Abstract
The influence of pH on the proton motive force of Vibrio costicola was determined by measuring the distributions of triphenylmethylphosphonium cation (membrane potential, delta psi) and either dimethyloxazolidinedione or methylamine (osmotic component, delta pH). As the pH of the medium was adjusted from 5.7 to 9.0, the proton motive force steadily decreased from about 170 to 100 mV. This decline occurred, despite a large increase in the membrane potential to its maximum value at pH 9.0, because of the loss of the pH gradient (inside alkaline). The cytoplasm and medium were of equal pH at 7.5; membrane permeability properties were lost at the pH extremes of 5.0 and 9.5. Protonophores and monensin prevented the net efflux of protons normally found when an oxygen pulse was given to an anaerobic cell suspension. A Na+/H+ antiport activity was measured for both Na+ influx and efflux and was shown to be dissipated by protonophores and monensin. These results strongly favor the concept that respiratory energy is used for proton efflux and that the resulting proton motive force may be converted to a sodium motive force through Na+/H+ antiport (driven by delta psi). A role for antiport activity in pH regulation of the cytosol can also explain the broad pH range for optimal growth, extending to the alkaline extreme of pH 9.0.
Full text
PDF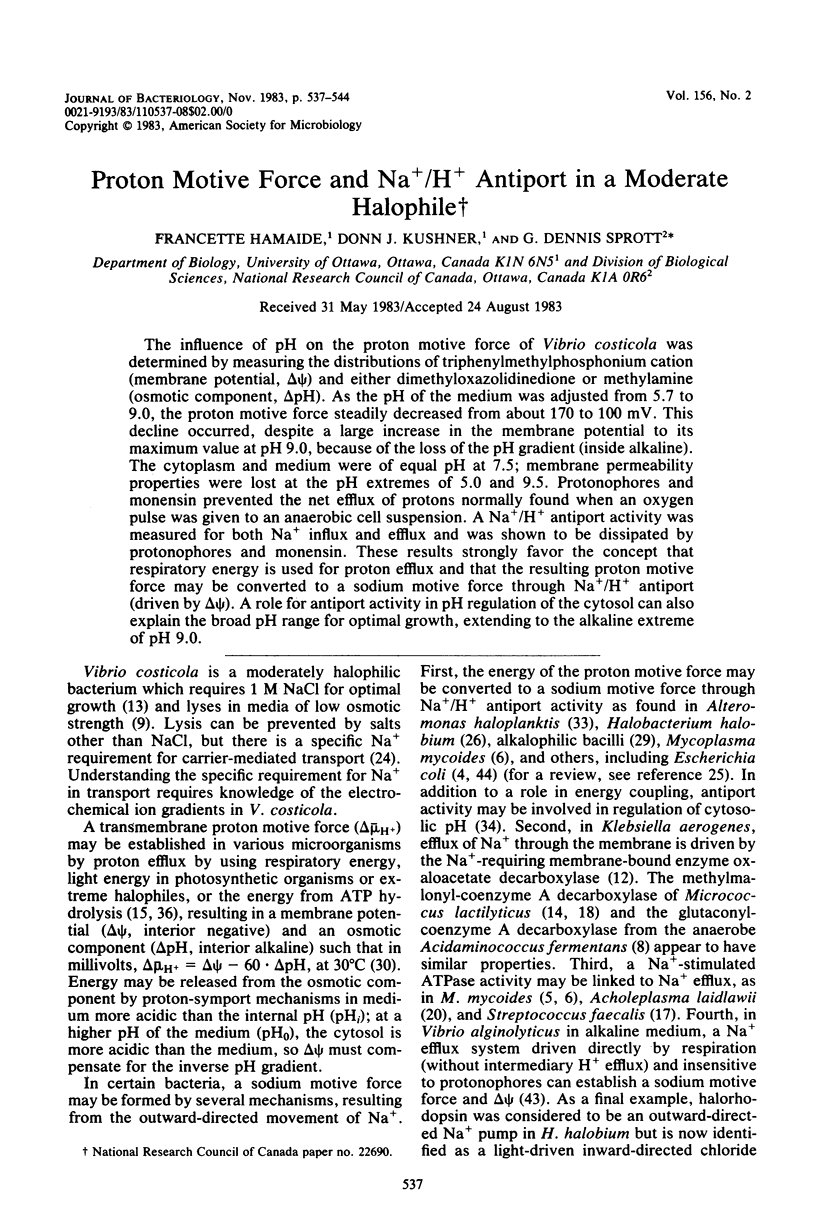
