Abstract
We isolated pleiotropic mutants of Klebsiella aerogenes with the transposon Tn5 which were unable to utilize a variety of poor sources of nitrogen. The mutation responsible was shown to be in the asnB gene, one of two genes coding for an asparagine synthetase. Mutations in both asnA and asnB were necessary to produce an asparagine requirement. Assays which could distinguish the two asparagine synthetase activities were developed in strains missing a high-affinity asparaginase. The asnA and asnB genes coded for ammonia-dependent and glutamine-dependent asparagine synthetases, respectively. Asparagine repressed both enzymes. When growth was nitrogen limited, the level of the ammonia-dependent enzyme was low and that of the glutamine-dependent enzyme was high. The reverse was true in a nitrogen-rich (ammonia-containing) medium. Furthermore, mutations in the glnG protein, a regulatory component of the nitrogen assimilatory system, increased the level of the ammonia-dependent enzyme. The glutamine-dependent asparagine synthetase was purified to 95%. It was a tetramer with four equal 57,000-dalton subunits and catalyzed the stoichiometric generation of asparagine, AMP, and inorganic pyrophosphate from aspartate, ATP, and glutamine. High levels of ammonium chloride (50 mM) could replace glutamine. The purified enzyme exhibited a substrate-independent glutaminase activity which was probably an artifact of purification. The tetramer could be dissociated; the monomer possessed the high ammonia-dependent activity and the glutaminase activity, but not the glutamine-dependent activity. In contrast, the purified ammonia-dependent asparagine synthetase, about 