Abstract
The membrane-bound ATPase of Mycoplasma gallisepticum selectively hydrolyzed purine nucleoside triphosphates and dATP. ADP, although not a substrate, inhibited ATP hydrolysis. The enzyme exhibited a pH optimum of 7.0 to 7.5 and an obligatory requirement for divalent cations. Dicyclohexylcarbodiimide at a concentration of 1 mM inhibited 95% of the ATPase activity at 37 degrees C, with 50% inhibition occurring at 22 microM dicyclohexylcarbodiimide. Sodium or potassium (or both) failed to stimulate activity by greater than 37%. Azide (2.6 mM), diethylstilbestrol (100 micrograms/ml), p-chloromercuribenzoate (1 mM), and vanadate (50 microM) inhibited 50, 91, 89, and 60%, respectively. The ATPase activity could not be removed from the membrane without detergent solubilization. Although most detergents inactivated the enzyme, the dipolar ionic detergent N-dodecyl-N,N-dimethyl-3-ammonio-1-propanesulfonate (0.1%) solubilized approximately 70% of the enzyme with only a minor loss in activity. The extraction led to a twofold increase in specific activity and retention of inhibition by dicyclohexylcarbodiimide and ADP. Glycerol greatly increased the stability of the solubilized enzyme. The properties of the membrane-bound ATPase are not consistent with any known ATPase. We postulate that the ATPase functions as an electrogenic proton pump.
Full text
PDF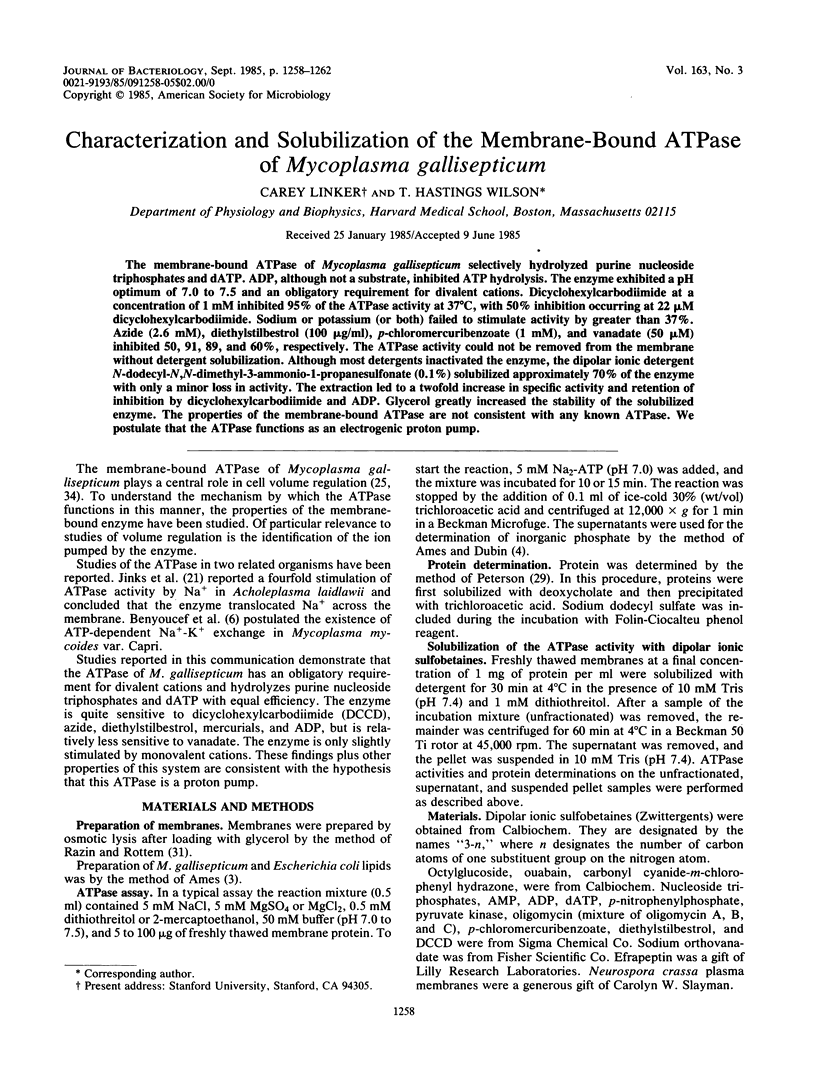
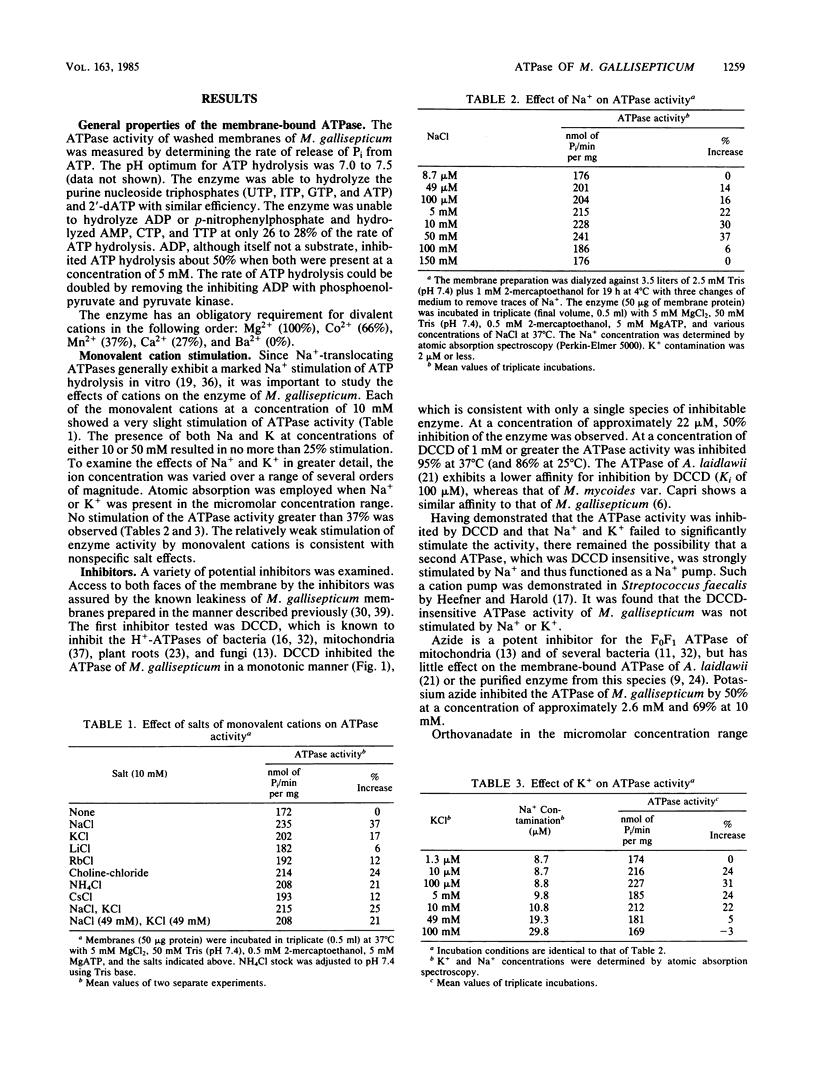
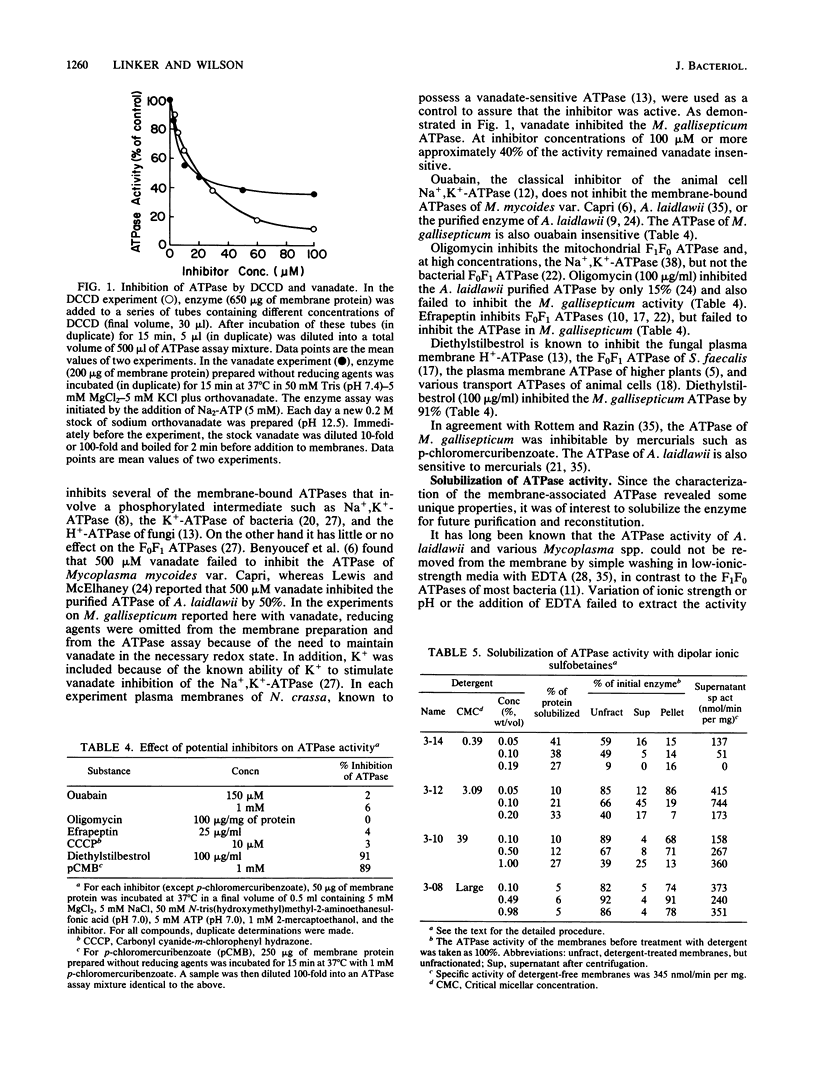
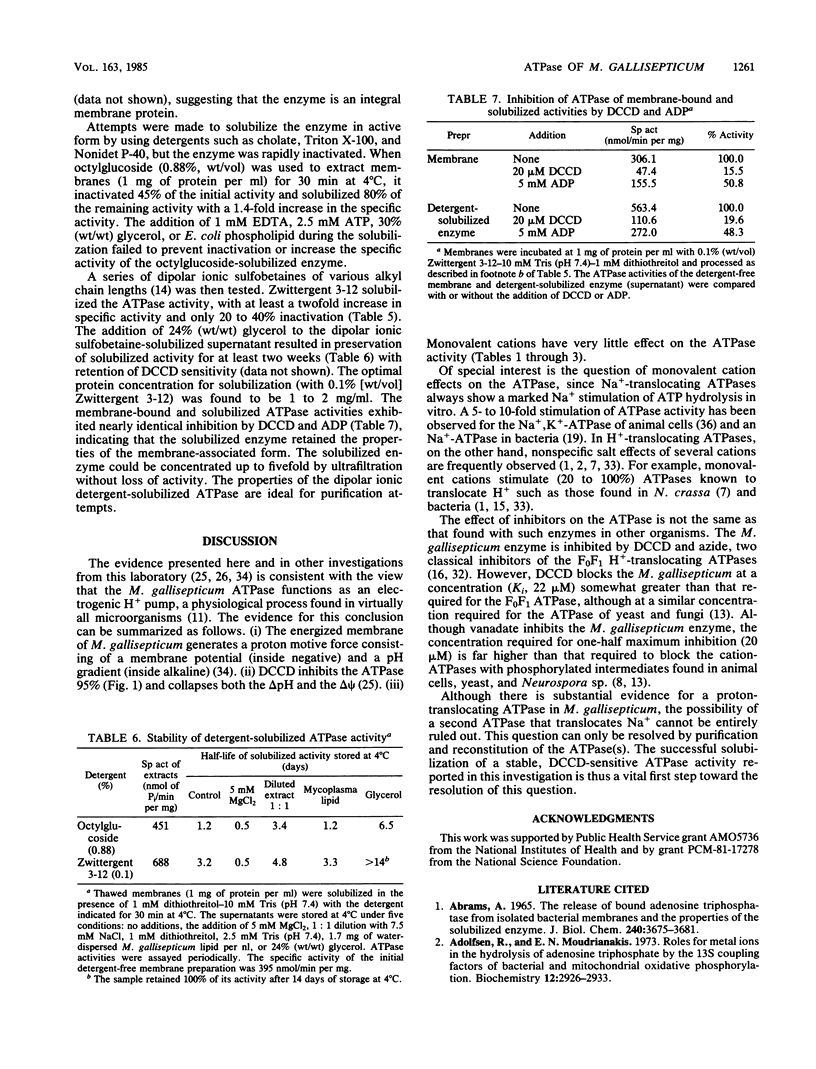
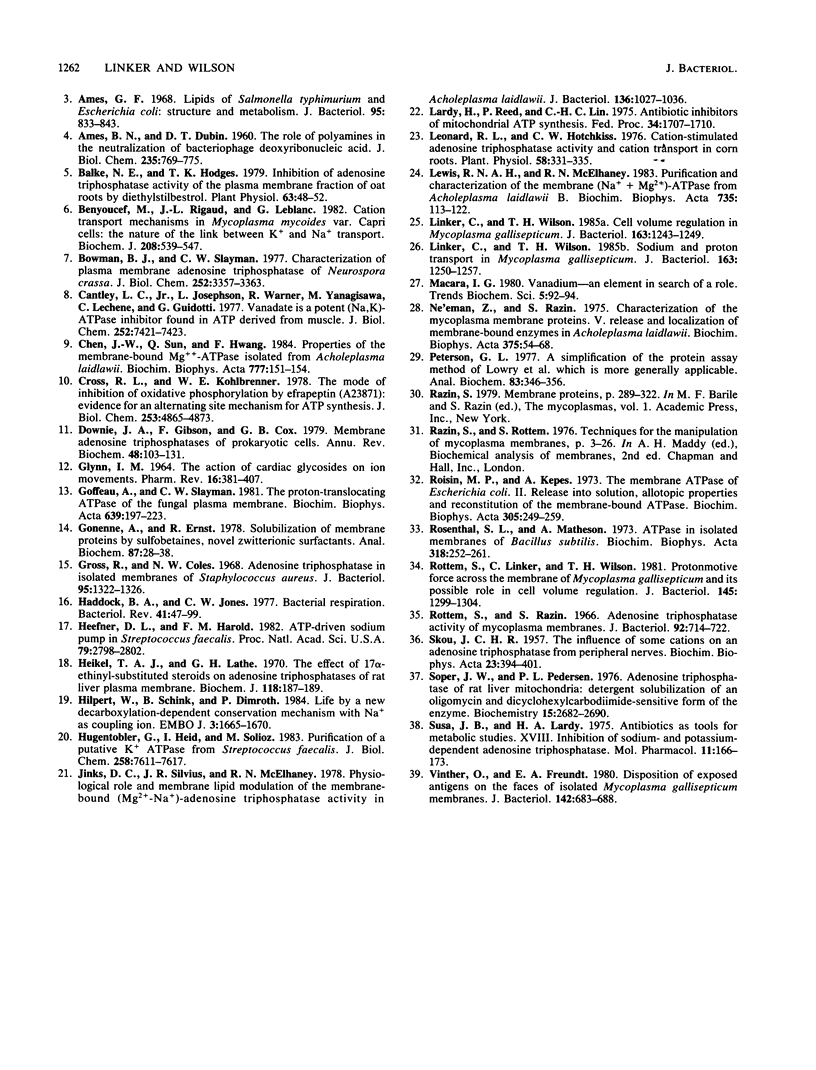
Selected References
