Abstract
The fermentation of fumarate and L-malate by Clostridium formicoaceticum was investigated. Growing and nongrowing cells degraded fumarate by dismutation to succinate, acetate, and CO2; on the other hand, only small amounts of succinate were detected when the organism was grown on L-malate. This dicarboxylic acid was mainly converted to acetate and CO2. The fermentation balances were modified if bicarbonate or formate were present in the medium. When C. formicoaceticum was grown in the presence of both dicarboxylic acids, fumarate was consumed before L-malate. The latter was mainly converted to acetate, whereas fumarate was fermented to acetate and succinate. Molar growth yields were determined to be 6 g of dry weight per mol of fumarate and 8 g of dry weight per mol of L-malate fermented.
Full text
PDF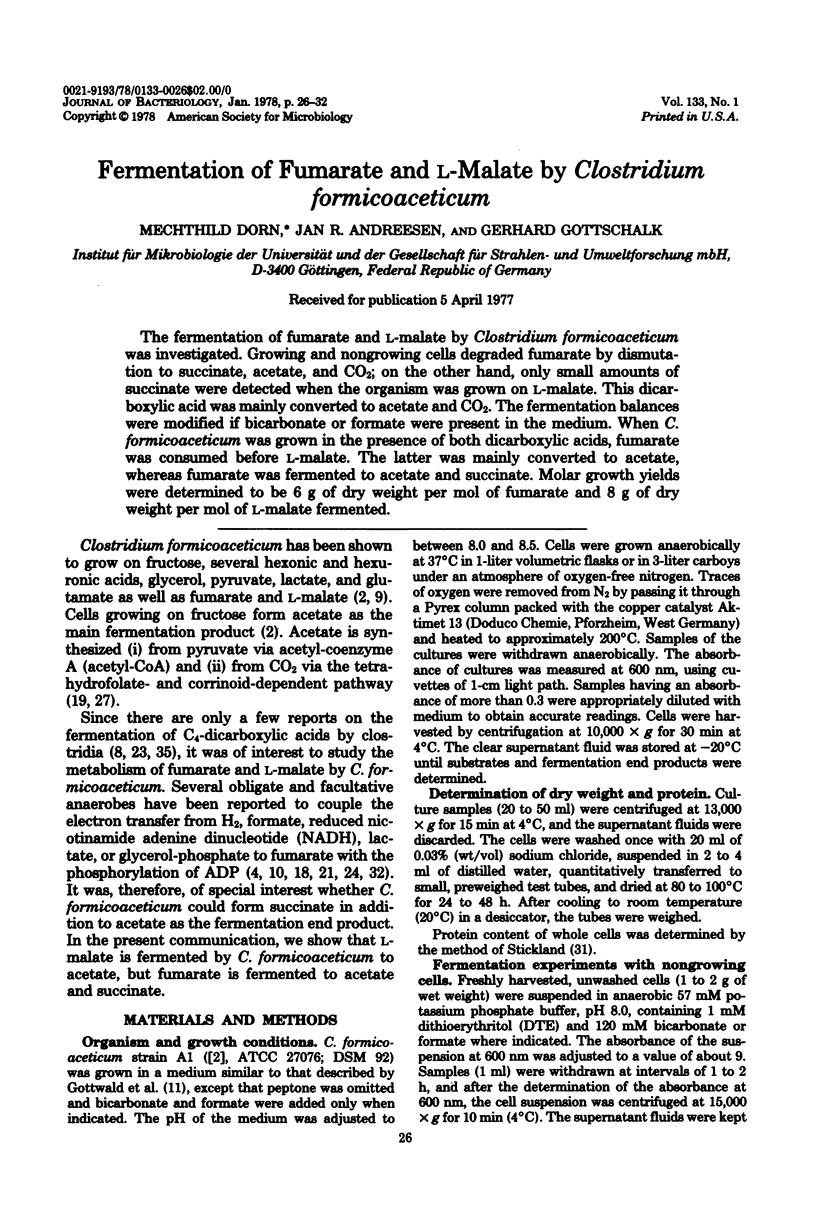
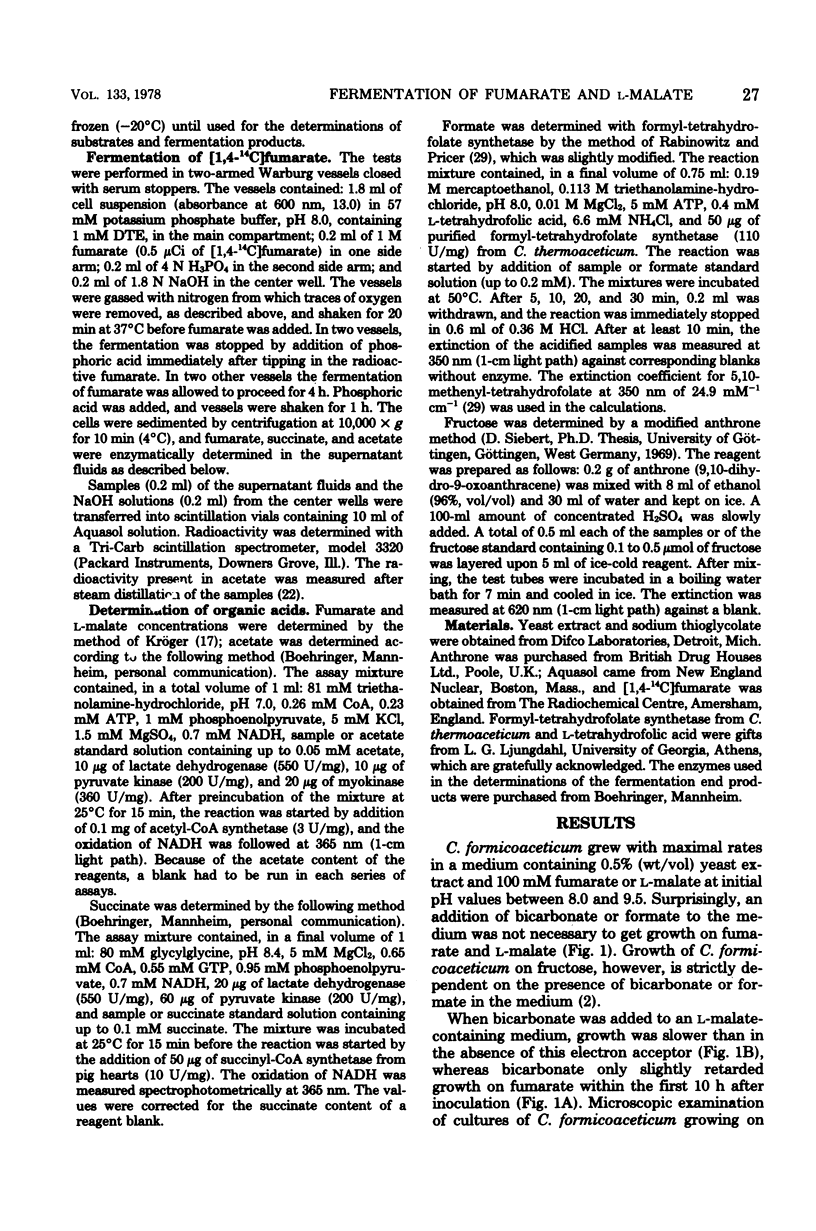
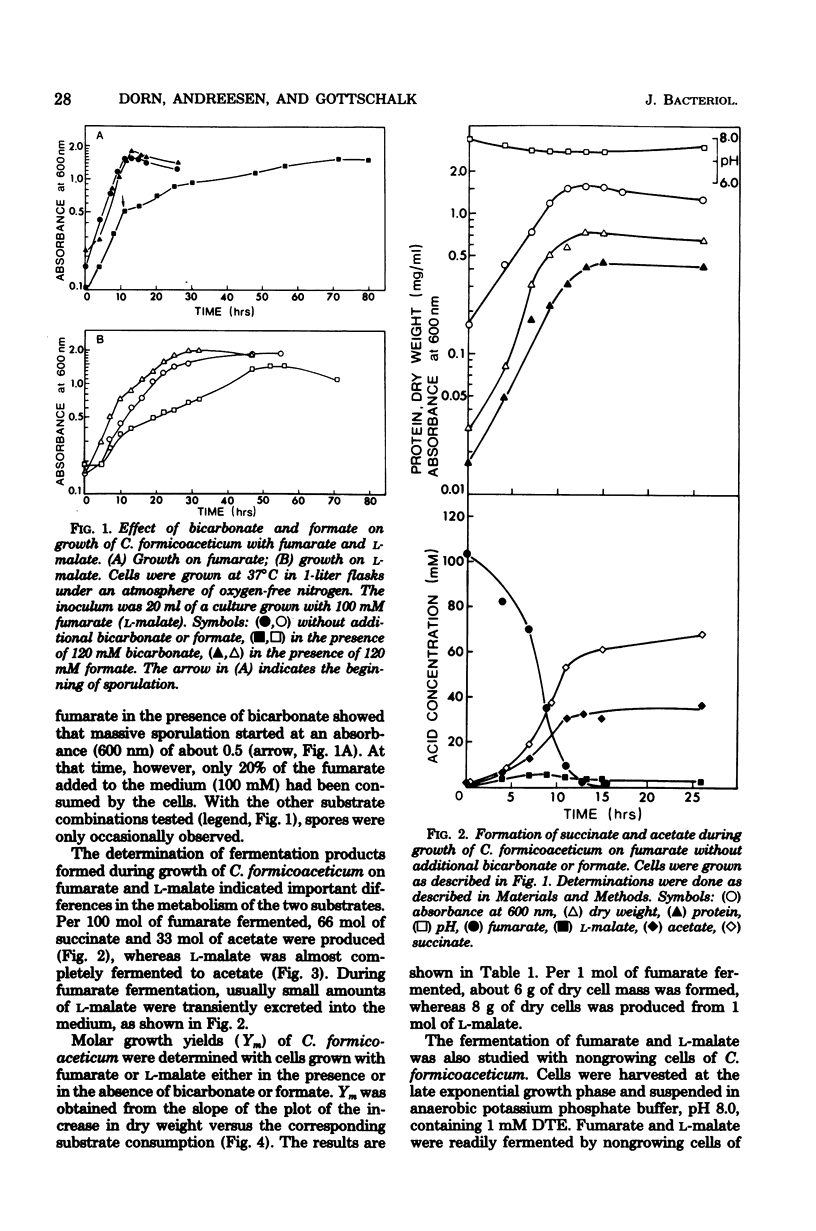
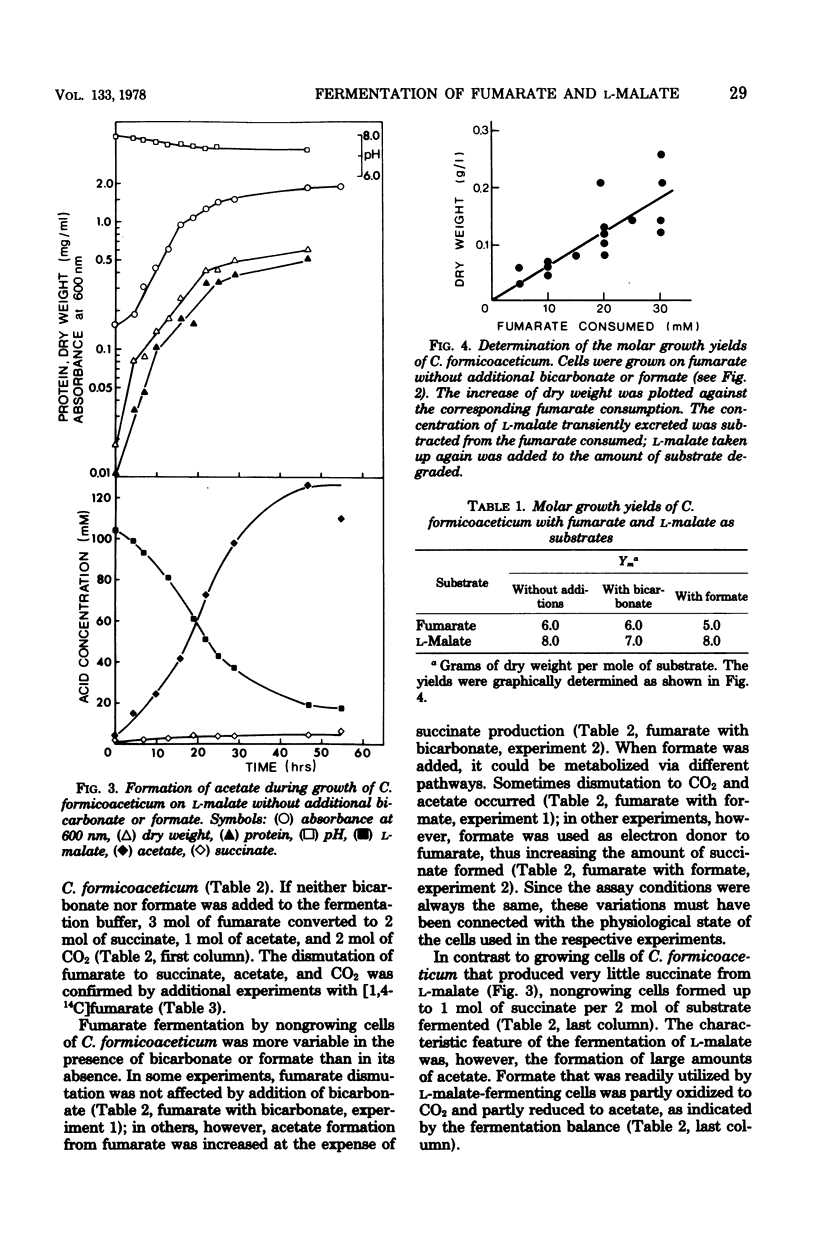
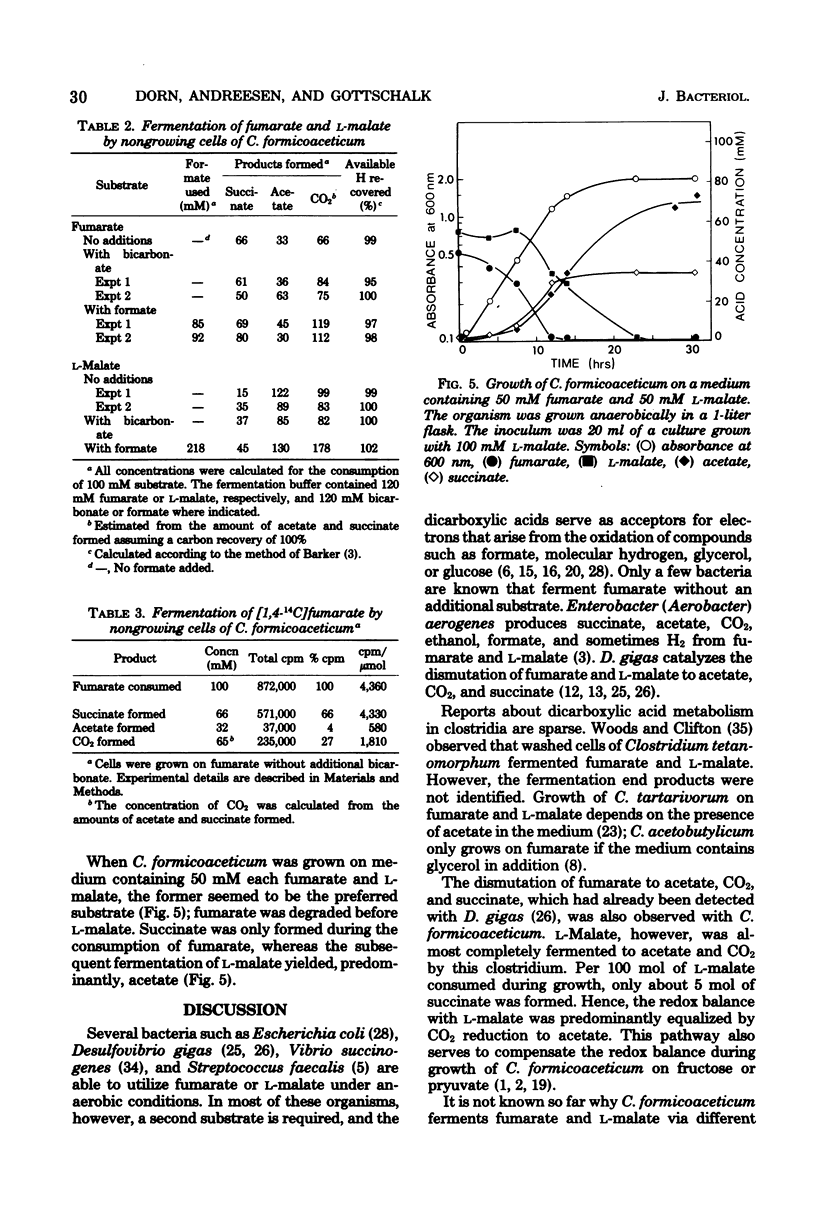
