Abstract
Starved cells of Streptococcus lactis ML3 (grown previously on galactose, lactose, or maltose) accumulated methyl-beta-D-thiogalactopyranoside (TMG) by the lactose:phosphotransferase system. More than 98% of accumulated sugar was present as a phosphorylated derivative, TMG-6-phosphate (TMG-6P). When a phosphotransferase system sugar (glucose, mannose, 2-deoxyglucose, or lactose) was added to the medium simultaneously with TMG, the beta-galactoside was excluded from the cells. Galactose enhanced the accumulation of TMG-6P. Glucose, mannose, lactose, or maltose plus arginine, was added to a suspension of TMG-6P-loaded cells of S. lactis ML3, elicited rapid expulsion of intracellular solute. The material recovered in the medium was exclusively free TMG. Expulsion of galactoside required both entry and metabolism of an appropriate sugar, and intracellular dephosphorylation of TMG-6P preceded efflux of TMG. The rate of dephosphorylation of TMG-6P by permeabilized cells was increased two-to threefold by adenosine 5'-triphosphate but was strongly inhibited by fluoride. S. lactis ML3 (DGr) was derived from S. lactis ML3 by positive selection for resistance to 2-deoxy-D-glucose and was defective in the enzyme IIMan component of the glucose:phosphotransferase system. Neither glucose nor mannose excluded TMG from cells of S. lactic ML3 (DGr), and these two sugars failed to elicit TMG expulsion from preloaded cells of the