These references are in PubMed. This may not be the complete list of references from this article.
- AMES B. N., DUBIN D. T. The role of polyamines in the neutralization of bacteriophage deoxyribonucleic acid. J Biol Chem. 1960 Mar;235:769–775. [PubMed] [Google Scholar]
- Abrams A. The release of bound adenosine triphosphatase from isolated bacterial membranes and the properties of the solubilized enzyme. J Biol Chem. 1965 Sep;240(9):3675–3681. [PubMed] [Google Scholar]
- Adolfsen R., Moudrianakis E. N. Roles for metal ions in the hydrolysis of adenosine triphosphate by the 13S coupling factors of bacterial and mitochondrial oxidative phosphorylation. Biochemistry. 1973 Jul 17;12(15):2926–2933. doi: 10.1021/bi00739a024. [DOI] [PubMed] [Google Scholar]
- Ames G. F. Lipids of Salmonella typhimurium and Escherichia coli: structure and metabolism. J Bacteriol. 1968 Mar;95(3):833–843. doi: 10.1128/jb.95.3.833-843.1968. [DOI] [PMC free article] [PubMed] [Google Scholar]
- Balke N. E., Hodges T. K. Inhibition of adenosine triphosphatase activity of the plasma membrane fraction of oat roots by diethylstilbestrol. Plant Physiol. 1979 Jan;63(1):48–52. doi: 10.1104/pp.63.1.48. [DOI] [PMC free article] [PubMed] [Google Scholar]
- Benyoucef M., Rigaud J. L., Leblanc G. Cation transport mechanisms in Mycoplasma mycoides var. Capri cells. The nature of the link between K+ and Na+ transport. Biochem J. 1982 Dec 15;208(3):539–547. doi: 10.1042/bj2080539. [DOI] [PMC free article] [PubMed] [Google Scholar]
- Bowman B. J., Slayman C. W. Characterization of plasma membrane adenosine triphosphatase of Neurospora crassa. J Biol Chem. 1977 May 25;252(10):3357–3363. [PubMed] [Google Scholar]