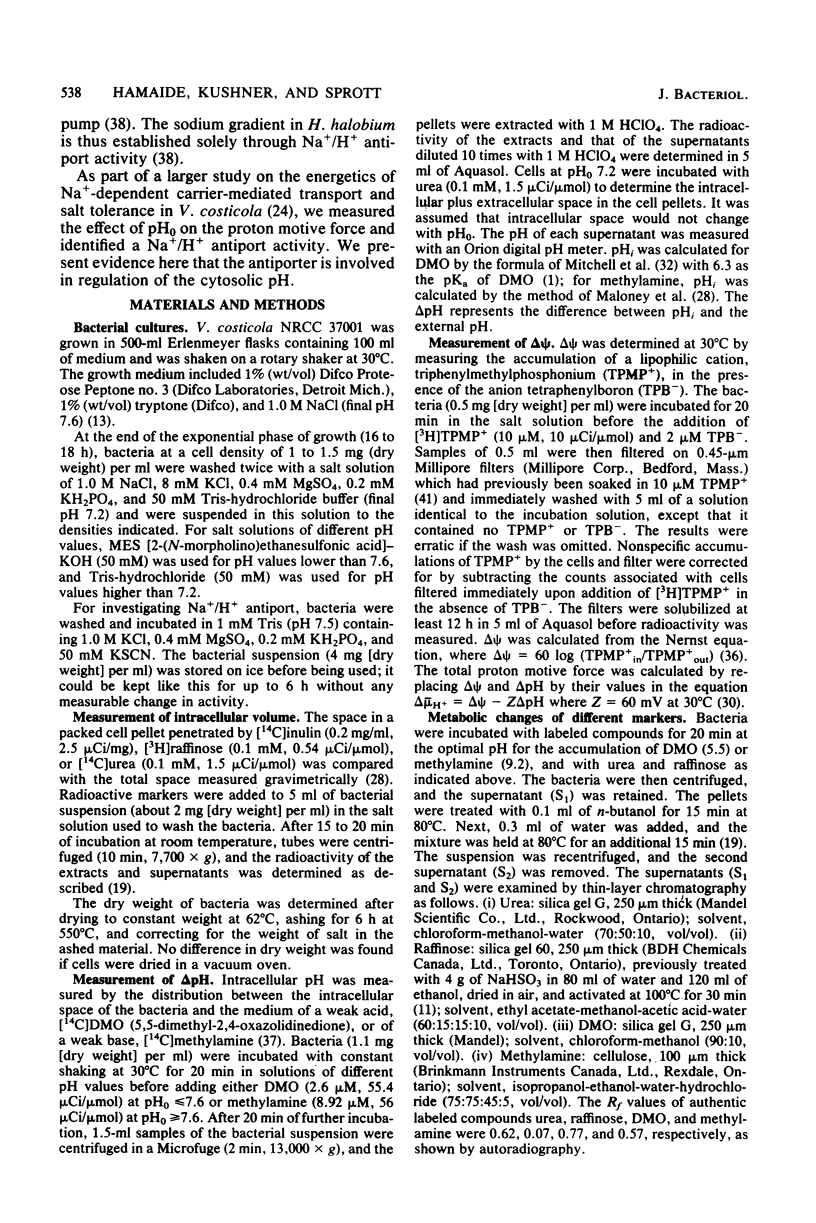
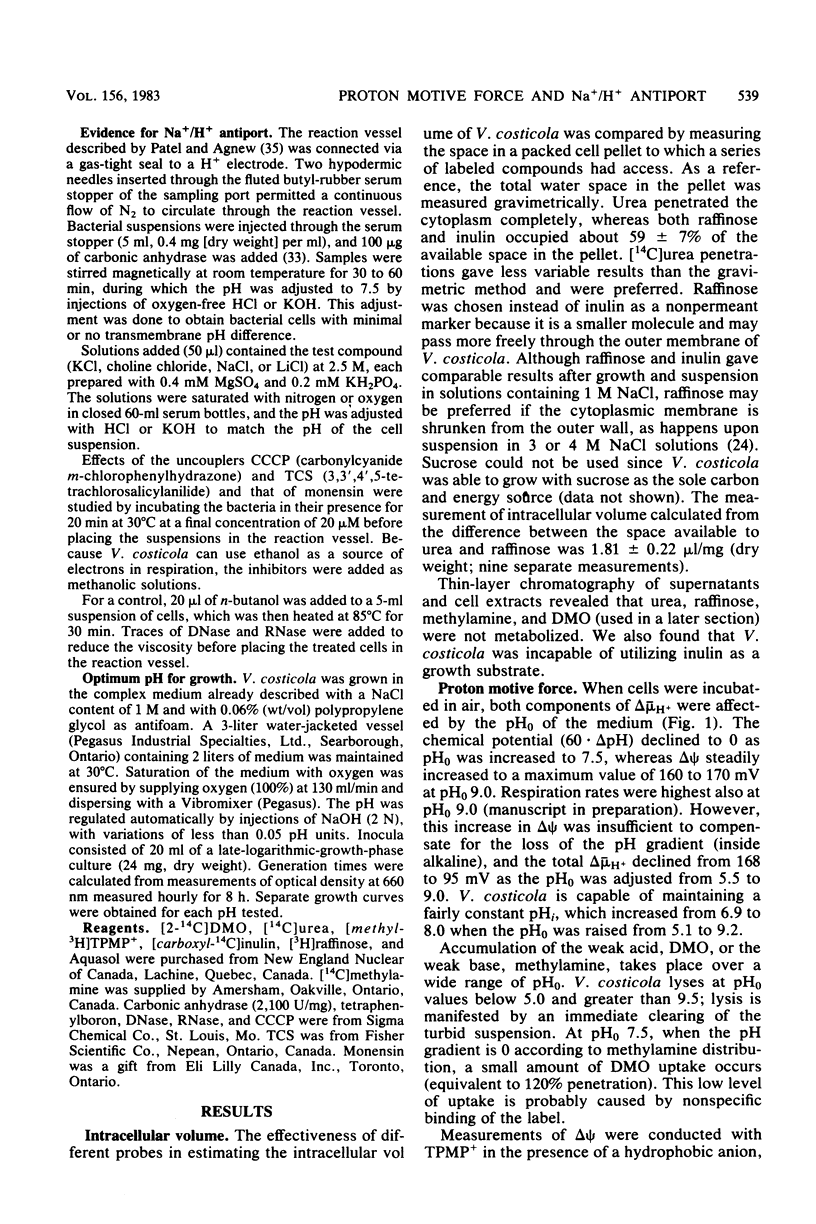
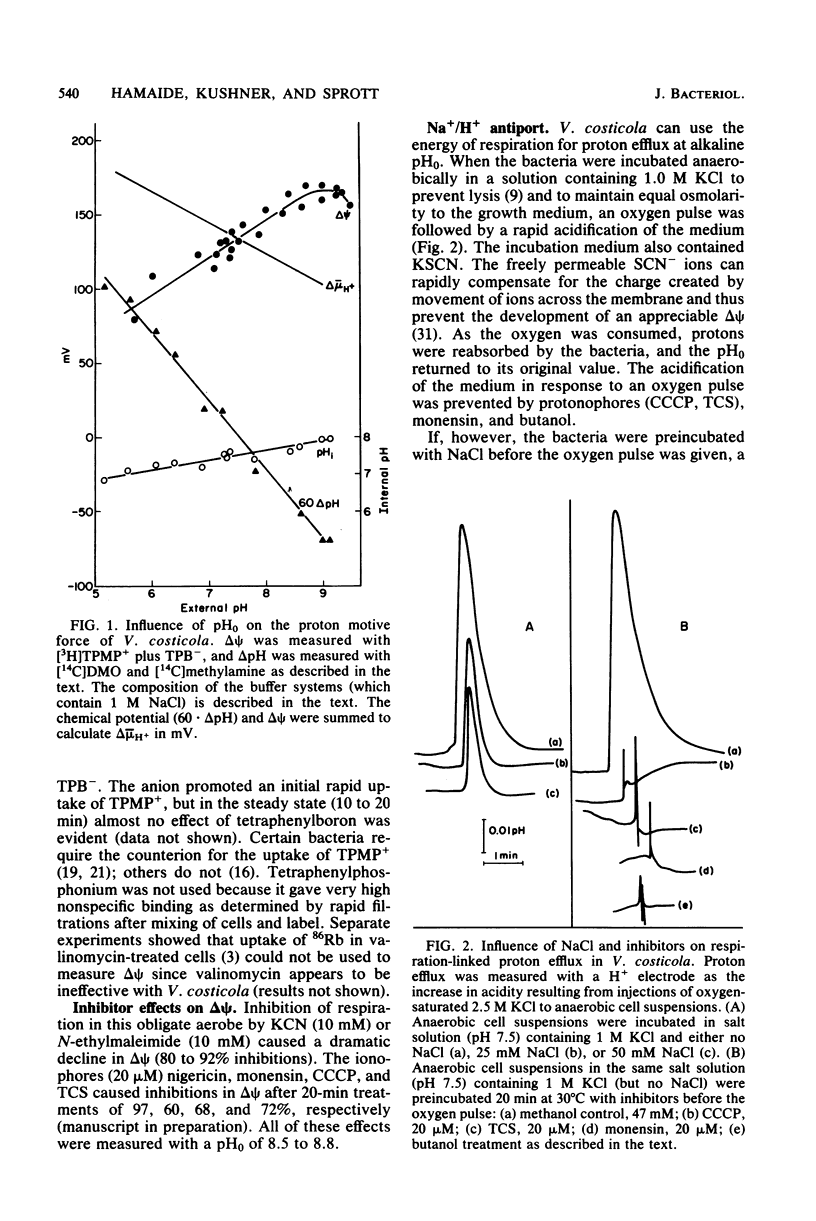
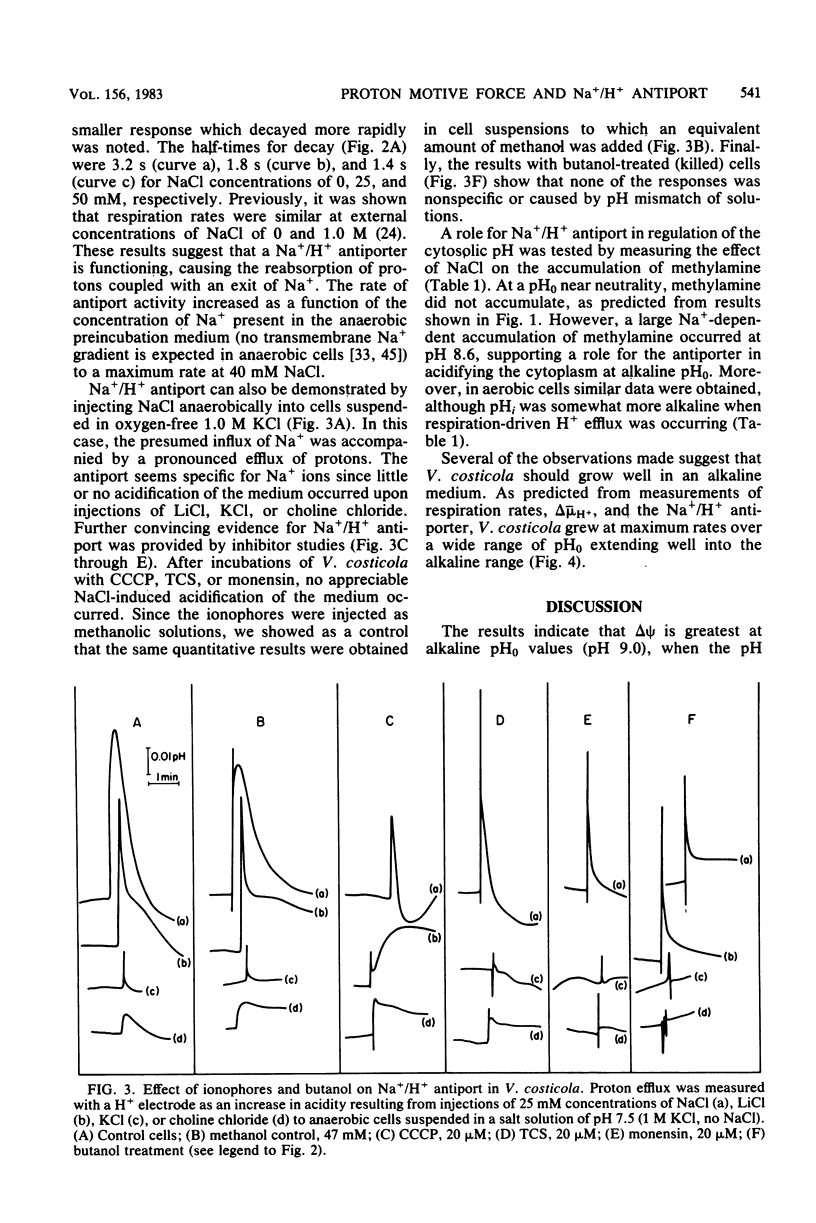
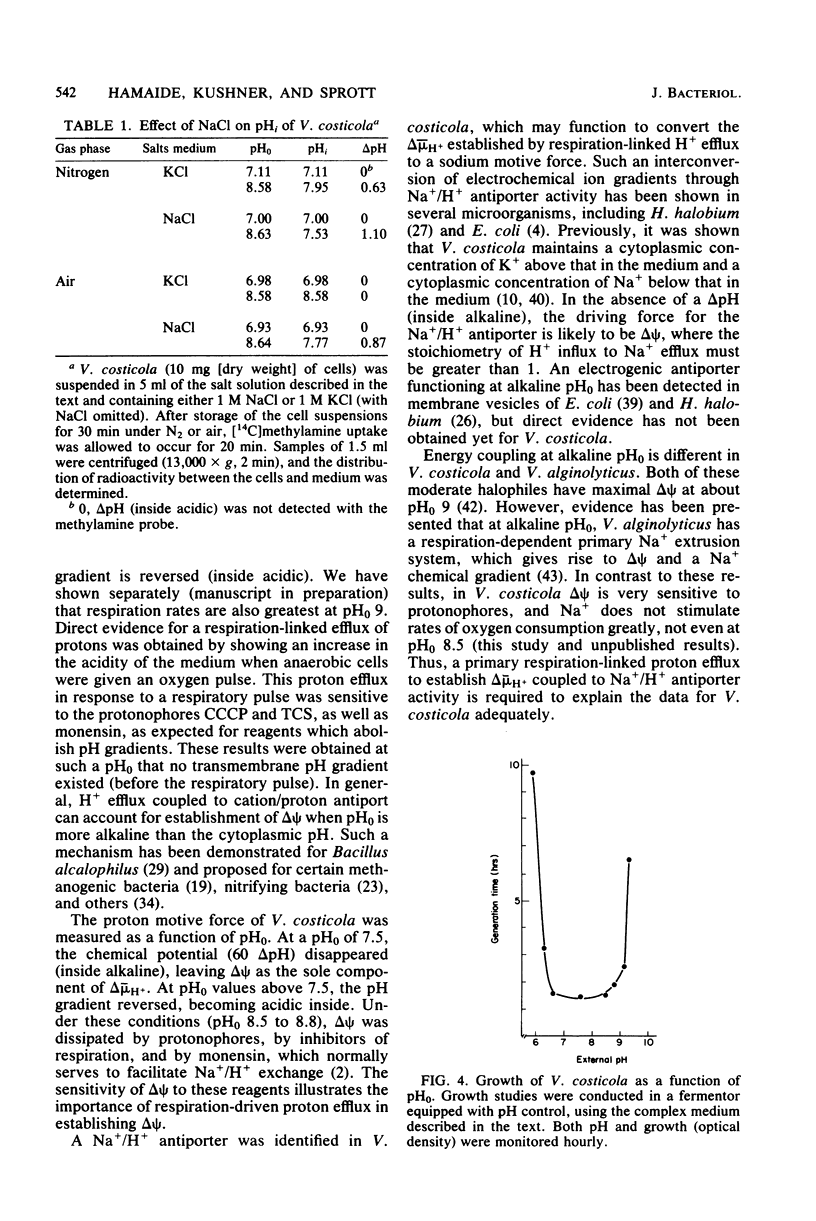
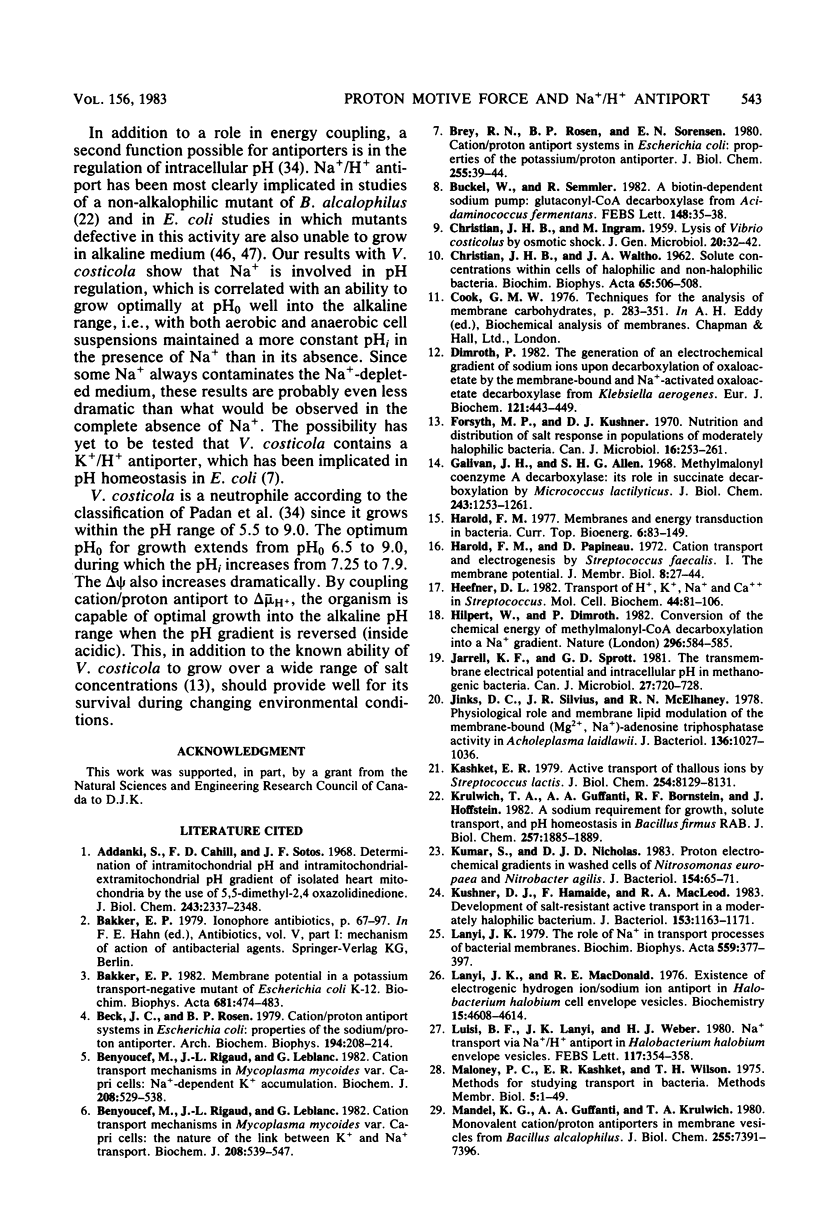
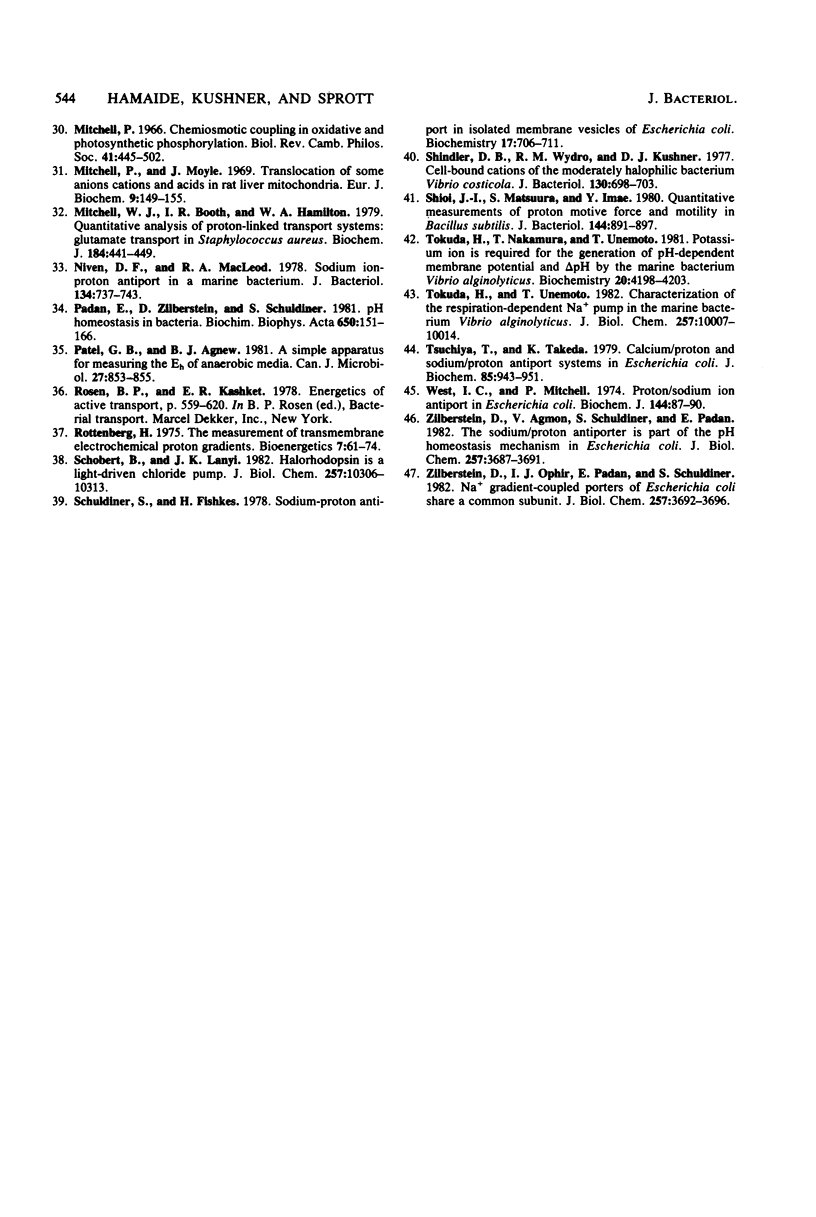
Selected References
These references are in PubMed. This may not be the complete list of references from this article.
- Addanki A., Cahill F. D., Sotos J. F. Determination of intramitochondrial pH and intramitochondrial-extramitochondrial pH gradient of isolated heart mitochondria by the use of 5,5-dimethyl-2,4-oxazolidinedione. I. Changes during respiration and adenosine triphosphate-dependent transport of Ca++, Mg++, and Zn++. J Biol Chem. 1968 May 10;243(9):2337–2348. [PubMed] [Google Scholar]
- Bakker E. P. Membrane potential in a potassium transport-negative mutant of Escherichia coli K-12. The distribution of rubidium in the presence of valinomycin indicates a higher potential than that of the tetraphenylphosphonium cation. Biochim Biophys Acta. 1982 Sep 15;681(3):474–483. doi: 10.1016/0005-2728(82)90190-6. [DOI] [PubMed] [Google Scholar]
- Beck J. C., Rosen B. P. Cation/proton antiport systems in escherichia coli: properties of the sodium/proton antiporter. Arch Biochem Biophys. 1979 Apr 15;194(1):208–214. doi: 10.1016/0003-9861(79)90611-8. [DOI] [PubMed] [Google Scholar]