40% pure, had a molecular weight of 80,000 and is probably a dimer of identical subunits. Asparagine inhibited both enzymes. Kinetic constants and the effect of pH, substrate, and product analogs were determined. The regulation and biochemistry of the asparagine synthetases prove the hypothesis strongly suggested by the genetic and physiological evidence that a glutamine-dependent enzyme is essential for asparagine synthesis when the nitrogen source is growth rate limiting.
Full text
PDF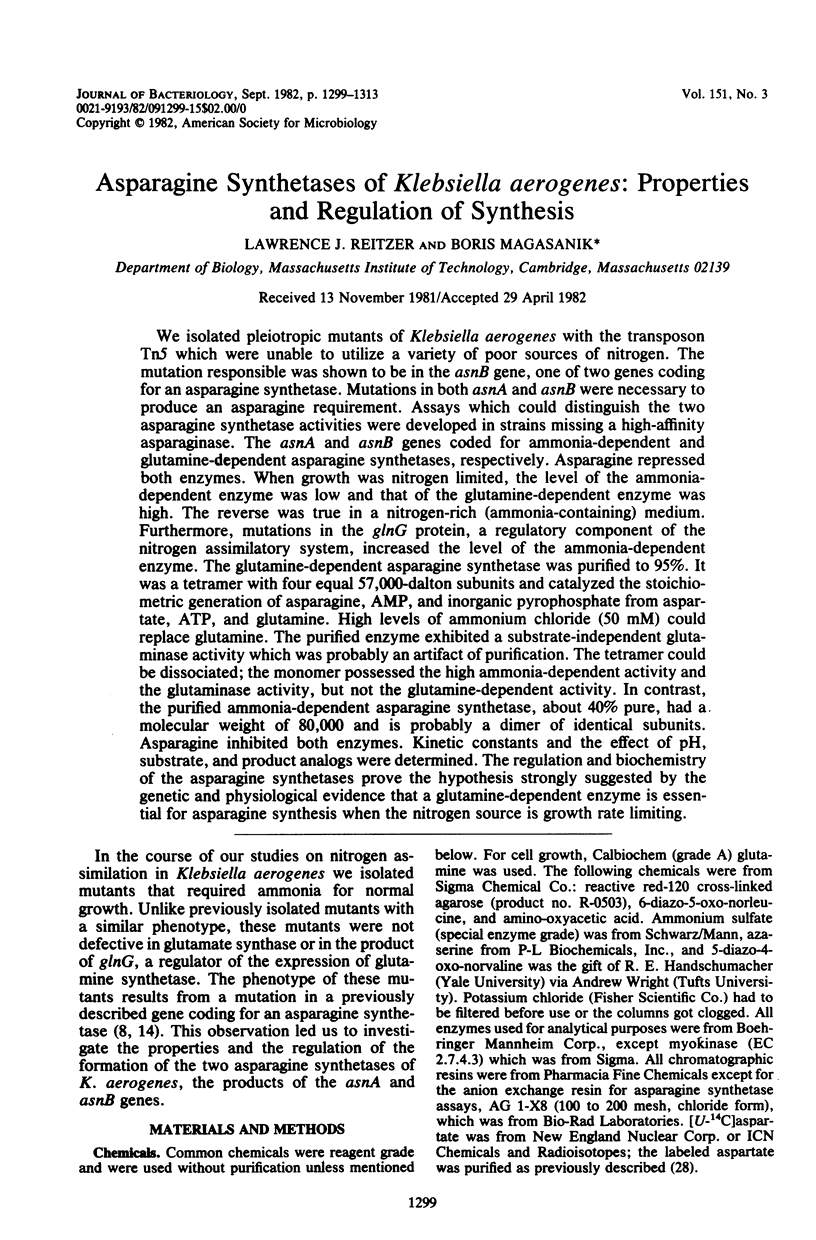
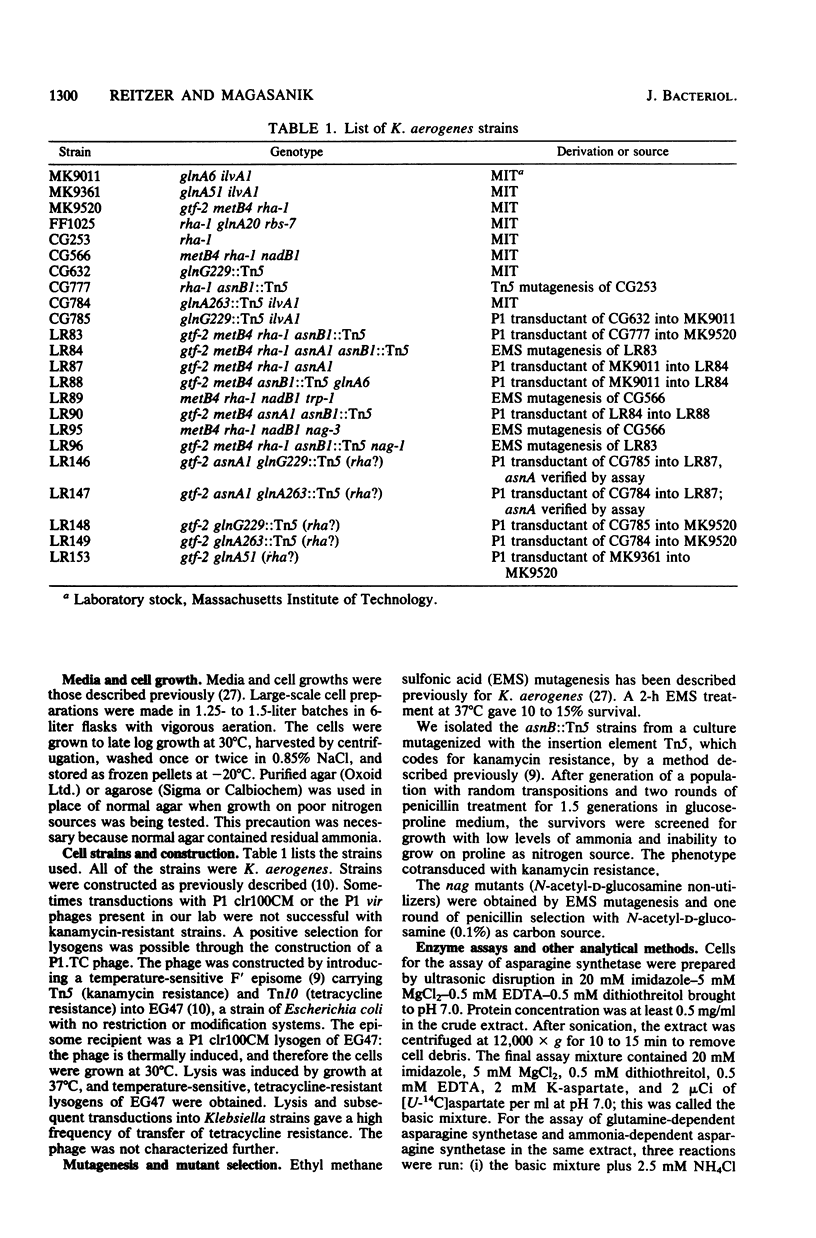
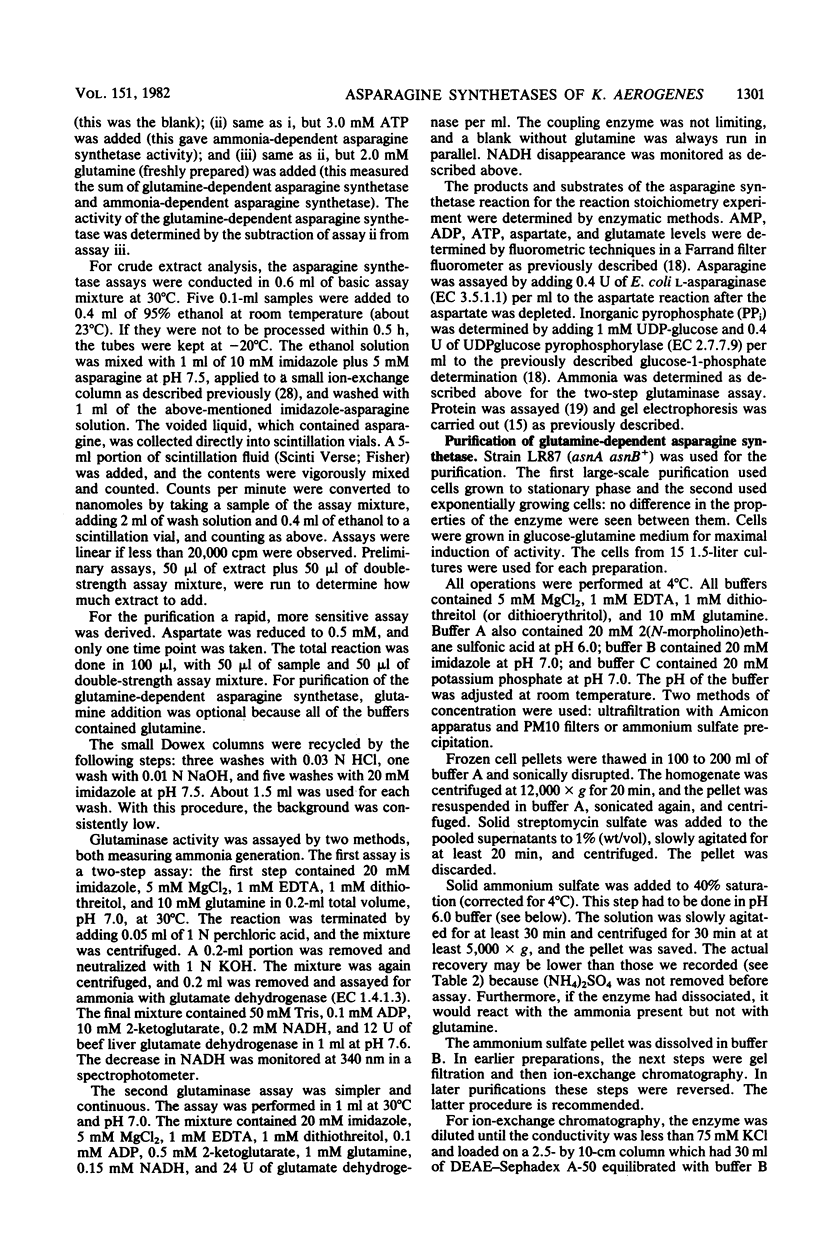
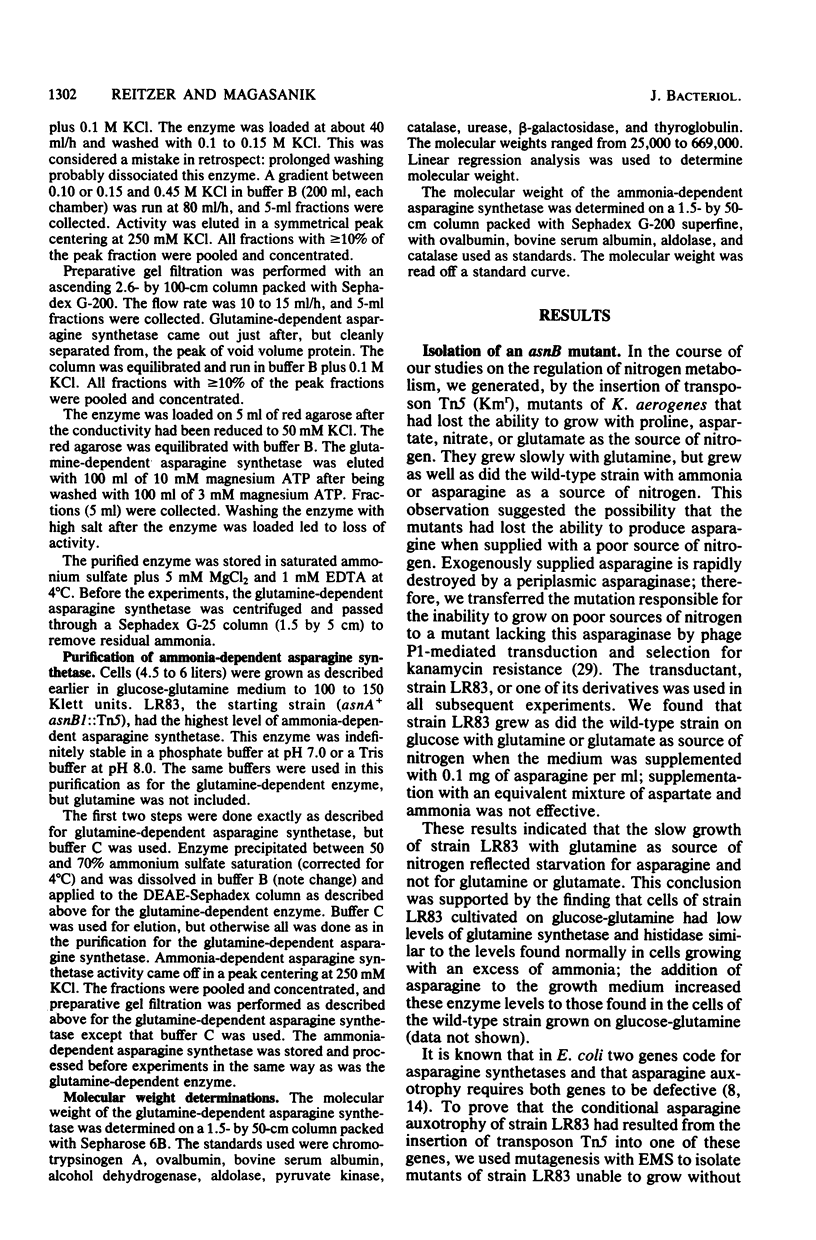
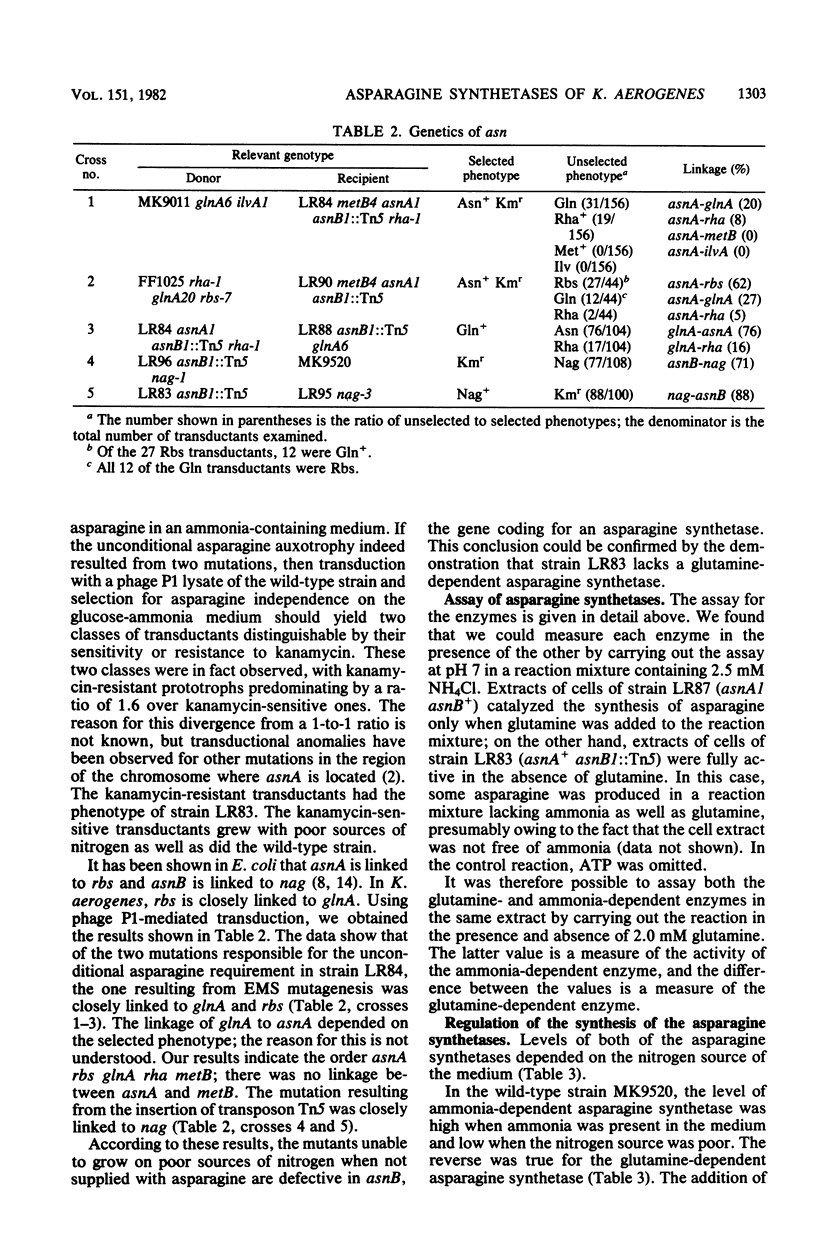
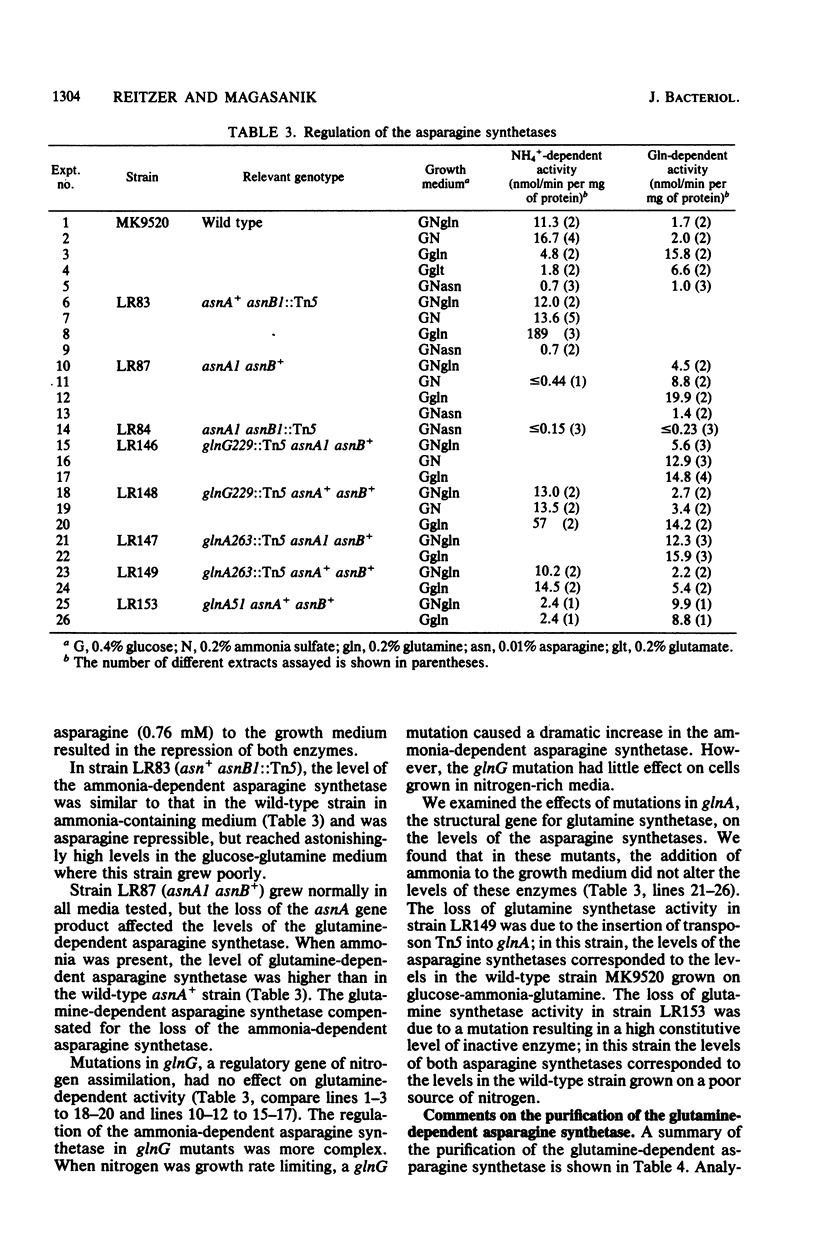
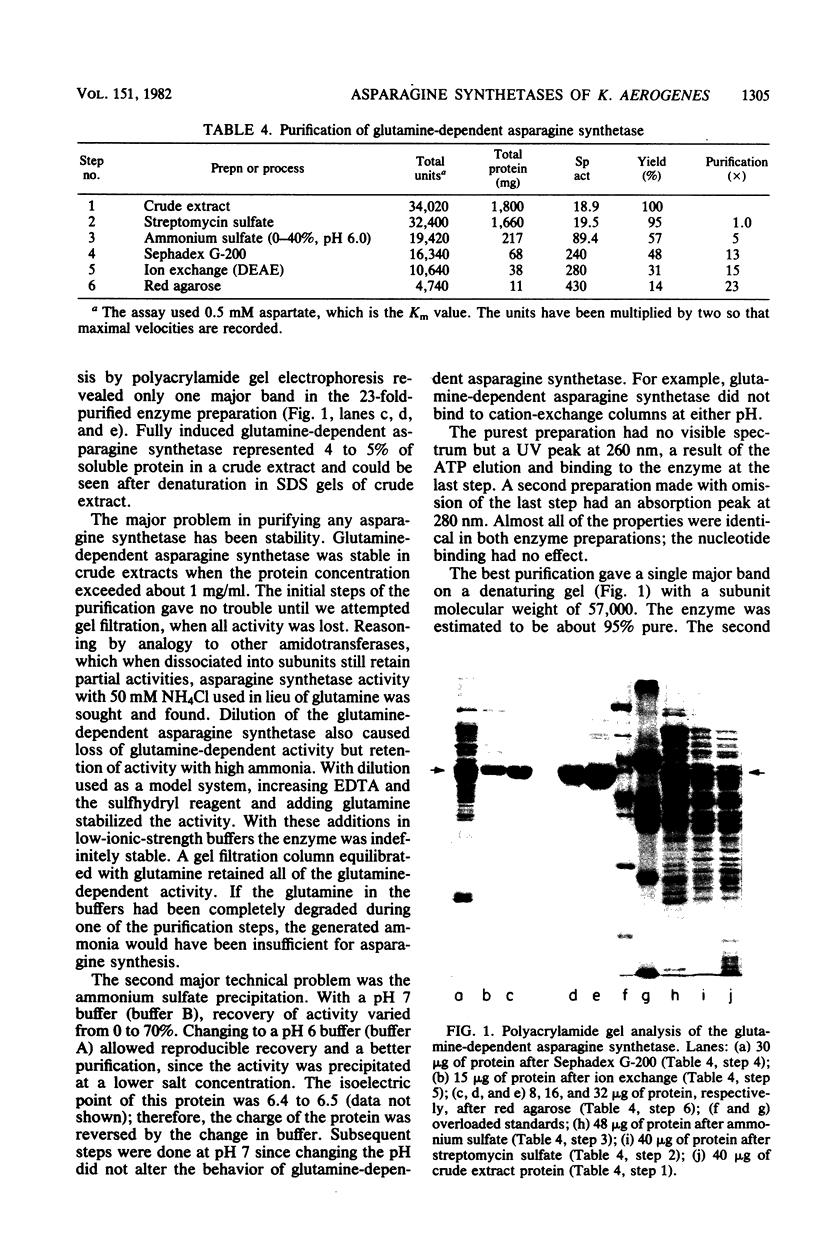
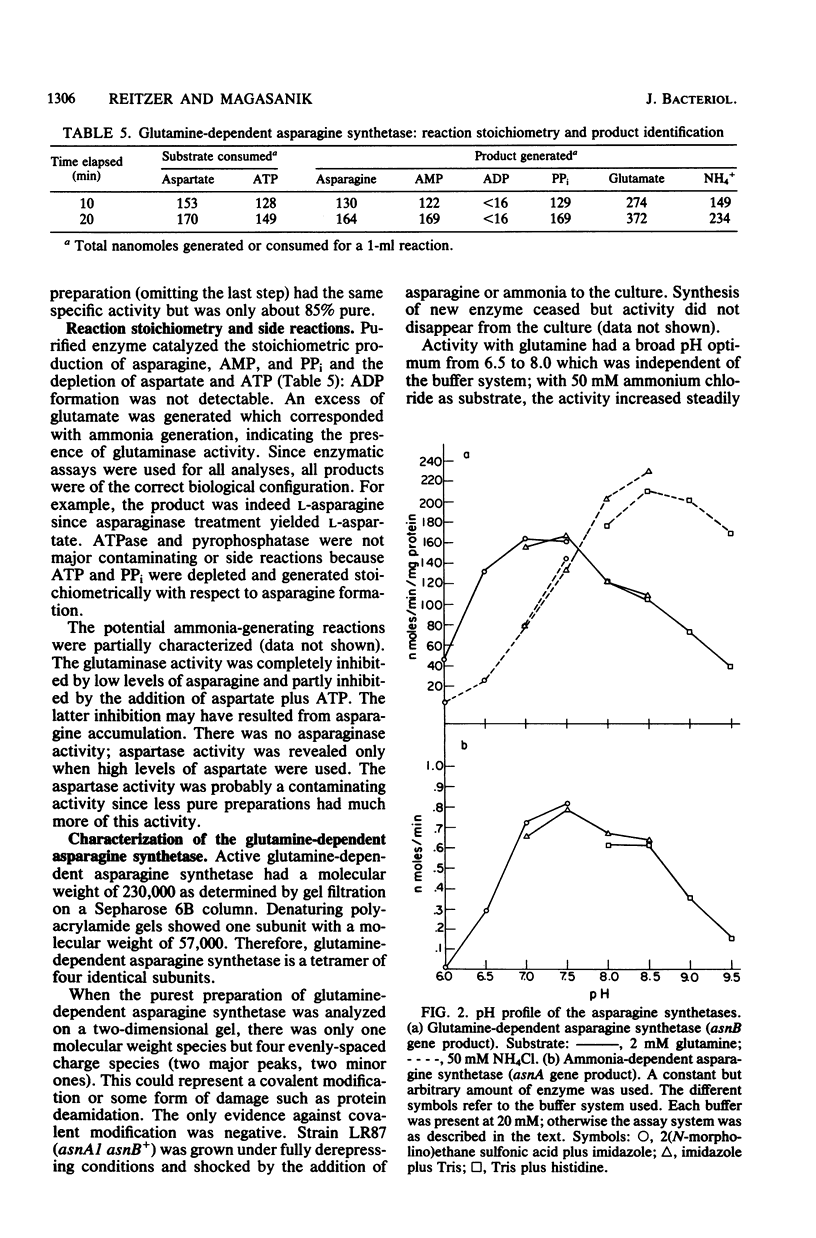