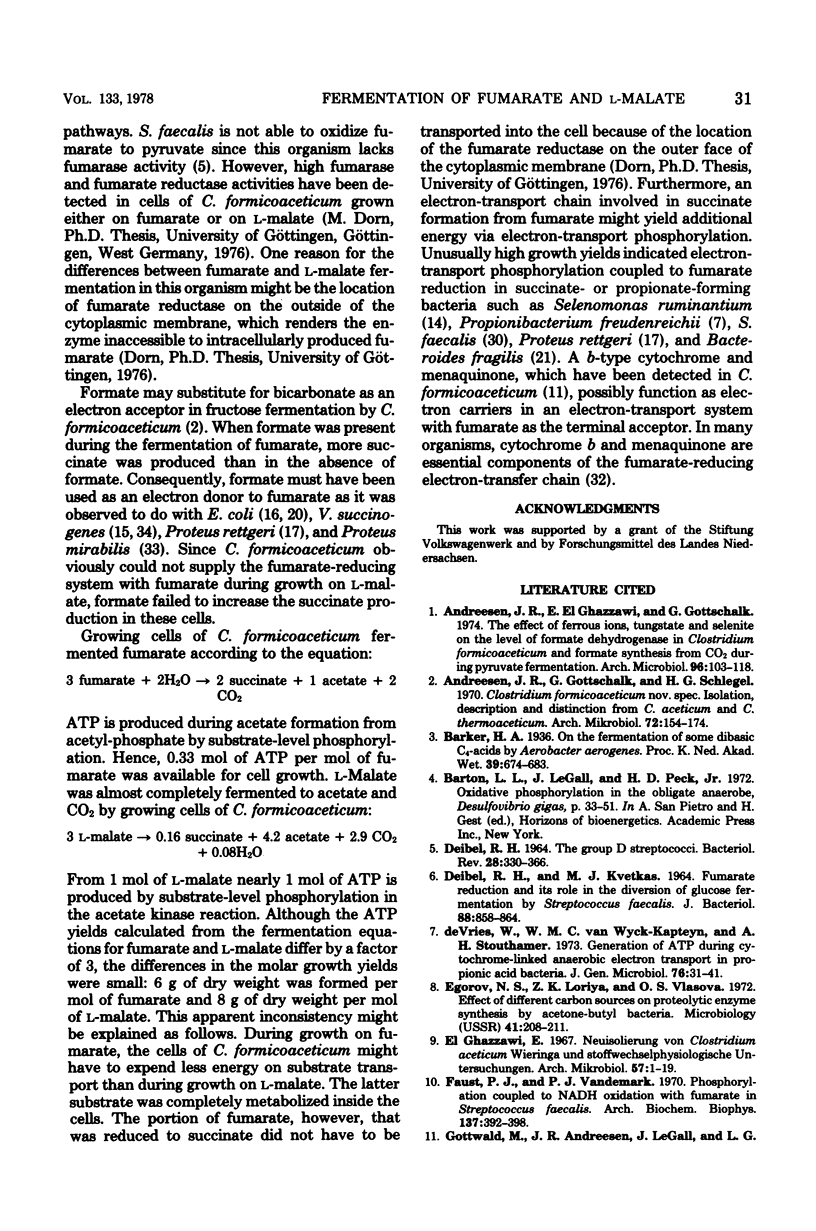
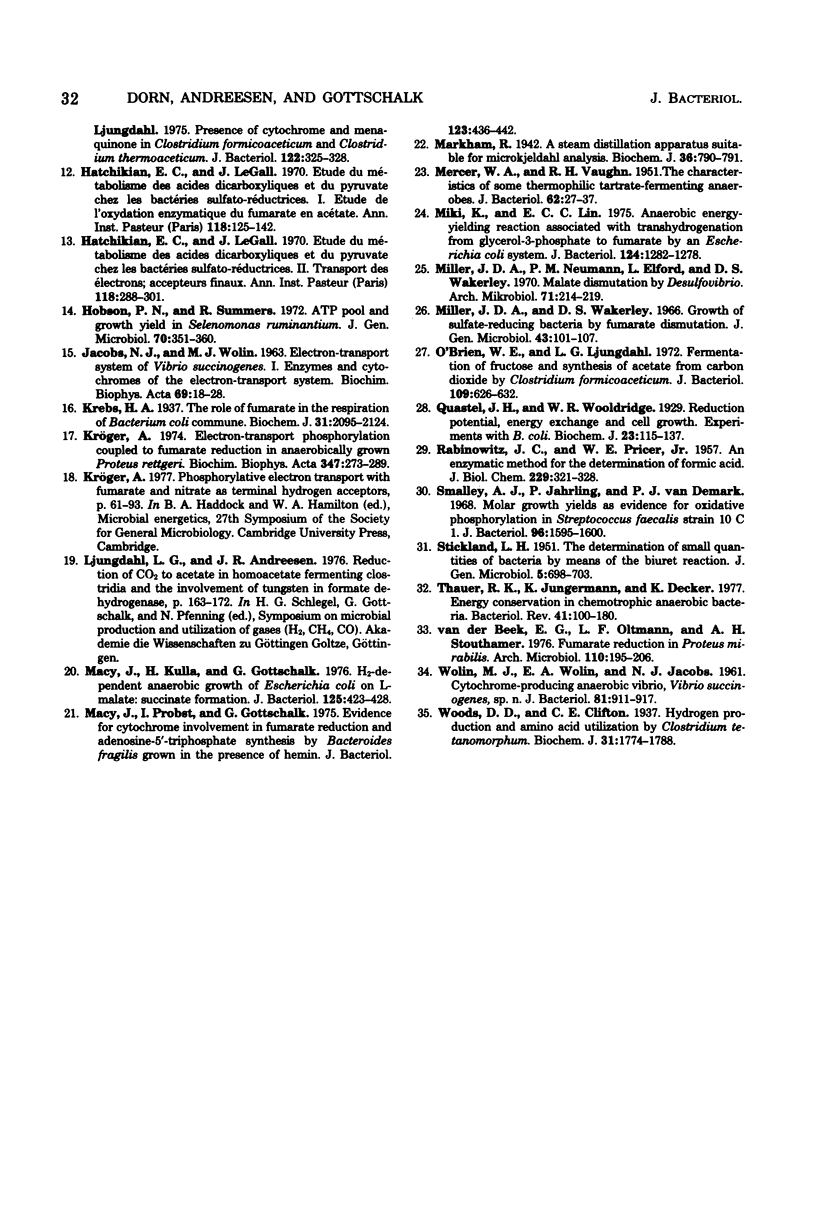
Selected References
These references are in PubMed. This may not be the complete list of references from this article.