mutant strain. Accumulation of TMG-6P by S. lactis ML3 can be regulation by two independent mechanisms whose activities promote exclusion or expulsion of galactoside from the cell.
Full text
PDF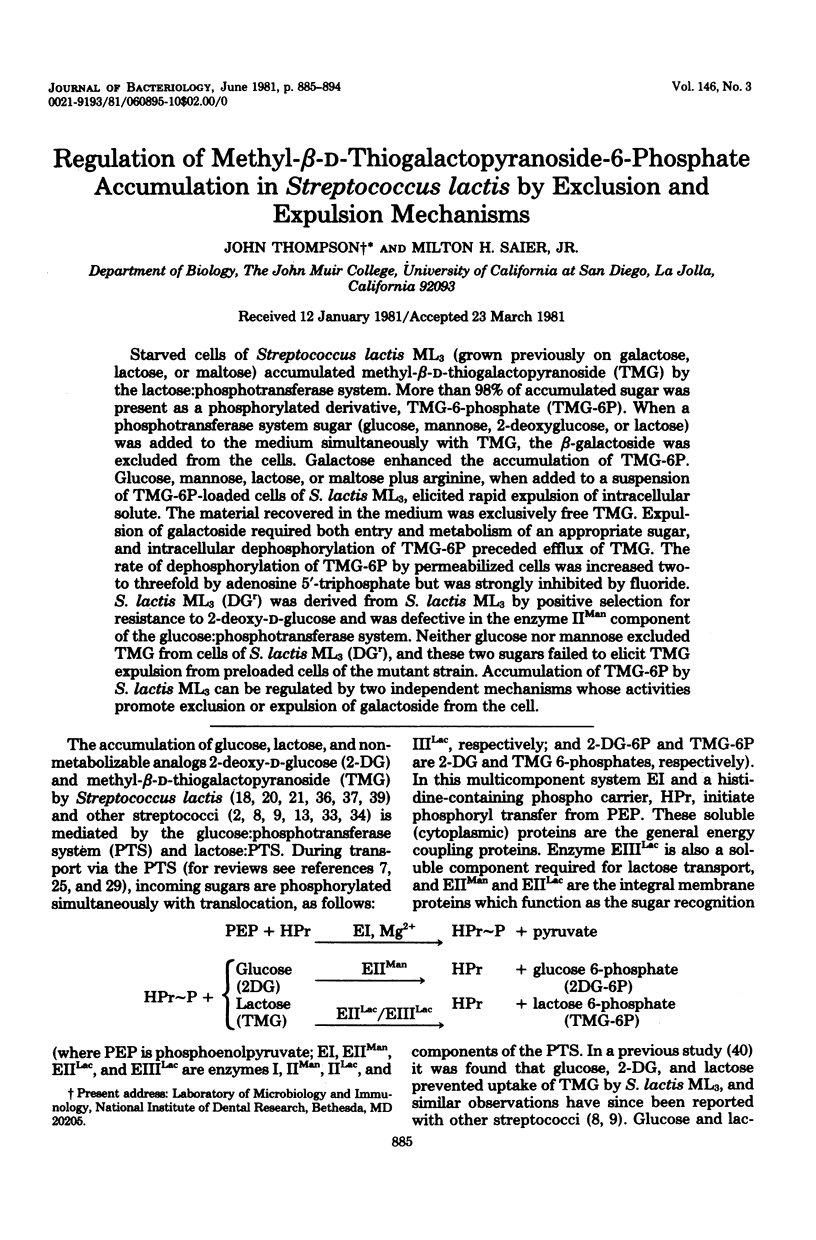
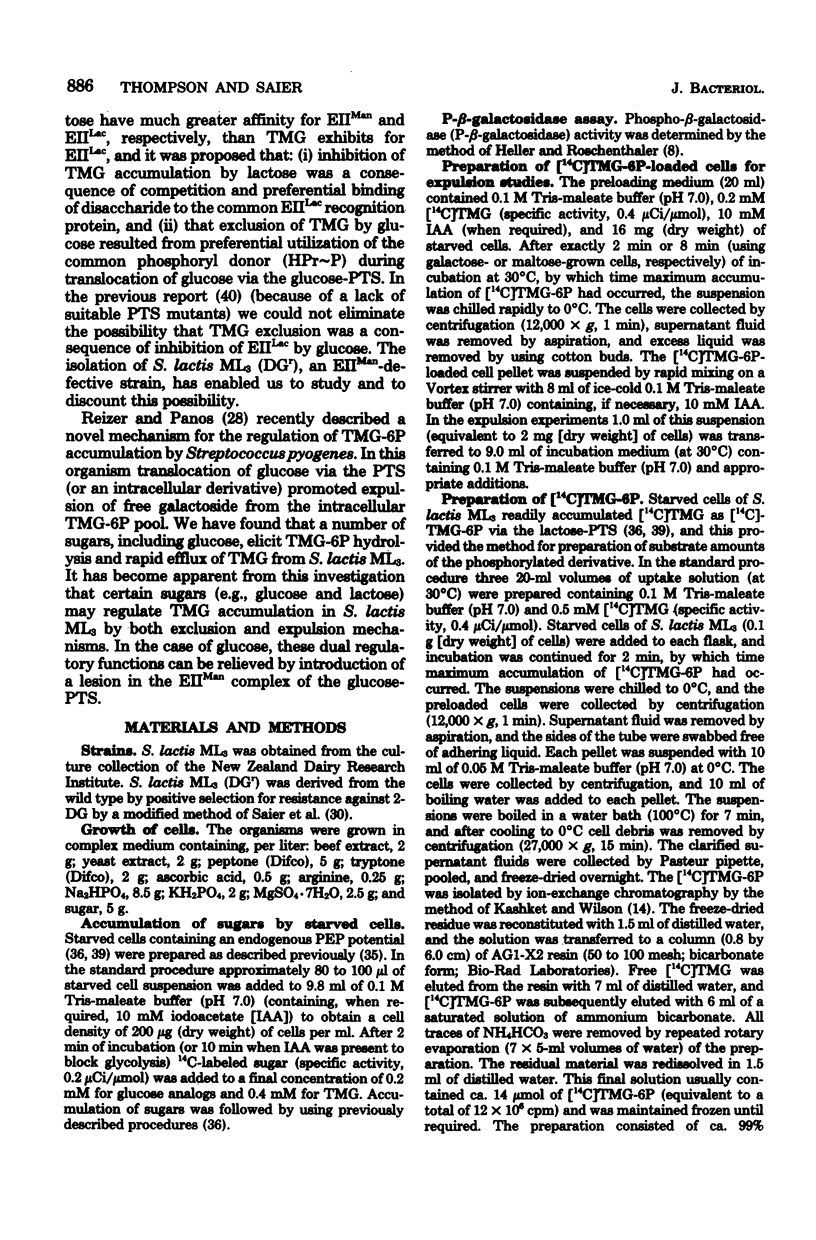
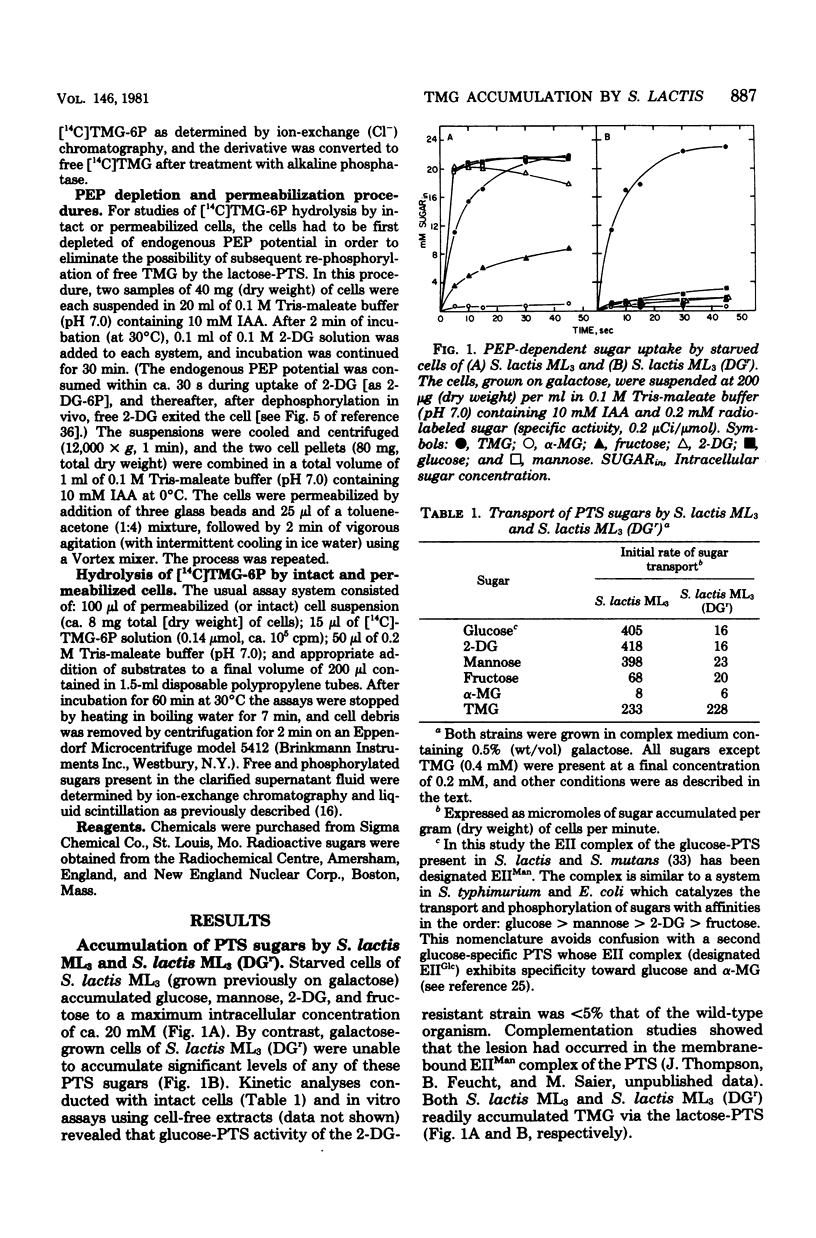
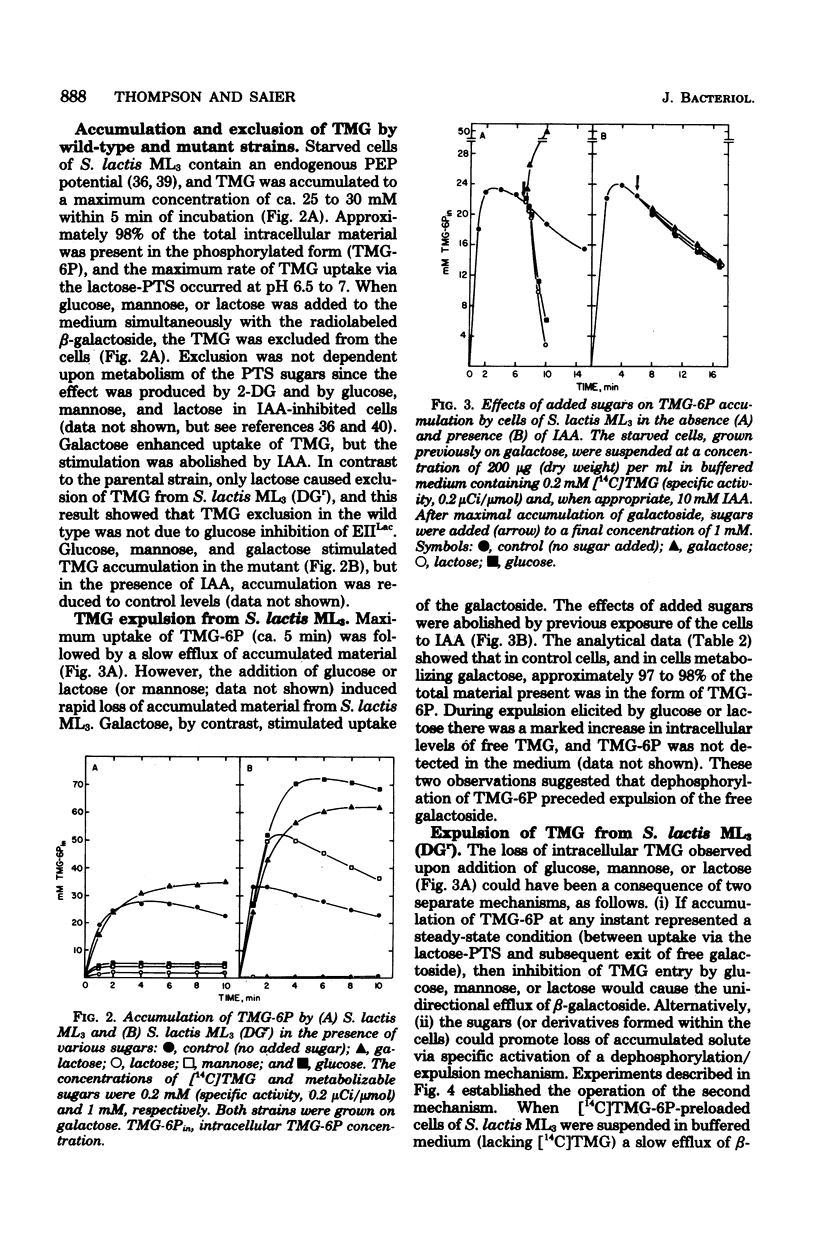