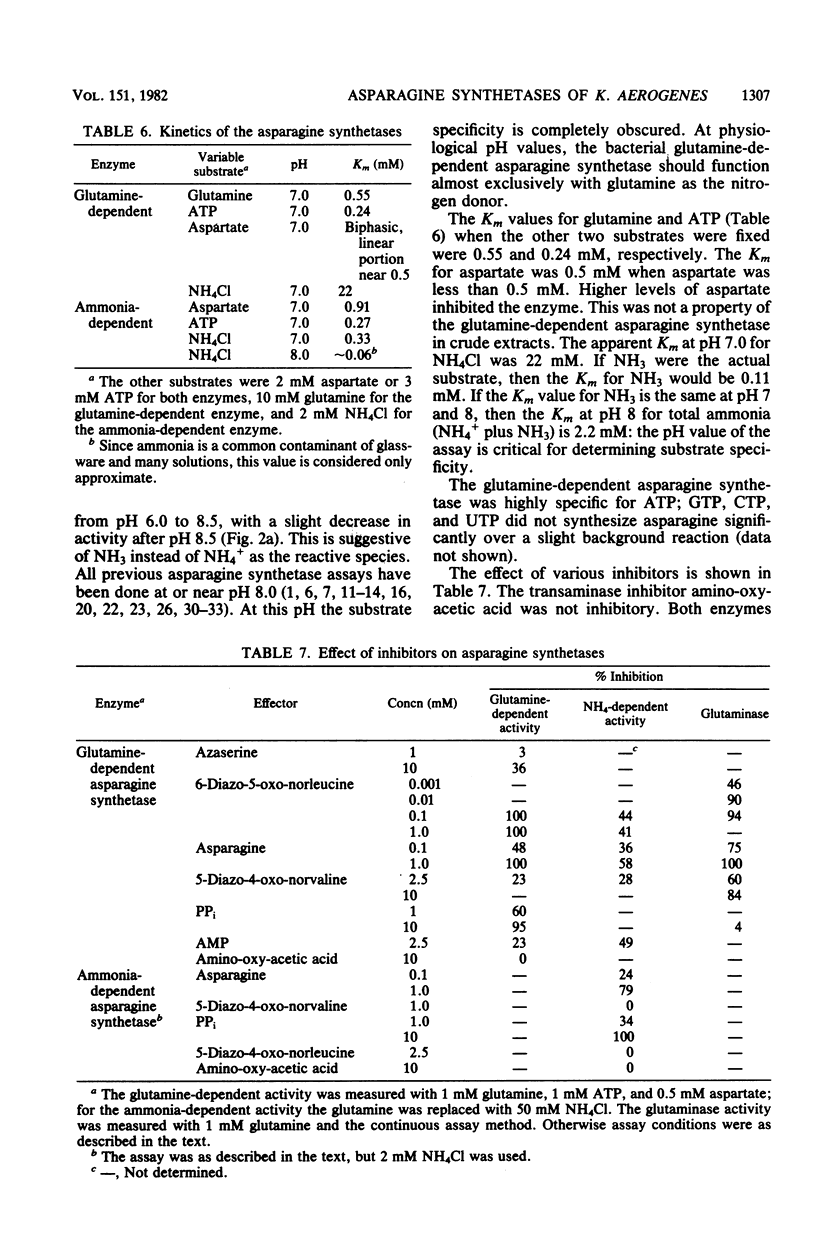
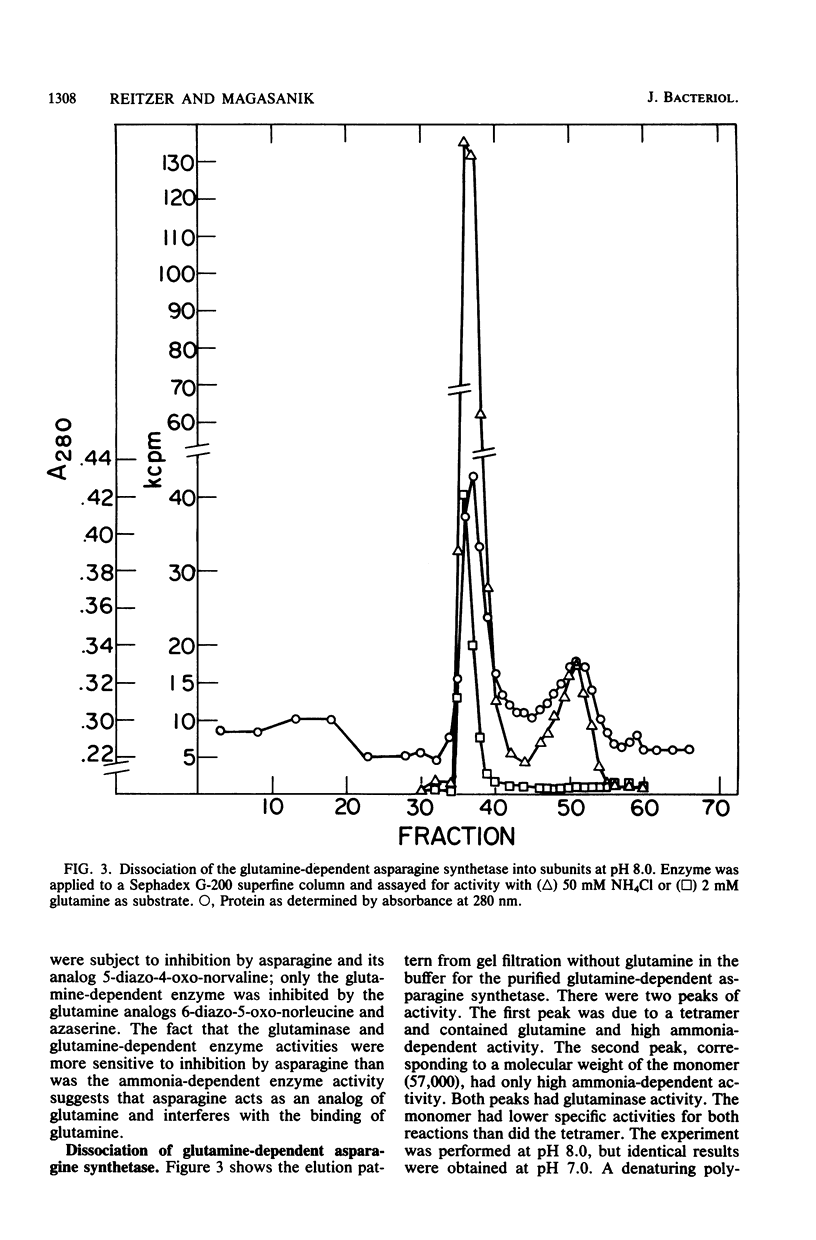
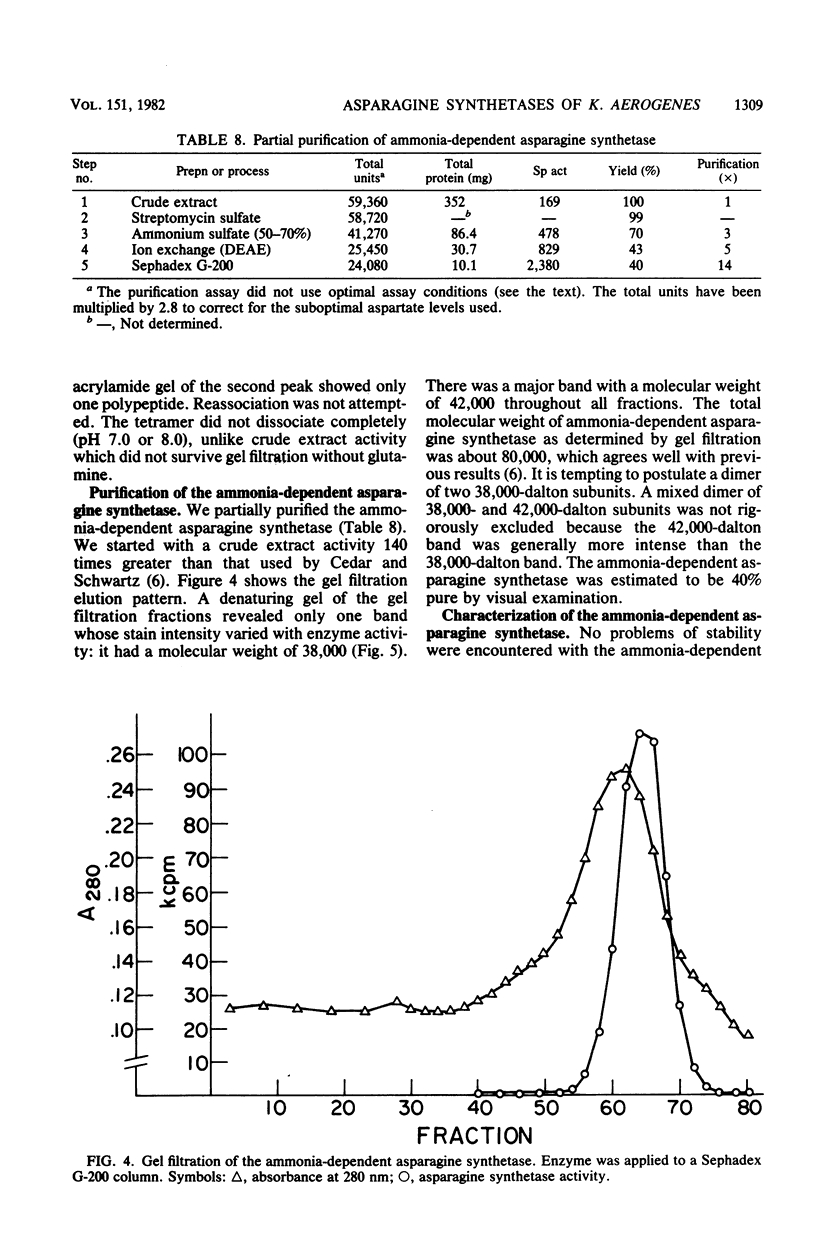
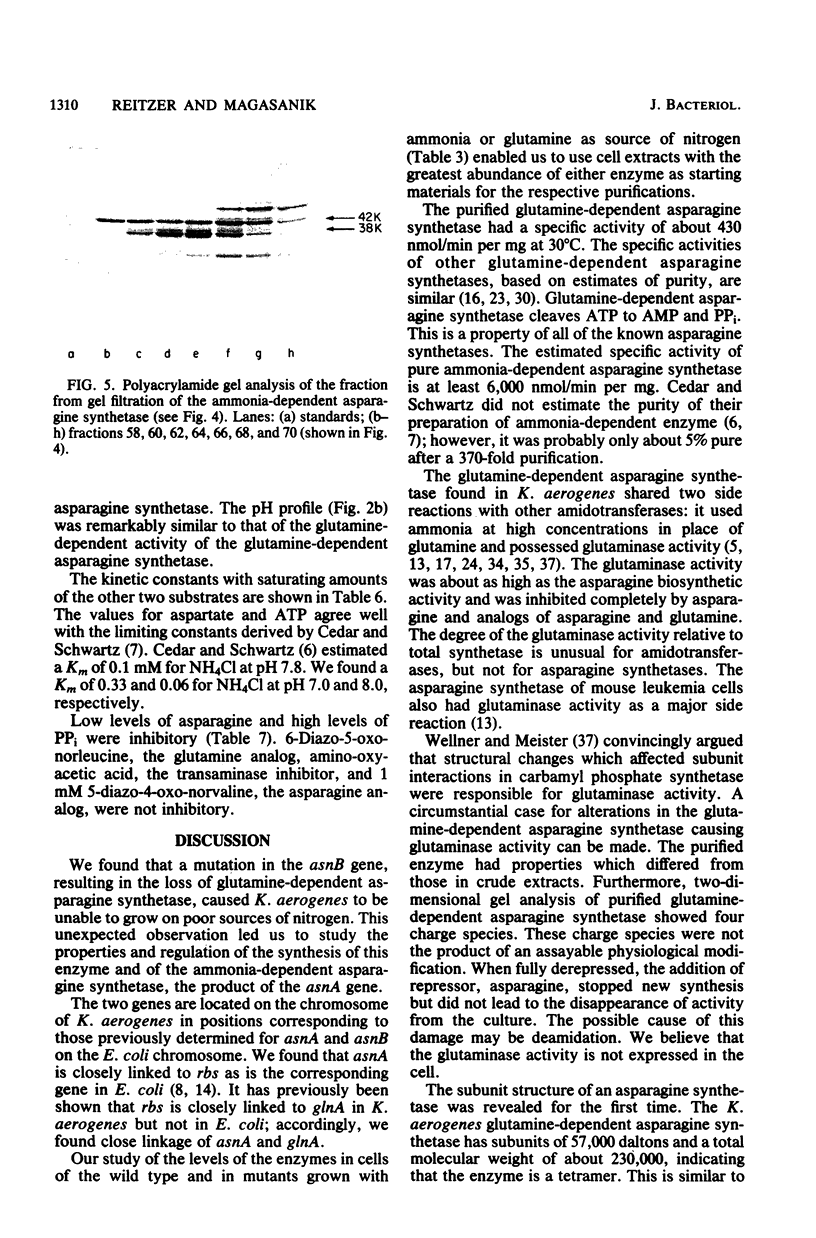
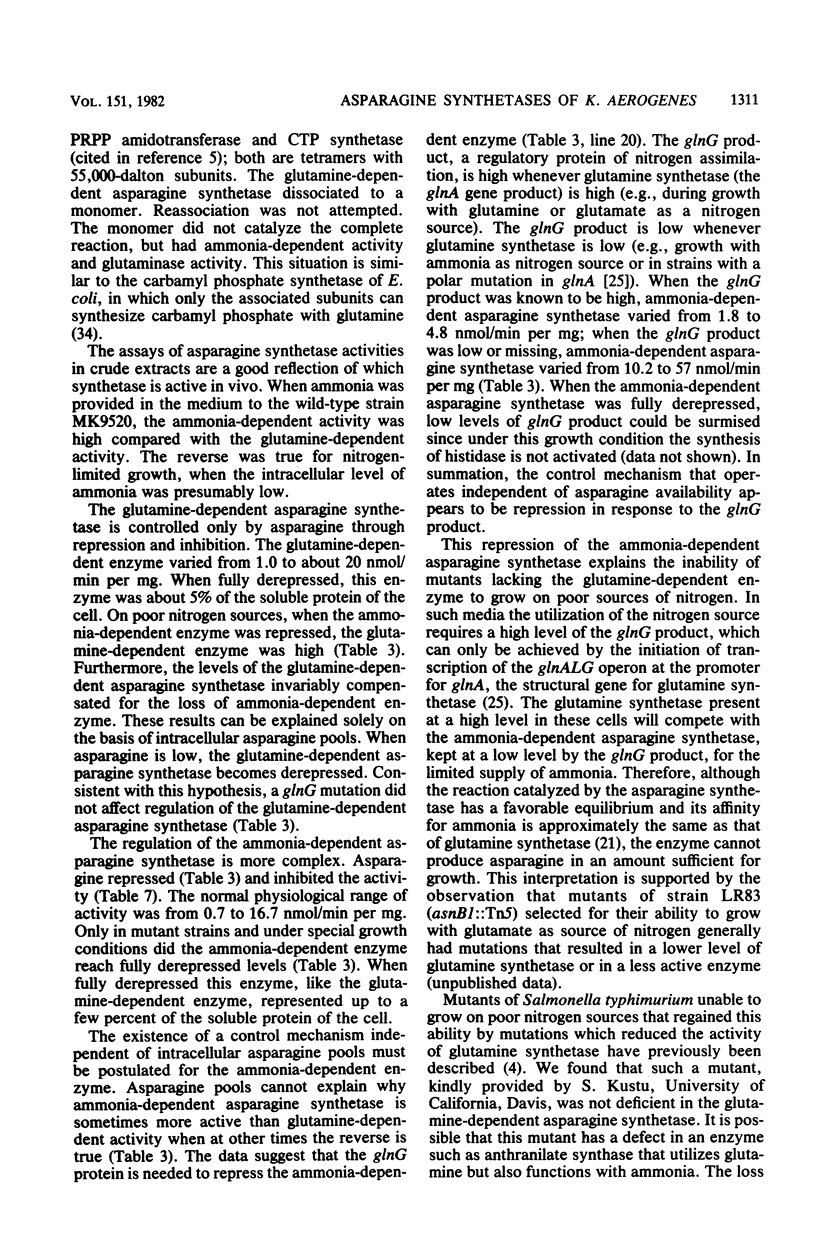
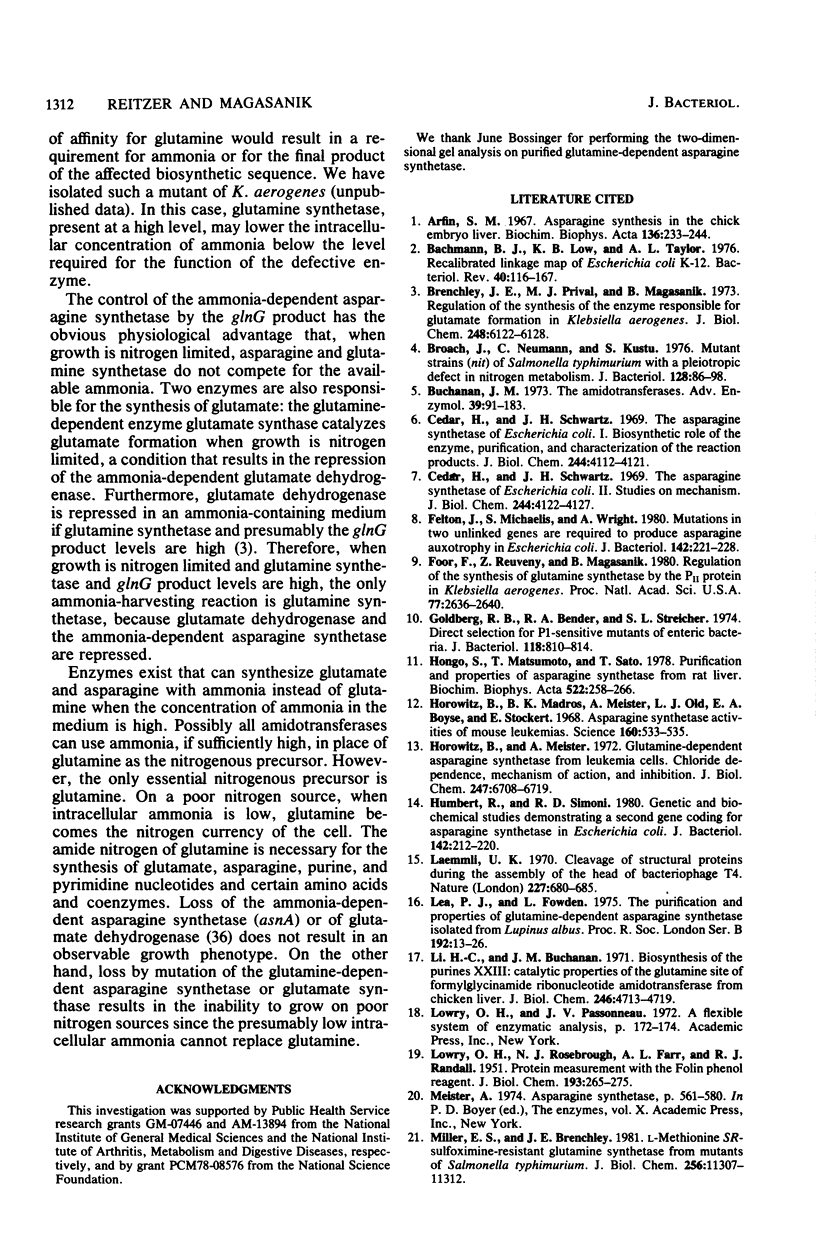
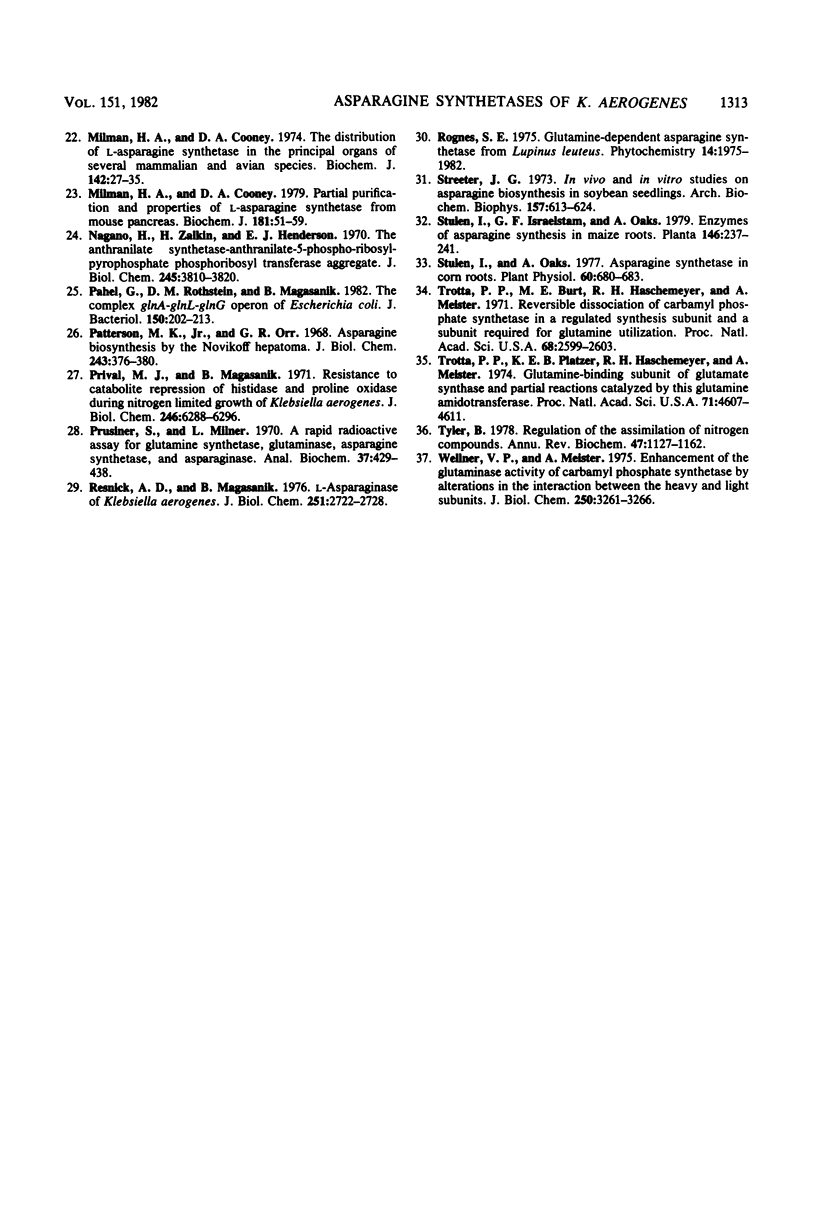
Images in this article
Selected References
These references are in PubMed. This may not be the complete list of references from this article.