- Benyoucef M., Rigaud J. L., Leblanc G. Cation transport mechanisms in Mycoplasma mycoides var. Capri cells. Na+-dependent K+ accumulation. Biochem J. 1982 Dec 15;208(3):529–538. doi: 10.1042/bj2080529. [DOI] [PMC free article] [PubMed] [Google Scholar]
- Benyoucef M., Rigaud J. L., Leblanc G. Cation transport mechanisms in Mycoplasma mycoides var. Capri cells. The nature of the link between K+ and Na+ transport. Biochem J. 1982 Dec 15;208(3):539–547. doi: 10.1042/bj2080539. [DOI] [PMC free article] [PubMed] [Google Scholar]
- Brey R. N., Rosen B. P., Sorensen E. N. Cation/proton antiport systems in Escherichia coli. Properties of the potassium/proton antiporter. J Biol Chem. 1980 Jan 10;255(1):39–44. [PubMed] [Google Scholar]
- Buckel W., Semmler R. A biotin-dependent sodium pump: glutaconyl-CoA decarboxylase from Acidaminococcus fermentans. FEBS Lett. 1982 Nov 1;148(1):35–38. doi: 10.1016/0014-5793(82)81237-4. [DOI] [PubMed] [Google Scholar]
- CHRISTIAN J. H., INGRAM M. Lysis of Vibrio costicolus by osmotic shock. J Gen Microbiol. 1959 Feb;20(1):32–42. doi: 10.1099/00221287-20-1-32. [DOI] [PubMed] [Google Scholar]
- CHRISTIAN J. H., WALTHO J. A. Solute concentrations within cells of halophilic and non-halophilic bacteria. Biochim Biophys Acta. 1962 Dec 17;65:506–508. doi: 10.1016/0006-3002(62)90453-5. [DOI] [PubMed] [Google Scholar]