- Cantley L. C., Jr, Josephson L., Warner R., Yanagisawa M., Lechene C., Guidotti G. Vanadate is a potent (Na,K)-ATPase inhibitor found in ATP derived from muscle. J Biol Chem. 1977 Nov 10;252(21):7421–7423. [PubMed] [Google Scholar]
- Chen J. W., Sun Q., Hwang F. Properties of the membrane-bound Mg2+-ATPase isolated from Acholeplasma laidlawii. Biochim Biophys Acta. 1984 Oct 17;777(1):151–154. doi: 10.1016/0005-2736(84)90508-x. [DOI] [PubMed] [Google Scholar]
- Cross R. L., Kohlbrenner W. E. The mode of inhibition of oxidative phosphorylation by efrapeptin (A23871). Evidence for an alternating site mechanism for ATP synthesis. J Biol Chem. 1978 Jul 25;253(14):4865–4873. [PubMed] [Google Scholar]
- Downie J. A., Gibson F., Cox G. B. Membrane adenosine triphosphatases of prokaryotic cells. Annu Rev Biochem. 1979;48:103–131. doi: 10.1146/annurev.bi.48.070179.000535. [DOI] [PubMed] [Google Scholar]
- GLYNN I. M. THE ACTION OF CARDIAC GLYCOSIDES ON ION MOVEMENTS. Pharmacol Rev. 1964 Dec;16:381–407. [PubMed] [Google Scholar]
- Goffeau A., Slayman C. W. The proton-translocating ATPase of the fungal plasma membrane. Biochim Biophys Acta. 1981 Dec 30;639(3-4):197–223. doi: 10.1016/0304-4173(81)90010-0. [DOI] [PubMed] [Google Scholar]
- Gonenne A., Ernst R. Solubilization of membrane proteins by sulfobetaines, novel zwitterionic surfactants. Anal Biochem. 1978 Jun 15;87(1):28–38. doi: 10.1016/0003-2697(78)90565-1. [DOI] [PubMed] [Google Scholar]
- Gross R., Coles N. W. Adenosine triphosphatase in isolated membranes of Staphylococcus aureus. J Bacteriol. 1968 Apr;95(4):1322–1326. doi: 10.1128/jb.95.4.1322-1326.1968. [DOI] [PMC free article] [PubMed] [Google Scholar]