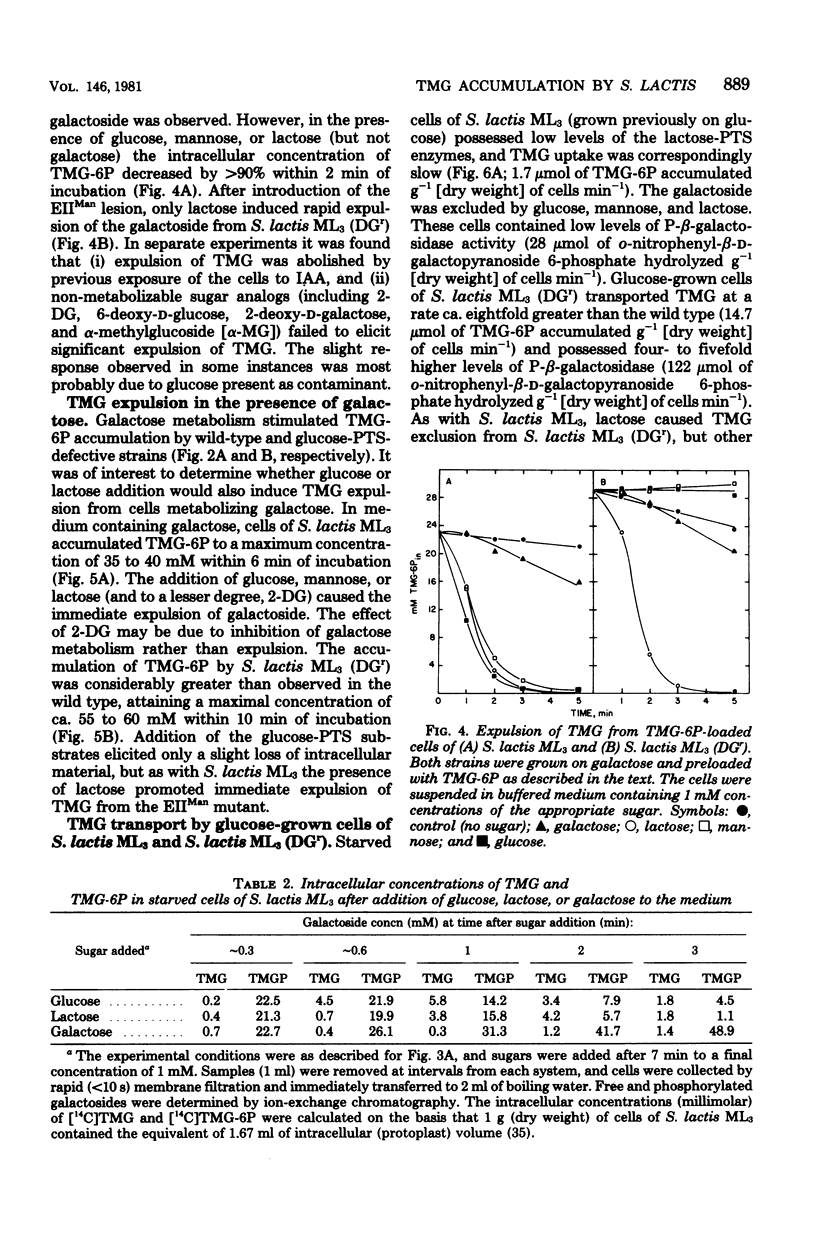
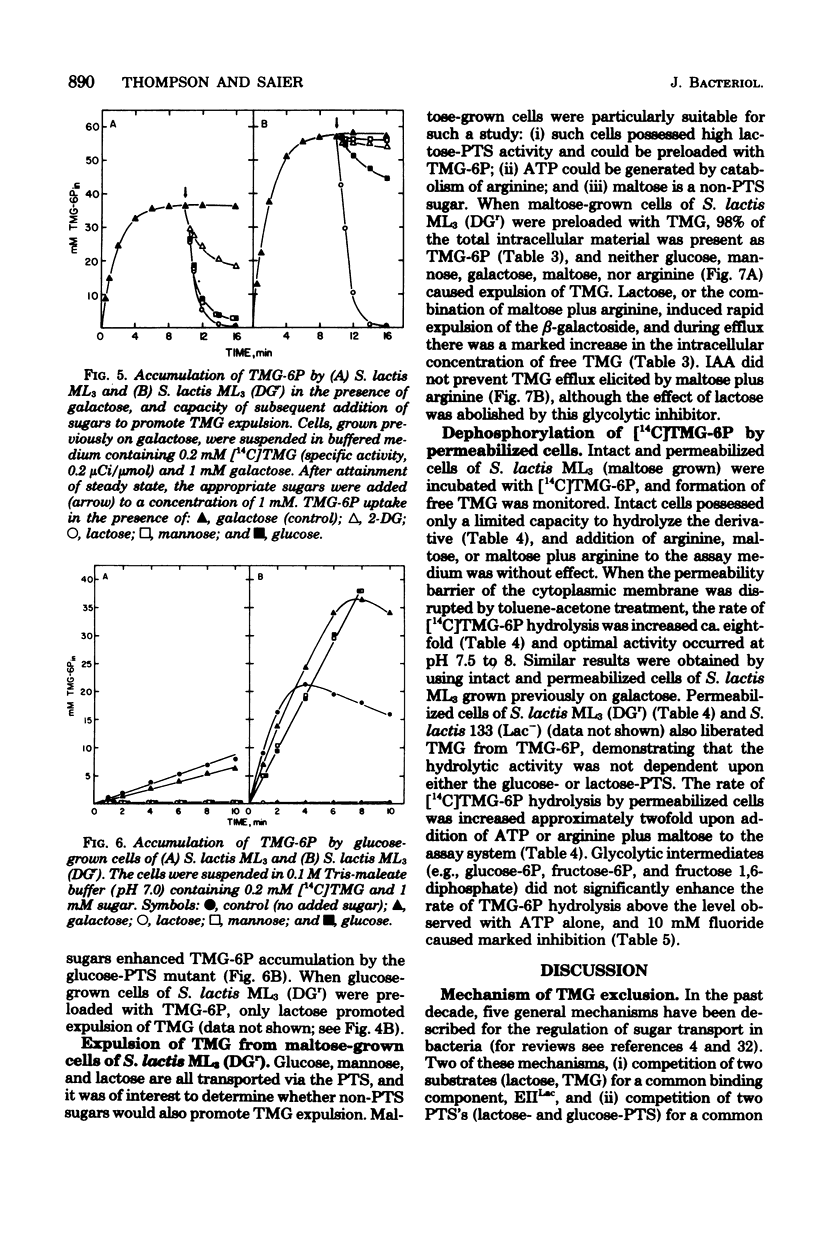
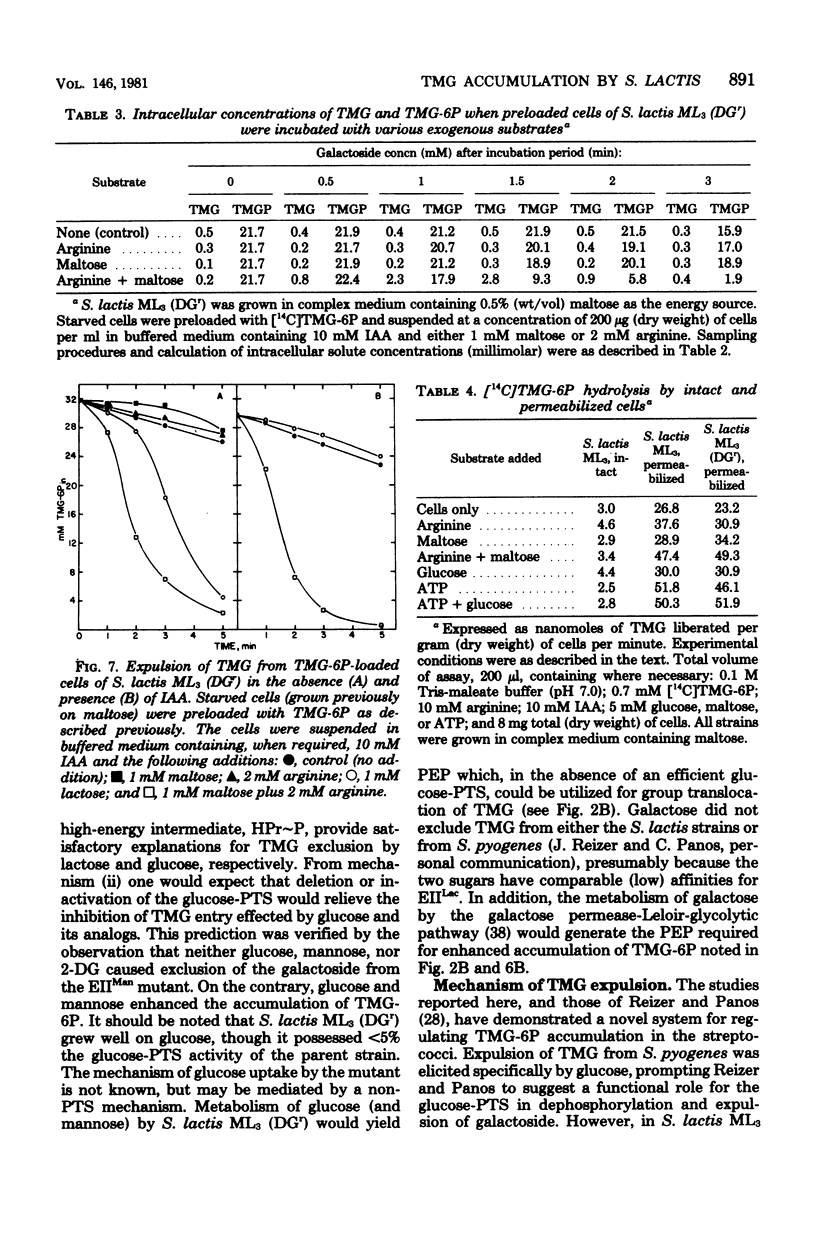
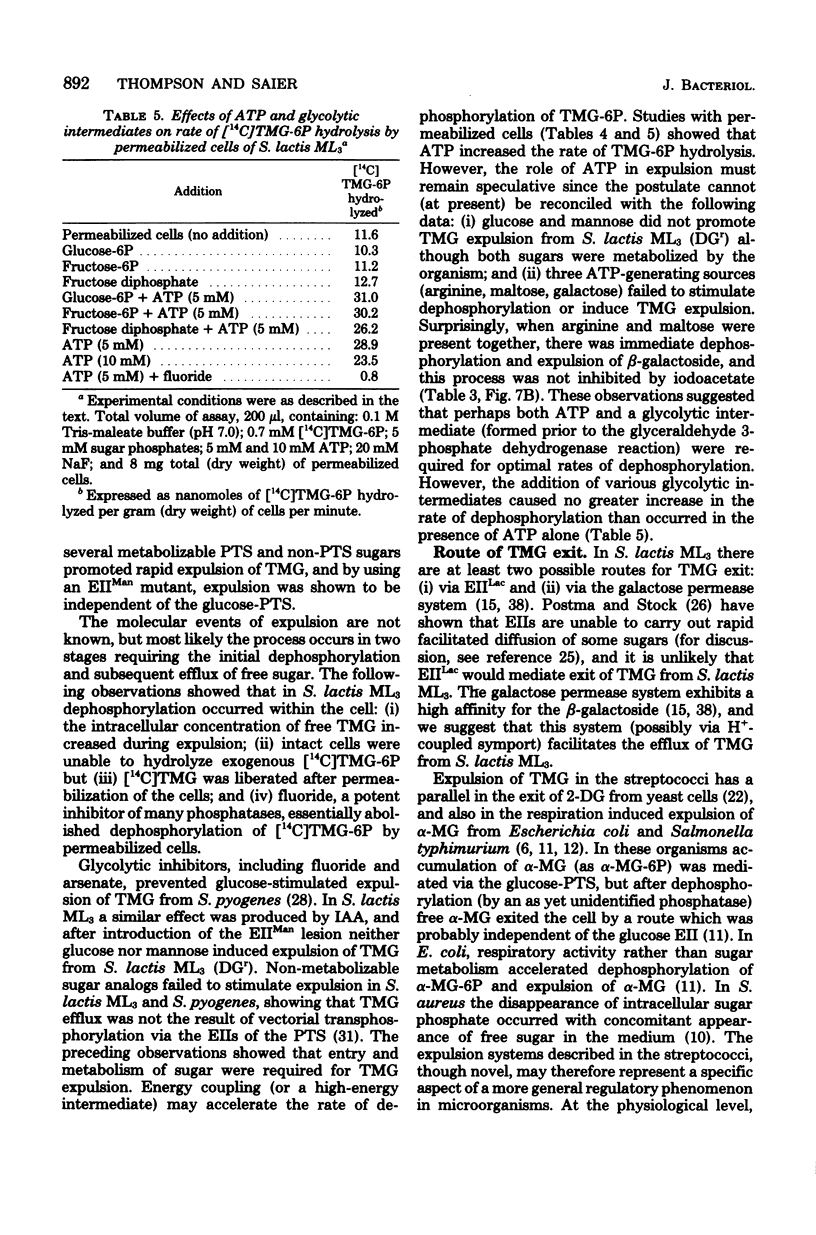
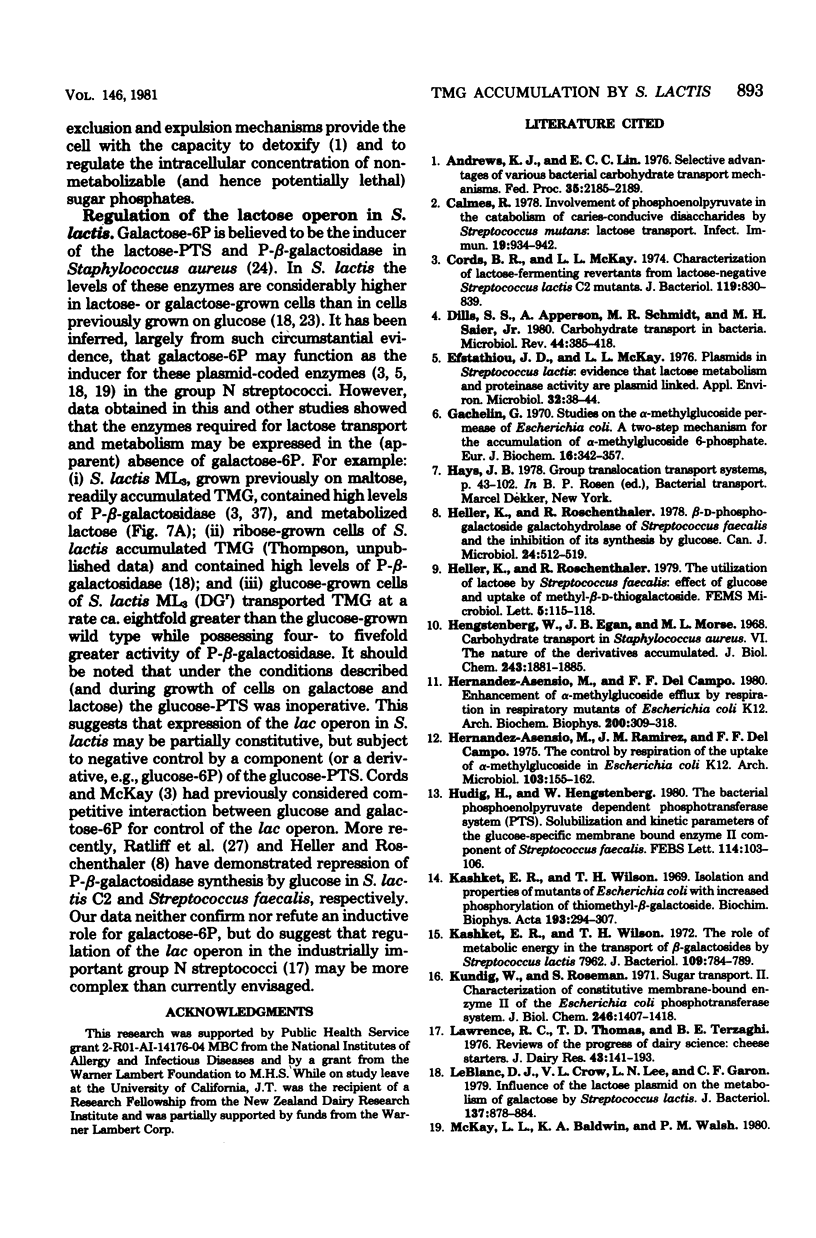
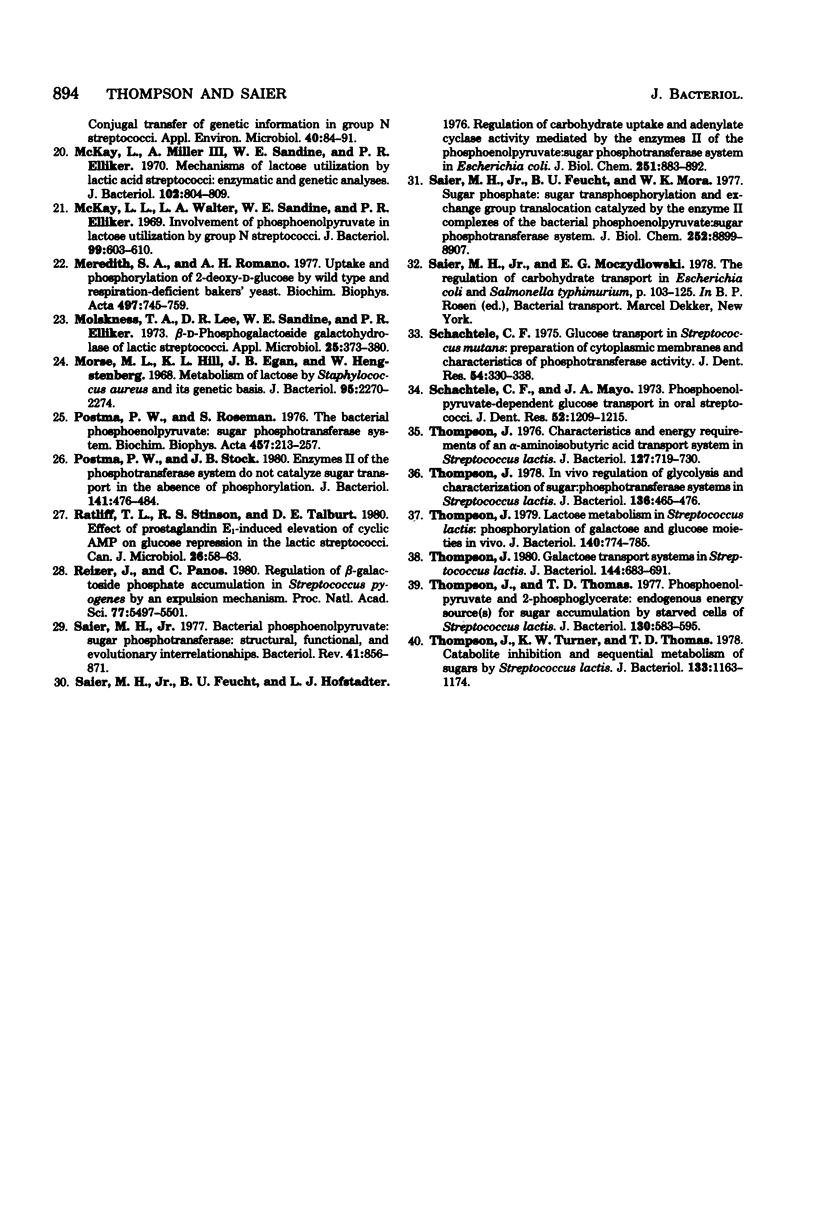
Selected References
These references are in PubMed. This may not be the complete list of references from this article.