- Andreesen J. R., El Ghazzawi E., Gottschalk G. The effect of ferrous ions, tungstate and selenite on the level of formate dehydrogenase in Clostridium formicoaceticum and formate synthesis from CO2 during pyruvate fermentation. Arch Mikrobiol. 1974 Mar 4;96(2):103–118. doi: 10.1007/BF00590167. [DOI] [PubMed] [Google Scholar]
- Andreesen J. R., Gottschalk G., Schlegel H. G. Clostridium formicoaceticum nov. spec. isolation, description and distinction from C. aceticum and C. thermoaceticum. Arch Mikrobiol. 1970;72(2):154–174. doi: 10.1007/BF00409521. [DOI] [PubMed] [Google Scholar]
- DEIBEL R. H., KVETKAS M. J. FUMARATE REDUCTION AND ITS ROLE IN THE DIVERSION OF GLUCOSE FERMENTATION BY STREPTOCOCCUS FAECALIS. J Bacteriol. 1964 Oct;88:858–864. doi: 10.1128/jb.88.4.858-864.1964. [DOI] [PMC free article] [PubMed] [Google Scholar]
- DEIBEL R. H. THE GROUP D STREPTOCOCCI. Bacteriol Rev. 1964 Sep;28:330–366. doi: 10.1128/br.28.3.330-366.1964. [DOI] [PMC free article] [PubMed] [Google Scholar]
- Faust P. J., Vandemark P. J. Phosphorylation coupled to NADH oxidation with fumarate in Streptococcus faecalis 10Cl. Arch Biochem Biophys. 1970 Apr;137(2):392–398. doi: 10.1016/0003-9861(70)90454-6. [DOI] [PubMed] [Google Scholar]
- Gottwald M., Andreesen J. R., LeGall J., Ljungdahl L. G. Presence of cytochrome and menaquinone in Clostridium formicoaceticum and Clostridium thermoaceticum. J Bacteriol. 1975 Apr;122(1):325–328. doi: 10.1128/jb.122.1.325-328.1975. [DOI] [PMC free article] [PubMed] [Google Scholar]
- Hatchikian E. C., Le Gall J. Etude du métabolisme des acides dicarboxyliques et du pyruvate chez les bactéries sulfato-réductrices. I. Etude de l'oxydation enzymatique du fumarate en acétate. Ann Inst Pasteur (Paris) 1970 Feb;118(2):125–142. [PubMed] [Google Scholar]
- Hatchikian E. C., Le Gall J. Etude du métabolisme des acides dicarboxyliques et du pyruvate chez les bactéries sulfato-réductrices. II. Transport des électrons; accepteurs finaux. Ann Inst Pasteur (Paris) 1970 Mar;118(3):288–301. [PubMed] [Google Scholar]
- JACOBS N. J., WOLIN M. J. Electron-transport system of Vibrio succinogenes. I. Enzymes and cytochromes of electron-transport system. Biochim Biophys Acta. 1963 Jan 1;69:18–28. doi: 10.1016/0006-3002(63)91221-6. [DOI] [PubMed] [Google Scholar]
- Krebs H. A. The role of fumarate in the respiration of Bacterium coli commune. Biochem J. 1937 Nov;31(11):2095–2124. doi: 10.1042/bj0312095. [DOI] [PMC free article] [PubMed] [Google Scholar]
- MERCER W. A., VAUGHN R. H. The characteristics of some thermophilic, tartrate-fermenting anaerobes. J Bacteriol. 1951 Jul;62(1):27–37. doi: 10.1128/jb.62.1.27-37.1951. [DOI] [PMC free article] [PubMed] [Google Scholar]
- Macy J., Kulla H., Gottschalk G. H2-dependent anaerobic growth of Escherichia coli on L-malate: succinate formation. J Bacteriol. 1976 Feb;125(2):423–428. doi: 10.1128/jb.125.2.423-428.1976. [DOI] [PMC free article] [PubMed] [Google Scholar]
- Macy J., Probst I., Gottschalk G. Evidence for cytochrome involvement in fumarate reduction and adenosine 5'-triphosphate synthesis by Bacteroides fragilis grown in the presence of hemin. J Bacteriol. 1975 Aug;123(2):436–442. doi: 10.1128/jb.123.2.436-442.1975. [DOI] [PMC free article] [PubMed] [Google Scholar]