- Haddock B. A., Jones C. W. Bacterial respiration. Bacteriol Rev. 1977 Mar;41(1):47–99. doi: 10.1128/br.41.1.47-99.1977. [DOI] [PMC free article] [PubMed] [Google Scholar]
- Heefner D. L., Harold F. M. ATP-driven sodium pump in Streptococcus faecalis. Proc Natl Acad Sci U S A. 1982 May;79(9):2798–2802. doi: 10.1073/pnas.79.9.2798. [DOI] [PMC free article] [PubMed] [Google Scholar]
- Heikel T. A., Lathe G. H. The effect of 17-alpha-ethinyl-substituted steroids on adenosine triphosphatases of rat liver plasma membrane. Biochem J. 1970 Jun;118(1):187–189. doi: 10.1042/bj1180187. [DOI] [PMC free article] [PubMed] [Google Scholar]
- Hilpert W., Schink B., Dimroth P. Life by a new decarboxylation-dependent energy conservation mechanism with Na as coupling ion. EMBO J. 1984 Aug;3(8):1665–1670. doi: 10.1002/j.1460-2075.1984.tb02030.x. [DOI] [PMC free article] [PubMed] [Google Scholar]
- Hugentobler G., Heid I., Solioz M. Purification of a putative K+-ATPase from Streptococcus faecalis. J Biol Chem. 1983 Jun 25;258(12):7611–7617. [PubMed] [Google Scholar]
- Jinks D. C., Silvius J. R., McElhaney R. N. Physiological role and membrane lipid modulation of the membrane-bound (Mg2+, na+)-adenosine triphosphatase activity in Acholeplasma laidlawii. J Bacteriol. 1978 Dec;136(3):1027–1036. doi: 10.1128/jb.136.3.1027-1036.1978. [DOI] [PMC free article] [PubMed] [Google Scholar]
- Lardy H., Reed P., Lin C. H. Antibiotic inhibitors of mitochondrial ATP synthesis. Fed Proc. 1975 Jul;34(8):1707–1710. [PubMed] [Google Scholar]