- Dimroth P. The generation of an electrochemical gradient of sodium ions upon decarboxylation of oxaloacetate by the membrane-bound and Na+-activated oxaloacetate decarboxylase from Klebsiella aerogenes. Eur J Biochem. 1982 Jan;121(2):443–449. doi: 10.1111/j.1432-1033.1982.tb05807.x. [DOI] [PubMed] [Google Scholar]
- Forsyth M. P., Kushner D. J. Nutrition and distribution of salt response in populations of moderately halophilic bacteria. Can J Microbiol. 1970 Apr;16(4):253–261. doi: 10.1139/m70-047. [DOI] [PubMed] [Google Scholar]
- Galivan J. H., Allen S. H. Methylmalonyl coenzyme A decarboxylase. Its role in succinate decarboxylation by Micrococcus lactilyticus. J Biol Chem. 1968 Mar 25;243(6):1253–1261. [PubMed] [Google Scholar]
- Harold F. M., Papineau D. Cation transport and electrogenesis by Streptococcus faecalis. I. The membrane potential. J Membr Biol. 1972;8(1):27–44. doi: 10.1007/BF01868093. [DOI] [PubMed] [Google Scholar]
- Heefner D. L. Transport of H+, K+, Na+ and Ca++ in Streptococcus. Mol Cell Biochem. 1982 Apr 30;44(2):81–106. doi: 10.1007/BF00226893. [DOI] [PubMed] [Google Scholar]
- Hilpert W., Dimroth P. Conversion of the chemical energy of methylmalonyl-CoA decarboxylation into a Na+ gradient. Nature. 1982 Apr 8;296(5857):584–585. doi: 10.1038/296584a0. [DOI] [PubMed] [Google Scholar]
- Jarrell K. F., Sprott G. D. The transmembrane electrical potential and intracellular pH in methanogenic bacteria. Can J Microbiol. 1981 Jul;27(7):720–728. doi: 10.1139/m81-110. [DOI] [PubMed] [Google Scholar]