- Markham R. A steam distillation apparatus suitable for micro-Kjeldahl analysis. Biochem J. 1942 Dec;36(10-12):790–791. doi: 10.1042/bj0360790. [DOI] [PMC free article] [PubMed] [Google Scholar]
- Miki K., Lin E. C. Anaerobic energy-yielding reaction associated with transhydrogenation from glycerol 3-phosphate to fumarate by an Escherichia coli system. J Bacteriol. 1975 Dec;124(3):1282–1287. doi: 10.1128/jb.124.3.1282-1287.1975. [DOI] [PMC free article] [PubMed] [Google Scholar]
- Miller J. D., Neumann P. M., Elford L., Wakerley D. S. Malate dismutation by Desulfovibrio. Arch Mikrobiol. 1970;71(3):214–219. doi: 10.1007/BF00410154. [DOI] [PubMed] [Google Scholar]
- Miller J. D., Wakerley D. S. Growth of sulphate-reducing bacteria by fumarate dismutation. J Gen Microbiol. 1966 Apr;43(1):101–107. doi: 10.1099/00221287-43-1-101. [DOI] [PubMed] [Google Scholar]
- O'Brien W. E., Ljungdahl L. G. Fermentation of fructose and synthesis of acetate from carbon dioxide by Clostridium formicoaceticum. J Bacteriol. 1972 Feb;109(2):626–632. doi: 10.1128/jb.109.2.626-632.1972. [DOI] [PMC free article] [PubMed] [Google Scholar]
- Quastel J. H., Wooldridge W. R. Reduction potential, energy exchange and cell growth: Experiments with B. coli. Biochem J. 1929;23(1):115–137. doi: 10.1042/bj0230115. [DOI] [PMC free article] [PubMed] [Google Scholar]
- RABINOWITZ J. C., PRICER W. E., Jr An enzymatic method for the determination of formic acid. J Biol Chem. 1957 Nov;229(1):321–328. [PubMed] [Google Scholar]
- STICKLAND L. H. The determination of small quantities of bacteria by means of the biuret reaction. J Gen Microbiol. 1951 Oct;5(4):698–703. doi: 10.1099/00221287-5-4-698. [DOI] [PubMed] [Google Scholar]
- Smalley A. J., Jahrling P., Van Demark P. J. Molar growth yields as evidence for oxidative phosphorylation in Streptococcus faecalis strain 10Cl. J Bacteriol. 1968 Nov;96(5):1595–1600. doi: 10.1128/jb.96.5.1595-1600.1968. [DOI] [PMC free article] [PubMed] [Google Scholar]
- Thauer R. K., Jungermann K., Decker K. Energy conservation in chemotrophic anaerobic bacteria. Bacteriol Rev. 1977 Mar;41(1):100–180. doi: 10.1128/br.41.1.100-180.1977. [DOI] [PMC free article] [PubMed] [Google Scholar]
- Van der Beek E. G., Oltmann L. F., Stouthamer A. H. Fumarate reduction in Proteus mirabilis. Arch Microbiol. 1976 Nov 2;110(23):195–206. doi: 10.1007/BF00690228. [DOI] [PubMed] [Google Scholar]
- WOLIN M. J., WOLIN E. A., JACOBS N. J. Cytochrome-producing anaerobic Vibrio succinogenes, sp. n. J Bacteriol. 1961 Jun;81:911–917. doi: 10.1128/jb.81.6.911-917.1961. [DOI] [PMC free article] [PubMed] [Google Scholar]
- Woods D. D., Clifton C. E. Studies in the metabolism of the strict anaerobes (genus Clostridium): Hydrogen production and amino-acid utilization by Clostridium tetanomorphum. Biochem J. 1937 Oct;31(10):1774–1788. doi: 10.1042/bj0311774. [DOI] [PMC free article] [PubMed] [Google Scholar]
- de Vries W., van Wyck-Kapteyn W. M., Stouthamer A. H. Generation of ATP during cytochrome-linked anaerobic electron transport in propionic acid bacteria. J Gen Microbiol. 1973 May;76(1):31–41. doi: 10.1099/00221287-76-1-31. [DOI] [PubMed] [Google Scholar]