- Leonard R. T., Hotchkiss C. W. Cation-stimulated Adenosine Triphosphatase Activity and Cation Transport in Corn Roots. Plant Physiol. 1976 Sep;58(3):331–335. doi: 10.1104/pp.58.3.331. [DOI] [PMC free article] [PubMed] [Google Scholar]
- Lewis R. N., McElhaney R. N. Purification and characterization of the membrane (Na+ + Mg2+)-ATPase from Acholeplasma laidlawii B. Biochim Biophys Acta. 1983 Oct 26;735(1):113–122. doi: 10.1016/0005-2736(83)90266-3. [DOI] [PubMed] [Google Scholar]
- Linker C., Wilson T. H. Cell volume regulation in Mycoplasma gallisepticum. J Bacteriol. 1985 Sep;163(3):1243–1249. doi: 10.1128/jb.163.3.1243-1249.1985. [DOI] [PMC free article] [PubMed] [Google Scholar]
- Linker C., Wilson T. H. Sodium and proton transport in Mycoplasma gallisepticum. J Bacteriol. 1985 Sep;163(3):1250–1257. doi: 10.1128/jb.163.3.1250-1257.1985. [DOI] [PMC free article] [PubMed] [Google Scholar]
- Ne'eman Z., Razin S. Characterization of the mycoplasma membrane proteins. V. Release and localization of membrane-bound enzymes in Acholeplasma laidlawii. Biochim Biophys Acta. 1975 Jan 14;375(1):54–68. doi: 10.1016/0005-2736(75)90072-3. [DOI] [PubMed] [Google Scholar]
- Peterson G. L. A simplification of the protein assay method of Lowry et al. which is more generally applicable. Anal Biochem. 1977 Dec;83(2):346–356. doi: 10.1016/0003-2697(77)90043-4. [DOI] [PubMed] [Google Scholar]
- Roisin M. P., Kepes A. The membrane ATPase of Escherichia coli. I. Release into solution, allotopic properties and reconstitution of membrane-bound ATPase. Biochim Biophys Acta. 1973 May 30;305(2):249–259. doi: 10.1016/0005-2728(73)90173-4. [DOI] [PubMed] [Google Scholar]