- Andrews K. J., Lin E. C. Selective advantages of various bacterial carbohydrate transport mechanisms. Fed Proc. 1976 Aug;35(10):2185–2189. [PubMed] [Google Scholar]
- Calmes R. Involvement of phosphoenolpyruvate in the catabolism of caries-conducive disaccharides by Streptococcus mutans: lactose transport. Infect Immun. 1978 Mar;19(3):934–942. doi: 10.1128/iai.19.3.934-942.1978. [DOI] [PMC free article] [PubMed] [Google Scholar]
- Cords B. R., McKay L. L. Characterization of lactose-fermenting revertants from lactose-negative Streptococcus lactis C2 mutants. J Bacteriol. 1974 Sep;119(3):830–839. doi: 10.1128/jb.119.3.830-839.1974. [DOI] [PMC free article] [PubMed] [Google Scholar]
- Dills S. S., Apperson A., Schmidt M. R., Saier M. H., Jr Carbohydrate transport in bacteria. Microbiol Rev. 1980 Sep;44(3):385–418. doi: 10.1128/mr.44.3.385-418.1980. [DOI] [PMC free article] [PubMed] [Google Scholar]
- Gachelin G. Studies on the alpha-methylglucoside permease of Escherichia coli. A two-step mechanism for the accumulation of alpha-methylglucoside 6-phosphate. Eur J Biochem. 1970 Oct;16(2):342–357. doi: 10.1111/j.1432-1033.1970.tb01088.x. [DOI] [PubMed] [Google Scholar]
- Heller K., Röschenthaler R. beta-D-phosphogalactoside galactohydrolase of Streptococcus faecalis and the inhibition of its synthesis by glucose. Can J Microbiol. 1978 May;24(5):512–519. doi: 10.1139/m78-084. [DOI] [PubMed] [Google Scholar]
- Hengstenberg W., Egan J. B., Morse M. L. Carbohydrate transport in Staphylococcus aureus. VI. The nature of the derivatives accumulated. J Biol Chem. 1968 Apr 25;243(8):1881–1885. [PubMed] [Google Scholar]
- Hernandez-Asensio M., Del Campo F. F. Enhancement of alpha-methylglucoside efflux by respiration in respiratory mutants of Escherichia coli K-12. Arch Biochem Biophys. 1980 Apr 1;200(2):309–318. doi: 10.1016/0003-9861(80)90360-4. [DOI] [PubMed] [Google Scholar]
- Hernandez-Asensio M., Ramirez J. M., Del Campo F. F. The control by respiration of the uptake of alpha-methyl glucoside in Escherichia coli K12. Arch Microbiol. 1975 Apr 7;103(2):155–162. doi: 10.1007/BF00436343. [DOI] [PubMed] [Google Scholar]
- Hüdig H., Hengstenberg W. The bacterial phosphoenolpyruvate dependent phosphotransferase system (PTS): solubilisation and kinetic parameters of the glucose-specific membrane bound enzyme II component of Streptococcus faecalis. FEBS Lett. 1980 May 19;114(1):103–106. doi: 10.1016/0014-5793(80)80869-6. [DOI] [PubMed] [Google Scholar]
- Kashket E. R., Wilson T. H. Isolation and properties of mutants of Escherichia coli with increased phosphorylations of thiomethyl-beta-galactoside. Biochim Biophys Acta. 1969;193(2):294–307. doi: 10.1016/0005-2736(69)90190-4. [DOI] [PubMed] [Google Scholar]
- Kashket E. R., Wilson T. H. Role of metabolic energy in the transport of -galactosides by Streptococcus lactis. J Bacteriol. 1972 Feb;109(2):784–789. doi: 10.1128/jb.109.2.784-789.1972. [DOI] [PMC free article] [PubMed] [Google Scholar]
- Kundig W., Roseman S. Sugar transport. II. Characterization of constitutive membrane-bound enzymes II of the Escherichia coli phosphotransferase system. J Biol Chem. 1971 Mar 10;246(5):1407–1418. [PubMed] [Google Scholar]
- LeBlanc D. J., Crow V. L., Lee L. N., Garon C. F. Influence of the lactose plasmid on the metabolism of galactose by Streptococcus lactis. J Bacteriol. 1979 Feb;137(2):878–884. doi: 10.1128/jb.137.2.878-884.1979. [DOI] [PMC free article] [PubMed] [Google Scholar]
- McKay L. L., Baldwin K. A., Walsh P. M. Conjugal transfer of genetic information in group N streptococci. Appl Environ Microbiol. 1980 Jul;40(1):84–89. doi: 10.1128/aem.40.1.84-91.1980. [DOI] [PMC free article] [PubMed] [Google Scholar]
- McKay L. L., Walter L. A., Sandine W. E., Elliker P. R. Involvement of phosphoenolpyruvate in lactose utilization by group N streptococci. J Bacteriol. 1969 Aug;99(2):603–610. doi: 10.1128/jb.99.2.603-610.1969. [DOI] [PMC free article] [PubMed] [Google Scholar]
