubMed] [Google Scholar]
- MCCONNELL W. B. STUDIES ON WHEAT PLANTS USING CARBON-14 COMPOUNDS. XXI. THE METABOLISM OF GLYCINE-2-C14. Can J Biochem. 1964 Sep;42:1293–1299. doi: 10.1139/o64-138. [DOI] [PubMed] [Google Scholar]
- Motokawa Y., Kikuchi G. Glycine metabolism by rat liver mitochondria. Isolation and some properties of the protein-bound intermediate of the reversible glycine cleavage reaction. Arch Biochem Biophys. 1974 Oct;164(2):634–640. doi: 10.1016/0003-9861(74)90075-7. [DOI] [PubMed] [Google Scholar]
- Motokawa Y., Kikuchi G. Glycine metabolism by rat liver mitochondria. Reconstruction of the reversible glycine cleavage system with partially purified protein components. Arch Biochem Biophys. 1974 Oct;164(2):624–633. doi: 10.1016/0003-9861(74)90074-5. [DOI] [PubMed] [Google Scholar]
- O'Brien W. E., Ljungdahl L. G. Fermentation of fructose and synthesis of acetate from carbon dioxide by Clostridium formicoaceticum. J Bacteriol. 1972 Feb;109(2):626–632. doi: 10.1128/jb.109.2.626-632.1972. [DOI] [PMC free article] [PubMed] [Google Scholar]
- PHARES E. F. Degradation of labeled propionic and acetic acids. Arch Biochem Biophys. 1951 Sep;33(2):173–178. doi: 10.1016/0003-9861(51)90094-x. [DOI] [PubMed] [Google Scholar]
- PINE L., BARKER H. A. Tracer experiments on the mechanism of acetate formation from carbon dioxide by Butyribacterium rettgeri. J Bacteriol. 1954 Aug;68(2):216–226. doi: 10.1128/jb.68.2.216-226.1954. [DOI] [PMC free article] [PubMed] [Google Scholar]
- PIZER L. I. GLYCINE SYNTHESIS AND METABOLISM IN ESCHERICHIA COLI. J Bacteriol. 1965 Apr;89:1145–1150. doi: 10.1128/jb.89.4.1145-1150.1965. [DOI] [PMC free article] [PubMed] [Google Scholar]
- Parker D. J., Wu T. F., Wood H. G. Total synthesis of acetate from CO 2 : methyltetrahydrofolate, an intermediate, and a procedure for separation of the folates. J Bacteriol. 1971 Nov;108(2):770–776. doi: 10.1128/jb.108.2.770-776.1971. [DOI] [PMC free article] [PubMed] [Google Scholar]
- Paukert J. L., Straus L. D., Rabinowitz J. C. Formyl-methyl-methylenetetrahydrofolate synthetase-(combined). An ovine protein with multiple catalytic activities. J Biol Chem. 1976 Aug 25;251(16):5104–5111. [PubMed] [Google Scholar]
- Pirie N. W. The manometric determination of formic acid. Biochem J. 1946;40(1):100–102. doi: 10.1042/bj0400100. [DOI] [PMC free article] [PubMed] [Google Scholar]
- RABINOWITZ J. C., PRICER W. E., Jr Purine fermentation by Clostridium cylindrosporum. IV. 4-Ureido-5-imidazolecarboxylic acid. J Biol Chem. 1956 Jan;218(1):189–199. [PubMed] [Google Scholar]
- RICHERT D. A., AMBERG R., WILSON M. Metabolism of glycine by avian liver. J Biol Chem. 1962 Jan;237:99–103. [PubMed] [Google Scholar]
- Ribbons D. W., Harrison J. E., Wadzinski A. M. Metabolism of single carbon compounds. Annu Rev Microbiol. 1970;24:135–158. doi: 10.1146/annurev.mi.24.100170.001031. [DOI] [PubMed] [Google Scholar]
- Robinson J. R., Klein S. M., Sagers R. D. Glycine metabolism. Lipoic acid as the prosthetic group in the electron transfer protein P2 from Peptococcus glycinophilus. J Biol Chem. 1973 Aug 10;248(15):5319–5323. [PubMed] [Google Scholar]