- Jinks D. C., Silvius J. R., McElhaney R. N. Physiological role and membrane lipid modulation of the membrane-bound (Mg2+, na+)-adenosine triphosphatase activity in Acholeplasma laidlawii. J Bacteriol. 1978 Dec;136(3):1027–1036. doi: 10.1128/jb.136.3.1027-1036.1978. [DOI] [PMC free article] [PubMed] [Google Scholar]
- Kashket E. R. Active transport of thallous ions by Streptococcus lactis. J Biol Chem. 1979 Sep 10;254(17):8129–8131. [PubMed] [Google Scholar]
- Krulwich T. A., Guffanti A. A., Bornstein R. F., Hoffstein J. A sodium requirement for growth, solute transport, and pH homeostasis in Bacillus firmus RAB. J Biol Chem. 1982 Feb 25;257(4):1885–1889. [PubMed] [Google Scholar]
- Kumar S., Nicholas D. J. Proton electrochemical gradients in washed cells of Nitrosomonas europaea and Nitrobacter agilis. J Bacteriol. 1983 Apr;154(1):65–71. doi: 10.1128/jb.154.1.65-71.1983. [DOI] [PMC free article] [PubMed] [Google Scholar]
- Kushner D. J., Hamaide F., MacLeod R. A. Development of salt-resistant active transport in a moderately halophilic bacterium. J Bacteriol. 1983 Mar;153(3):1163–1171. doi: 10.1128/jb.153.3.1163-1171.1983. [DOI] [PMC free article] [PubMed] [Google Scholar]
- Lanyi J. K., MacDonald R. E. Existence of electrogenic hydrogen ion/sodium ion antiport in Halobacterium halobium cell envelope vesicles. Biochemistry. 1976 Oct 19;15(21):4608–4614. doi: 10.1021/bi00666a010. [DOI] [PubMed] [Google Scholar]