- Arfin S. M. Asparagine synthesis in the chick embryo liver. Biochim Biophys Acta. 1967 Mar 22;136(2):233–244. doi: 10.1016/0304-4165(67)90068-2. [DOI] [PubMed] [Google Scholar]
- Bachmann B. J., Low K. B., Taylor A. L. Recalibrated linkage map of Escherichia coli K-12. Bacteriol Rev. 1976 Mar;40(1):116–167. doi: 10.1128/br.40.1.116-167.1976. [DOI] [PMC free article] [PubMed] [Google Scholar]
- Brenchley J. E., Prival M. J., Magasanik B. Regulation of the synthesis of enzymes responsible for glutamate formation in Klebsiella aerogenes. J Biol Chem. 1973 Sep 10;248(17):6122–6128. [PubMed] [Google Scholar]
- Broach J., Neumann C., Kustu S. Mutant strains (nit) of Salmonella typhimurium with a pleiotropic defect in nitrogen metabolism. J Bacteriol. 1976 Oct;128(1):86–98. doi: 10.1128/jb.128.1.86-98.1976. [DOI] [PMC free article] [PubMed] [Google Scholar]
- Buchanan J. M. The amidotransferases. Adv Enzymol Relat Areas Mol Biol. 1973;39:91–183. doi: 10.1002/9780470122846.ch2. [DOI] [PubMed] [Google Scholar]
- Cedar H., Schwartz J. H. The asparagine synthetase of Escherhic coli. I. Biosynthetic role of the enzyme, purification, and characterization of the reaction products. J Biol Chem. 1969 Aug 10;244(15):4112–4121. [PubMed] [Google Scholar]
- Cedar H., Schwartz J. H. The asparagine synthetase of Escherichia coli. II. Studies on mechanism. J Biol Chem. 1969 Aug 10;244(15):4122–4127. [PubMed] [Google Scholar]
- Felton J., Michaelis S., Wright A. Mutations in two unlinked genes are required to produce asparagine auxotrophy in Escherichia coli. J Bacteriol. 1980 Apr;142(1):221–228. doi: 10.1128/jb.142.1.221-228.1980. [DOI] [PMC free article] [PubMed] [Google Scholar]
- Foor F., Reuveny Z., Magasanik B. Regulation of the synthesis of glutamine synthetase by the PII protein in Klebsiella aerogenes. Proc Natl Acad Sci U S A. 1980 May;77(5):2636–2640. doi: 10.1073/pnas.77.5.2636. [DOI] [PMC free article] [PubMed] [Google Scholar]
- Goldberg R. B., Bender R. A., Streicher S. L. Direct selection for P1-sensitive mutants of enteric bacteria. J Bacteriol. 1974 Jun;118(3):810–814. doi: 10.1128/jb.118.3.810-814.1974. [DOI] [PMC free article] [PubMed] [Google Scholar]
- Hongo S., Matsumoto T., Sato T. Purification and properties of asparagine synthetase from rat liver. Biochim Biophys Acta. 1978 Jan 12;522(1):258–266. doi: 10.1016/0005-2744(78)90342-x. [DOI] [PubMed] [Google Scholar]
- Horowitz B., Madras B. K., Meister A., Old L. J., Boyes E. A., Stockert E. Asparagine synthetase activity of mouse leukemias. Science. 1968 May 3;160(3827):533–535. doi: 10.1126/science.160.3827.533. [DOI] [PubMed] [Google Scholar]
- Horowitz B., Meister A. Glutamine-dependent asparagine synthetase from leukemia cells. Chloride dependence, mechanism of action, and inhibition. J Biol Chem. 1972 Oct 25;247(20):6708–6719. [PubMed] [Google Scholar]
- Humbert R., Simoni R. D. Genetic and biomedical studies demonstrating a second gene coding for asparagine synthetase in Escherichia coli. J Bacteriol. 1980 Apr;142(1):212–220. doi: 10.1128/jb.142.1.212-220.1980. [DOI] [PMC free article] [PubMed] [Google Scholar]
- LOWRY O. H., ROSEBROUGH N. J., FARR A. L., RANDALL R. J. Protein measurement with the Folin phenol reagent. J Biol Chem. 1951 Nov;193(1):265–275. [PubMed] [Google Scholar]
- Laemmli U. K. Cleavage of structural proteins during the assembly of the head of bacteriophage T4. Nature. 1970 Aug 15;227(5259):680–685. doi: 10.1038/227680a0. [DOI] [PubMed] [Google Scholar]
- Li H. C., Buchanan J. M. Biosynthesis of the purines. 33. Catalytic properties of the glutamine site of formylglycinamide ribonucleotide amidotransferase from chicken liver. J Biol Chem. 1971 Aug 10;246(15):4713–4719. [PubMed] [Google Scholar]
- Miller E. S., Brenchley J. E. L-Methionine SR-sulfoximine-resistant glutamine synthetase from mutants of Salmonella typhimurium. J Biol Chem. 1981 Nov 10;256(21):11307–11312. [PubMed] [Google Scholar]
- Milman H. A., Cooney D. A. Partial purification and properties of L-asparagine synthetase from mouse pancreas. Biochem J. 1979 Jul 1;181(1):51–59. doi: 10.1042/bj1810051. [DOI] [PMC free article] [PubMed] [Google Scholar]
- Milman H. A., Cooney D. A. The distribution of L-asparagine synthetase in the principal organs of several mammalian and avian species. Biochem J. 1974 Jul;142(1):27–35. doi: 10.1042/bj1420027. [DOI] [PMC free article] [PubMed] [Google Scholar]
- Nagano H., Zalkin H., Henderson E. J. The anthranilate synthetase-anthranilate-5-phosphorribosylpyrophosphate phosphoribosyltransferase aggregate. On the reaction mechanism of anthranilate synthetase from Salmonella typhimurium. J Biol Chem. 1970 Aug 10;245(15):3810–3820. [PubMed] [Google Scholar]
- Pahel G., Rothstein D. M., Magasanik B. Complex glnA-glnL-glnG operon of Escherichia coli. J Bacteriol. 1982 Apr;150(1):202–213. doi: 10.1128/jb.150.1.202-213.1982. [DOI] [PMC free article] [PubMed] [Google Scholar]
- Patterson M. K., Jr, Orr G. R. Asparagine biosynthesis by the Novikoff Hepatoma isolation, purification, property, and mechanism studies of the enzyme system. J Biol Chem. 1968 Jan 25;243(2):376–380. [PubMed] [Google Scholar]
- Prival M. J., Magasanik B. Resistance to catabolite repression of histidase and proline oxidase during nitrogen-limited growth of Klebsiella aerogenes. J Biol Chem. 1971 Oct 25;246(20):6288–6296. [PubMed] [Google Scholar]
- Prusiner S., Milner L. A rapid radioactive assay for glutamine synthetase, glutaminase, asparagine synthetase, and asparaginase. Anal Biochem. 1970 Oct;37(2):429–438. doi: 10.1016/0003-2697(70)90069-2. [DOI] [PubMed] [Google Scholar]
- Resnick A. D., Magasanik B. L-Asparaginase of Klebsiella aerogenes. Activation of its synthesis by glutamine synthetase. J Biol Chem. 1976 May 10;251(9):2722–2728. [PubMed] [Google Scholar]
- Streeter J. G. In vivo and in vitro studies on asparagine biosynthesis in soybean seedlings. Arch Biochem Biophys. 1973 Aug;157(2):613–624. doi: 10.1016/0003-9861(73)90681-4. [DOI] [PubMed] [Google Scholar]
- Stulen I., Oaks A. Asparagine synthetase in corn roots. Plant Physiol. 1977 Nov;60(5):680–683. doi: 10.1104/pp.60.5.680. [DOI] [PMC free article] [PubMed] [Google Scholar]
- Trotta P. P., Burt M. E., Haschemeyer R. H., Meister A. Reversible dissociation of carbamyl phosphate synthetase into a regulated synthesis subunit and a subunit required for glutamine utilization. Proc Natl Acad Sci U S A. 1971 Oct;68(10):2599–2603. doi: 10.1073/pnas.68.10.2599. [DOI] [PMC free article] [PubMed] [Google Scholar]
- Trotta P. P., Platzer K. E., Haschemeyer R. H., Meister A. Glutamine-binding subunit of glutamate synthase and partial reactions catalyzed by this glutamine amidotransferase. Proc Natl Acad Sci U S A. 1974 Nov;71(11):4607–4611. doi: 10.1073/pnas.71.11.4607. [DOI] [PMC free article] [PubMed] [Google Scholar]
- Tyler B. Regulation of the assimilation of nitrogen compounds. Annu Rev Biochem. 1978;47:1127–1162. doi: 10.1146/annurev.bi.47.070178.005403. [DOI] [PubMed] [Google Scholar]
- Wellner V. P., Meister A. Enhancement of the glutaminase activity of carbamyl phosphate synthetase by alterations in the interaction between the heavy and light subunits. J Biol Chem. 1975 May 10;250(9):3261–3266. [PubMed] [Google Scholar]