- Rosenthal S. L., Matheson A. ATPase in isolated membranes of Bacillus subtilis. Biochim Biophys Acta. 1973 Aug 22;318(2):252–261. doi: 10.1016/0005-2736(73)90118-1. [DOI] [PubMed] [Google Scholar]
- Rottem S., Linker C., Wilson T. H. Proton motive force across the membrane of Mycoplasma gallisepticum and its possible role in cell volume regulation. J Bacteriol. 1981 Mar;145(3):1299–1304. doi: 10.1128/jb.145.3.1299-1304.1981. [DOI] [PMC free article] [PubMed] [Google Scholar]
- Rottem S., Razin S. Adenosine triphosphatase activity of mycoplasma membranes. J Bacteriol. 1966 Sep;92(3):714–722. doi: 10.1128/jb.92.3.714-722.1966. [DOI] [PMC free article] [PubMed] [Google Scholar]
- SKOU J. C. The influence of some cations on an adenosine triphosphatase from peripheral nerves. Biochim Biophys Acta. 1957 Feb;23(2):394–401. doi: 10.1016/0006-3002(57)90343-8. [DOI] [PubMed] [Google Scholar]
- Soper J. W., Pedersen P. L. Adenosine triphosphatase of rat liver mitochondria: detergent solubilization of an oligomycin- and dicyclohexylcarbodiimide-sensitive form of the enzyme. Biochemistry. 1976 Jun 15;15(12):2682–2690. doi: 10.1021/bi00657a031. [DOI] [PubMed] [Google Scholar]
- Susa J. B., Lardy H. A. Antibiotics as tools for metabolic studies. XVIII. Inhibition of sodium- and potassium-dependent adenosine triphosphatase. Mol Pharmacol. 1975 Mar;11(2):166–173. [PubMed] [Google Scholar]
- Vinther O., Freundt E. A. Disposition of exposed antigens on the faces of isolated Mycoplasma gallisepticum membranes. J Bacteriol. 1980 May;142(2):683–688. doi: 10.1128/jb.142.2.683-688.1980. [DOI] [PMC free article] [PubMed] [Google Scholar]