- Lanyi J. K. The role of Na+ in transport processes of bacterial membranes. Biochim Biophys Acta. 1979 Dec 20;559(4):377–397. doi: 10.1016/0304-4157(79)90011-x. [DOI] [PubMed] [Google Scholar]
- Luisi B. F., Lanyi J. K., Weber H. J. Na+ transport via Na+/H+ antiport in Halobacterium halobium envelope vesicles. FEBS Lett. 1980 Aug 11;117(1):354–358. doi: 10.1016/0014-5793(80)80979-3. [DOI] [PubMed] [Google Scholar]
- Mandel K. G., Guffanti A. A., Krulwich T. A. Monovalent cation/proton antiporters in membrane vesicles from Bacillus alcalophilus. J Biol Chem. 1980 Aug 10;255(15):7391–7396. [PubMed] [Google Scholar]
- Mitchell P. Chemiosmotic coupling in oxidative and photosynthetic phosphorylation. Biol Rev Camb Philos Soc. 1966 Aug;41(3):445–502. doi: 10.1111/j.1469-185x.1966.tb01501.x. [DOI] [PubMed] [Google Scholar]
- Mitchell P., Moyle J. Translocation of some anions cations and acids in rat liver mitochondria. Eur J Biochem. 1969 Jun;9(2):149–155. doi: 10.1111/j.1432-1033.1969.tb00588.x. [DOI] [PubMed] [Google Scholar]
- Mitchell W. J., Booth I. R., Hamilton W. A. Quantitative analysis of proton-linked transport systems. Glutamate transport in Staphylococcus aureus. Biochem J. 1979 Nov 15;184(2):441–449. doi: 10.1042/bj1840441. [DOI] [PMC free article] [PubMed] [Google Scholar]