- SAGERS R. D., BENZIMAN M., GUNSALUS I. C. Acetate formation in Clostridium acidi-urici: acetokinase. J Bacteriol. 1961 Aug;82:233–238. doi: 10.1002/path.1700820136. [DOI] [PMC free article] [PubMed] [Google Scholar]
- SAGERS R. D., GUNSALUS I. C. Intermediatry metabolism of Diplococcus glycinophilus. I. Glycine cleavage and one-carbon interconversions. J Bacteriol. 1961 Apr;81:541–549. doi: 10.1128/jb.81.4.541-549.1961. [DOI] [PMC free article] [PubMed] [Google Scholar]
- SWIM H. E., KRAMPITZ L. O. Acetic acid oxidation by Escherichia coli; evidence for the occurrence of a tricarboxylic acid cycle. J Bacteriol. 1954 Apr;67(4):419–425. doi: 10.1128/jb.67.4.419-425.1954. [DOI] [PMC free article] [PubMed] [Google Scholar]
- Salem A. R., Hacking A. J., Quayle J. R. Cleavage of malyl-Coenzyme A into acetyl-Coenzyme A and glyoxylate by Pseudomonas AM1 and other C1-unit-utilizing bacteria. Biochem J. 1973 Sep;136(1):89–96. doi: 10.1042/bj1360089. [DOI] [PMC free article] [PubMed] [Google Scholar]
- Schulman M., Ghambeer R. K., Ljungdahl L. G., Wood H. G. Total synthesis of acetate from CO2. VII. Evidence with Clostridium thermoaceticum that the carboxyl of acetate is derived from the carboxyl of pyruvate by transcarboxylation and not by fixation of CO2. J Biol Chem. 1973 Sep 25;248(18):6255–6261. [PubMed] [Google Scholar]
- Schulman M., Parker D., Ljungdahl L. G., Wood H. G. Total synthesis of acetate from CO 2 . V. Determination by mass analysis of the different types of acetate formed from 13 CO 2 by heterotrophic bacteria. J Bacteriol. 1972 Feb;109(2):633–644. doi: 10.1128/jb.109.2.633-644.1972. [DOI] [PMC free article] [PubMed] [Google Scholar]
- Schulman M., Wood H. G. Determination and degradation of microquantities of acetate. Anal Biochem. 1971 Feb;39(2):505–520. doi: 10.1016/0003-2697(71)90441-6. [DOI] [PubMed] [Google Scholar]
- Tait G. H. Glycine decarboxylase in Rhodopseudomonas spheroides and in rat liver mitochondria. Biochem J. 1970 Aug;118(5):819–830. doi: 10.1042/bj1180819. [DOI] [PMC free article] [PubMed] [Google Scholar]
- Tanner R. S., Wolfe R. S., Ljungdahl L. G. Tetrahydrofolate enzyme levels in Acetobacterium woodii and their implication in the synthesis of acetate from CO2. J Bacteriol. 1978 May;134(2):668–670. doi: 10.1128/jb.134.2.668-670.1978. [DOI] [PMC free article] [PubMed] [Google Scholar]
- Thauer R. K. CO 2 reduction to formate in Clostridium acidi-urici. J Bacteriol. 1973 Apr;114(1):443–444. doi: 10.1128/jb.114.1.443-444.1973. [DOI] [PMC free article] [PubMed] [Google Scholar]
- UYEDA K., RABINOWITZ J. C. METABOLISM OF FORMIMINOGLYCINE. GLYCINE FORMIMINOTRANSFERASE. J Biol Chem. 1965 Apr;240:1701–1710. [PubMed] [Google Scholar]
- Uyeda K., Rabinowitz J. C. Metabolism of formiminoglycine. Formiminotetrahydrofolate cyclodeaminase. J Biol Chem. 1967 Jan 10;242(1):24–31. [PubMed] [Google Scholar]
- Vogels G. D., Van der Drift C. Degradation of purines and pyrimidines by microorganisms. Bacteriol Rev. 1976 Jun;40(2):403–468. doi: 10.1128/br.40.2.403-468.1976. [DOI] [PMC free article] [PubMed] [Google Scholar]
- Welty F. K., Wood H. G. Purification of the "corrinoid" enzyme involved in the synthesis of acetate by Clostridium thermoaceticum. J Biol Chem. 1978 Aug 25;253(16):5832–5838. [PubMed] [Google Scholar]