- McKay L., Miller A., 3rd, Sandine W. E., Elliker P. R. Mechanisms of lactose utilization by lactic acid streptococci: enzymatic and genetic analyses. J Bacteriol. 1970 Jun;102(3):804–809. doi: 10.1128/jb.102.3.804-809.1970. [DOI] [PMC free article] [PubMed] [Google Scholar]
- Meredith S. A., Romano A. H. Uptake and phosphorylation of 2-deoxy-D-glucose by wild type and respiration-deficient bakers' yeast. Biochim Biophys Acta. 1977 May 26;497(3):745–759. doi: 10.1016/0304-4165(77)90295-1. [DOI] [PubMed] [Google Scholar]
- Molskness T. A., Lee D. R., Sandine W. E., Elliker P. R. -D-phosphogalactoside galactohydrolase of lactic streptococci. Appl Microbiol. 1973 Mar;25(3):373–380. doi: 10.1128/am.25.3.373-380.1973. [DOI] [PMC free article] [PubMed] [Google Scholar]
- Morse M. L., Hill K. L., Egan J. B., Hengstenberg W. Metabolism of lactose by Staphylococcus aureus and its genetic basis. J Bacteriol. 1968 Jun;95(6):2270–2274. doi: 10.1128/jb.95.6.2270-2274.1968. [DOI] [PMC free article] [PubMed] [Google Scholar]
- Postma P. W., Stock J. B. Enzymes II of the phosphotransferase system do not catalyze sugar transport in the absence of phosphorylation. J Bacteriol. 1980 Feb;141(2):476–484. doi: 10.1128/jb.141.2.476-484.1980. [DOI] [PMC free article] [PubMed] [Google Scholar]
- Ratliff T. L., Stinson R. S., Talburt D. E. Effect of prostaglandin E1-induced elevation of cyclic AMP on glucose repression in the lactic streptococci. Can J Microbiol. 1980 Jan;26(1):58–63. doi: 10.1139/m80-009. [DOI] [PubMed] [Google Scholar]
- Saier M. H., Jr Bacterial phosphoenolpyruvate: sugar phosphotransferase systems: structural, functional, and evolutionary interrelationships. Bacteriol Rev. 1977 Dec;41(4):856–871. doi: 10.1128/br.41.4.856-871.1977. [DOI] [PMC free article] [PubMed] [Google Scholar]
- Saier M. H., Jr, Feucht B. U., Hofstadter L. J. Regulation of carbohydrate uptake and adenylate cyclase activity mediated by the enzymes II of the phosphoenolpyruvate: sugar phosphotransferase system in Escherichia coli. J Biol Chem. 1976 Feb 10;251(3):883–892. [PubMed] [Google Scholar]
- Saier M. H., Jr, Feucht B. U., Mora W. K. Sugar phosphate: sugar transphosphorylation and exchange group translocation catalyzed by the enzyme 11 complexes of the bacterial phosphoenolpyruvate: sugar phosphotransferase system. J Biol Chem. 1977 Dec 25;252(24):8899–8907. [PubMed] [Google Scholar]
- Schachtele C. F., Mayo J. A. Phosphoenolpyruvate-dependent glucose transport in oral streptococci. J Dent Res. 1973 Nov-Dec;52(6):1209–1215. doi: 10.1177/00220345730520060801. [DOI] [PubMed] [Google Scholar]
- Thompson J. Characteristics and energy requirements of an alpha-aminoisobutyric acid transport system in Streptococcus lactis. J Bacteriol. 1976 Aug;127(2):719–730. doi: 10.1128/jb.127.2.719-730.1976. [DOI] [PMC free article] [PubMed] [Google Scholar]
- Thompson J. Galactose transport systems in Streptococcus lactis. J Bacteriol. 1980 Nov;144(2):683–691. doi: 10.1128/jb.144.2.683-691.1980. [DOI] [PMC free article] [PubMed] [Google Scholar]
- Thompson J. Lactose metabolism in Streptococcus lactis: phosphorylation of galactose and glucose moieties in vivo. J Bacteriol. 1979 Dec;140(3):774–785. doi: 10.1128/jb.140.3.774-785.1979. [DOI] [PMC free article] [PubMed] [Google Scholar]
- Thompson J., Thomas T. D. Phosphoenolpyruvate and 2-phosphoglycerate: endogenous energy source(s) for sugar accumulation by starved cells of Streptococcus lactis. J Bacteriol. 1977 May;130(2):583–595. doi: 10.1128/jb.130.2.583-595.1977. [DOI] [PMC free article] [PubMed] [Google Scholar]
- Thompson J., Turner K. W., Thomas T. D. Catabolite inhibition and sequential metabolism of sugars by Streptococcus lactis. J Bacteriol. 1978 Mar;133(3):1163–1174. doi: 10.1128/jb.133.3.1163-1174.1978. [DOI] [PMC free article] [PubMed] [Google Scholar]