- Niven D. F., MacLeod R. A. Sodium ion-proton antiport in a marine bacterium. J Bacteriol. 1978 Jun;134(3):737–743. doi: 10.1128/jb.134.3.737-743.1978. [DOI] [PMC free article] [PubMed] [Google Scholar]
- Padan E., Zilberstein D., Schuldiner S. pH homeostasis in bacteria. Biochim Biophys Acta. 1981 Dec;650(2-3):151–166. doi: 10.1016/0304-4157(81)90004-6. [DOI] [PubMed] [Google Scholar]
- Patel G. B., Agnew B. J. A simple apparatus for measuring the Eh of anaerobic media. Can J Microbiol. 1981 Aug;27(8):853–855. doi: 10.1139/m81-134. [DOI] [PubMed] [Google Scholar]
- Rottenberg H. The measurement of transmembrane electrochemical proton gradients. J Bioenerg. 1975 May;7(2):61–74. doi: 10.1007/BF01558427. [DOI] [PubMed] [Google Scholar]
- Schobert B., Lanyi J. K. Halorhodopsin is a light-driven chloride pump. J Biol Chem. 1982 Sep 10;257(17):10306–10313. [PubMed] [Google Scholar]
- Schuldiner S., Fishkes H. Sodium-proton antiport in isolated membrane vesicles of Escherichia coli. Biochemistry. 1978 Feb 21;17(4):706–711. doi: 10.1021/bi00597a023. [DOI] [PubMed] [Google Scholar]
- Shindler D. B., Wydro R. M., Kushner D. J. Cell-bound cations of the moderately halophilic bacterium Vibrio costicola. J Bacteriol. 1977 May;130(2):698–703. doi: 10.1128/jb.130.2.698-703.1977. [DOI] [PMC free article] [PubMed] [Google Scholar]
- Shioi J. I., Matsuura S., Imae Y. Quantitative measurements of proton motive force and motility in Bacillus subtilis. J Bacteriol. 1980 Dec;144(3):891–897. doi: 10.1128/jb.144.3.891-897.1980. [DOI] [PMC free article] [PubMed] [Google Scholar]
- Tokuda H., Nakamura T., Unemoto T. Potassium ion is required for the generation of pH-dependent membrane potential and delta pH by the marine bacterium Vibrio alginolyticus. Biochemistry. 1981 Jul 7;20(14):4198–4203. doi: 10.1021/bi00517a038. [DOI] [PubMed] [Google Scholar]
- Tokuda H., Unemoto T. Characterization of the respiration-dependent Na+ pump in the marine bacterium Vibrio alginolyticus. J Biol Chem. 1982 Sep 10;257(17):10007–10014. [PubMed] [Google Scholar]
- Tsuchiya T., Takeda K. Calcium/proton and sodium/proton antiport systems in Escherichia coli. J Biochem. 1979 Apr;85(4):943–951. doi: 10.1093/oxfordjournals.jbchem.a132426. [DOI] [PubMed] [Google Scholar]
- West I. C., Mitchell P. Proton/sodium ion antiport in Escherichia coli. Biochem J. 1974 Oct;144(1):87–90. doi: 10.1042/bj1440087. [DOI] [PMC free article] [PubMed] [Google Scholar]
- Zilberstein D., Agmon V., Schuldiner S., Padan E. The sodium/proton antiporter is part of the pH homeostasis mechanism in Escherichia coli. J Biol Chem. 1982 Apr 10;257(7):3687–3691. [PubMed] [Google Scholar]
- Zilberstein D., Ophir I. J., Padan E., Schuldiner S. Na+ gradient-coupled porters of EScherichia coli share a common subunit. J Biol Chem. 1982 Apr 10;257(7):3692–3696. [PubMed] [Google Scholar]