Abstract
The transduction of energy through biological membranes was investigated in Escherichia coli strains defective in the ATP synthetase complex. Everted vesicles prepared from strains containing an uncA or uncB mutation were compared with those of the parental strain for their ability to couple energy derived from the oxidation of substrates by the electron transport chain or from the hydrolysis of ATP by the Mg2+-adenosine triphosphatase, as measured by the energy-dependent quenching of quinacrine fluorescence or the active transport of 45Ca2+. Removal of the Mg2+-adenosine triphosphatase from membranes derived from the parental or an uncA strain caused a loss of energy-linked functions and a concomitant increase in the permeability of the membrane for protons. Proton impermeability was restored by treatment with N,N'-dicyclohexylcarbodiimide. When membranes of the uncB strain were treated in a similar manner, there was no loss of respiratory-driven functions, nor was there a change in proton permeability. These observations suggest that the uncB mutation specifically results in alteration of an intrinsic membrane protein channel necessary for the generation of utilzation of the electrochemical gradient of protons by that complex. Loss of the function of the proton channel is believed to prevent the transduction of energy through the ATP synthetase complex.
Full text
PDF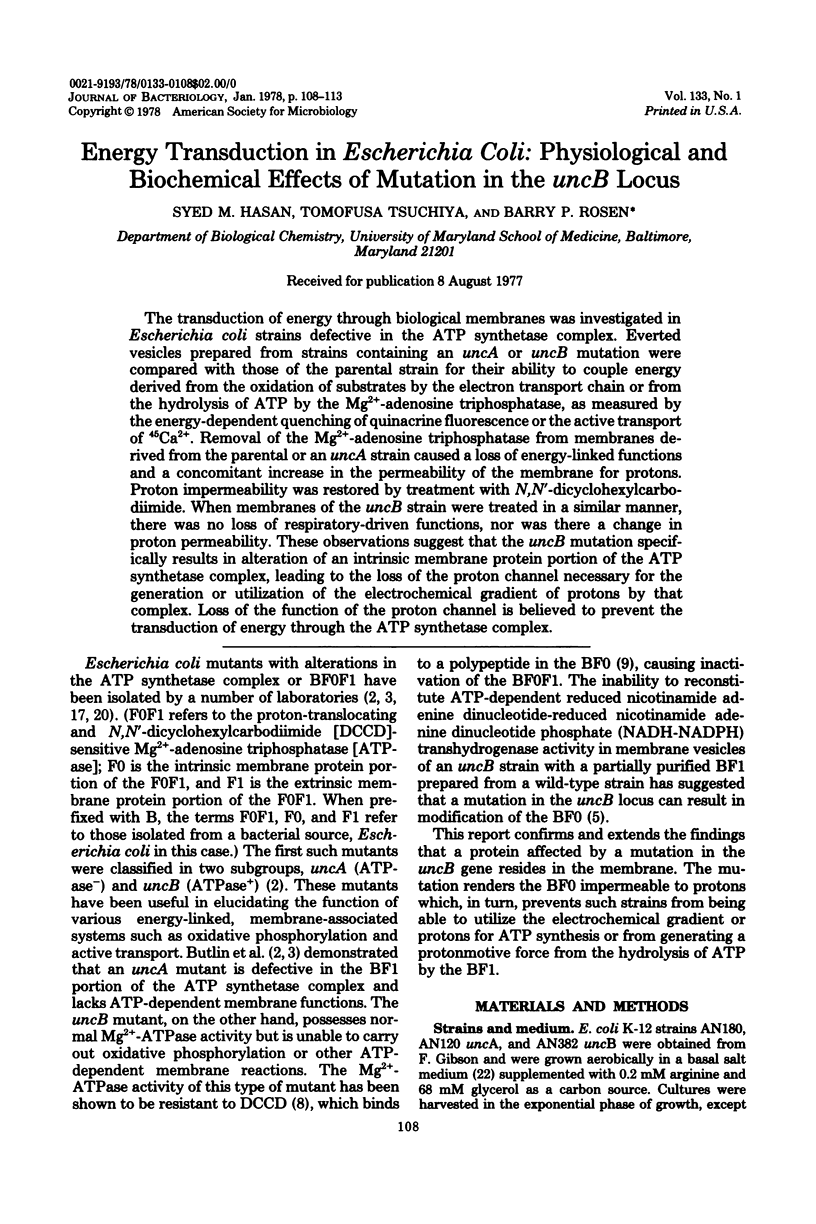
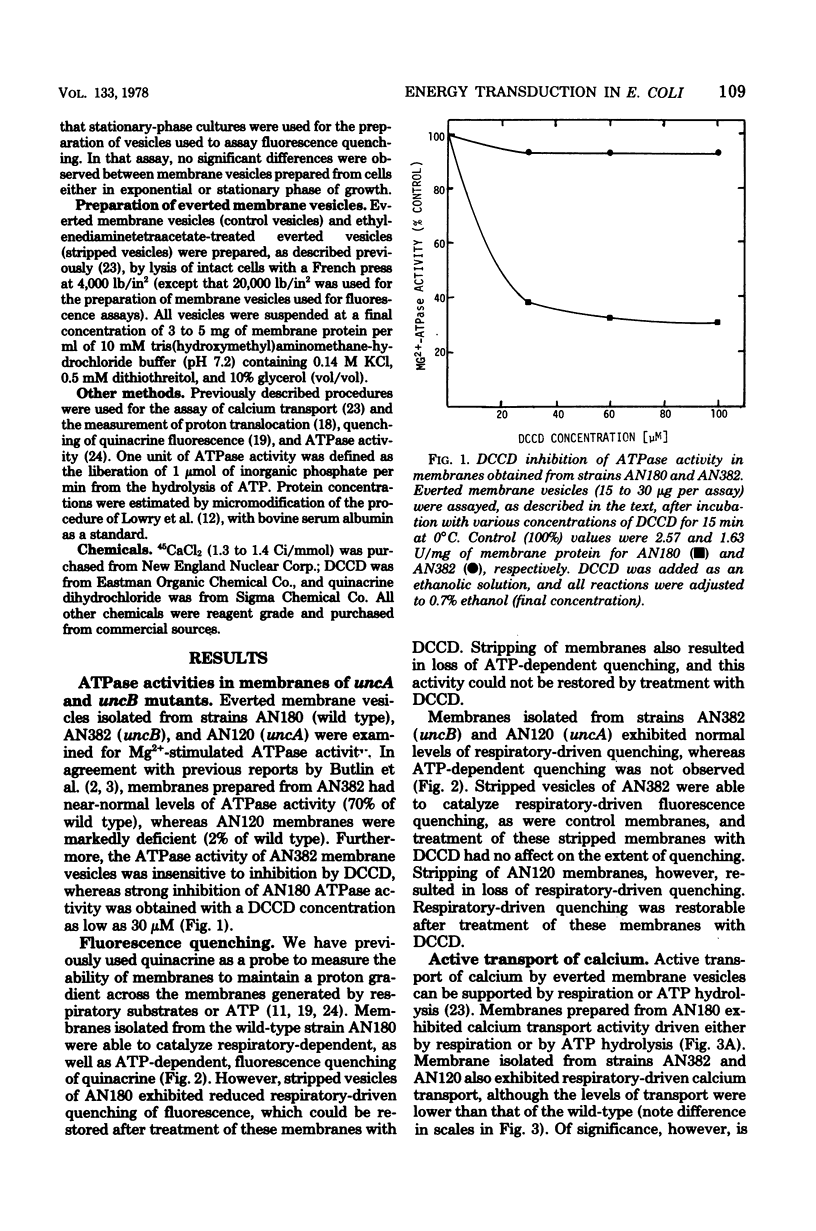
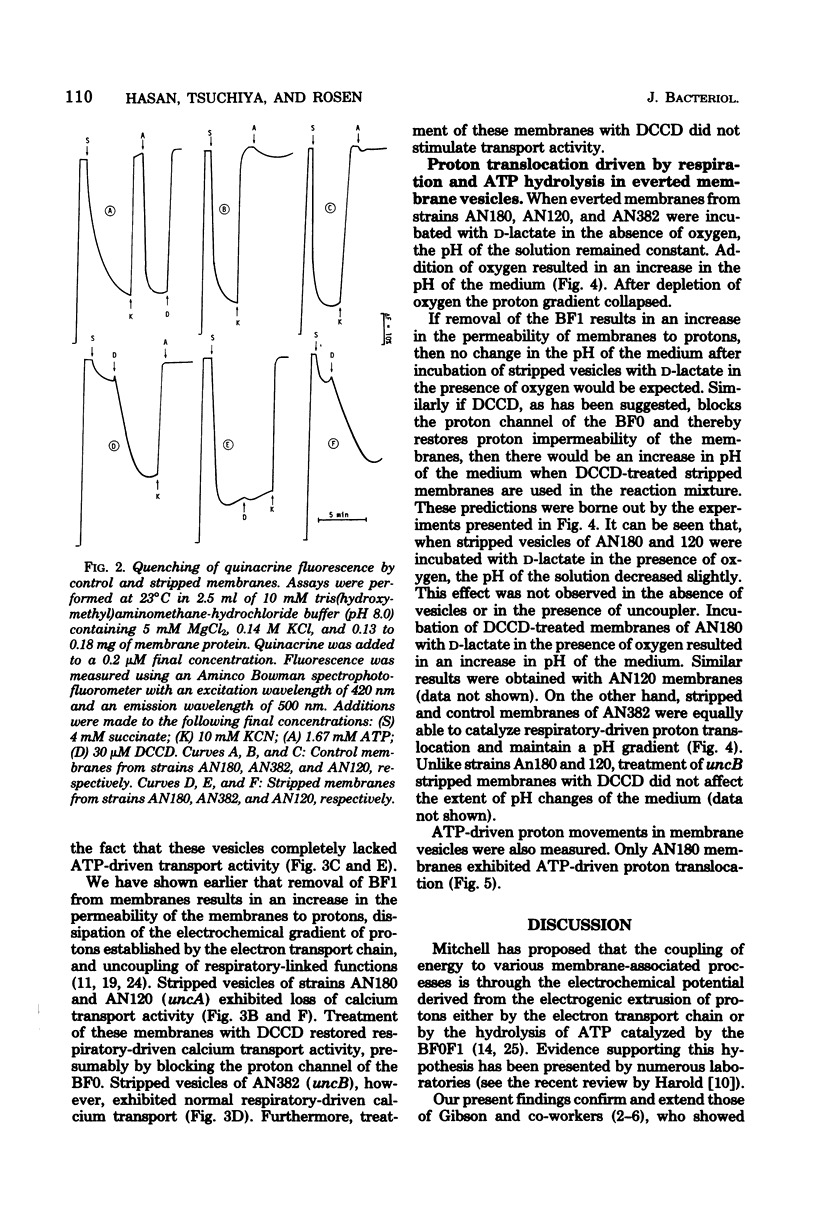
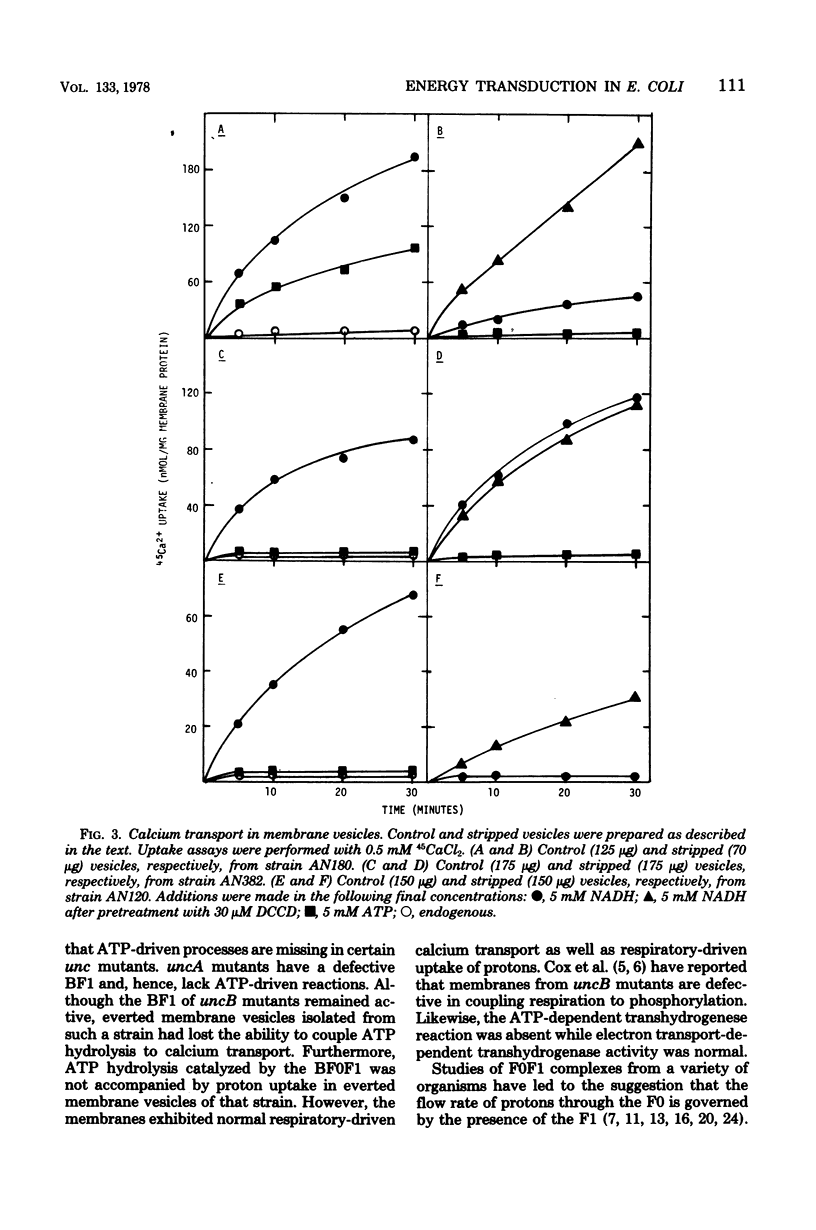
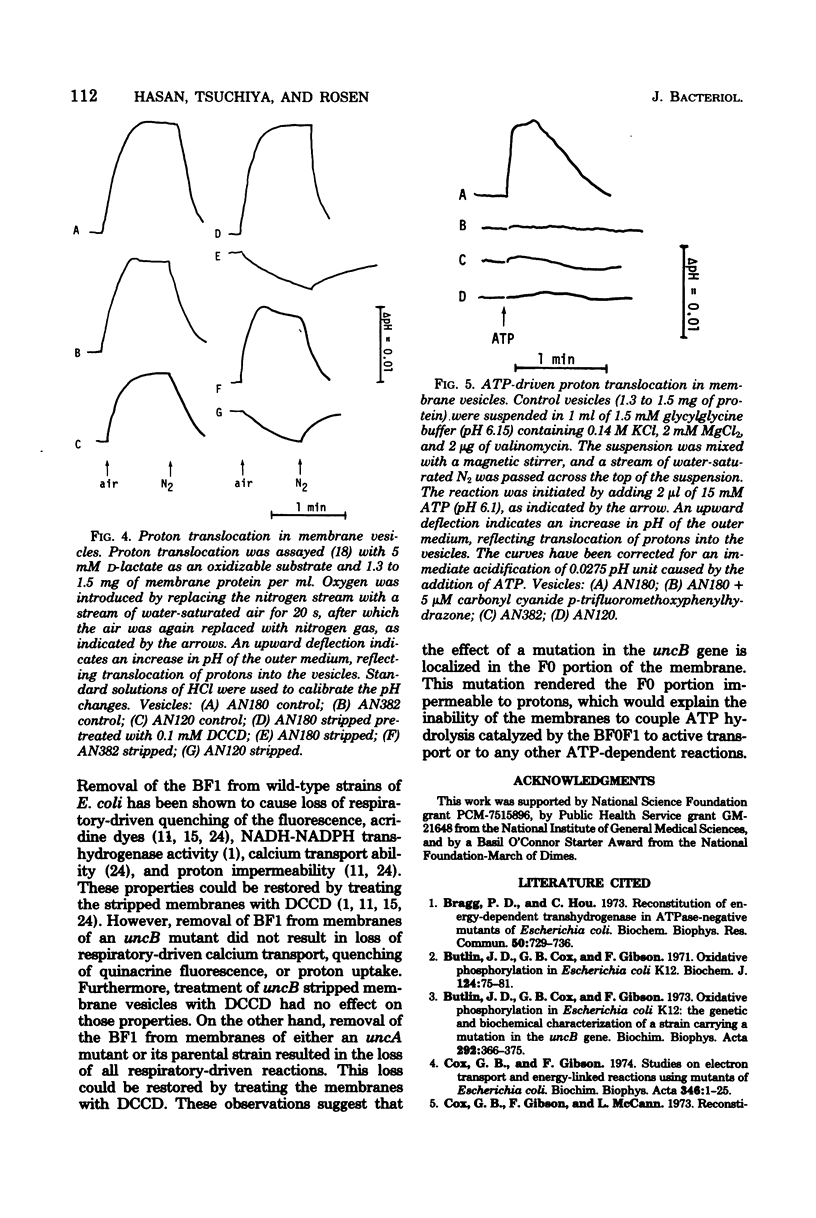
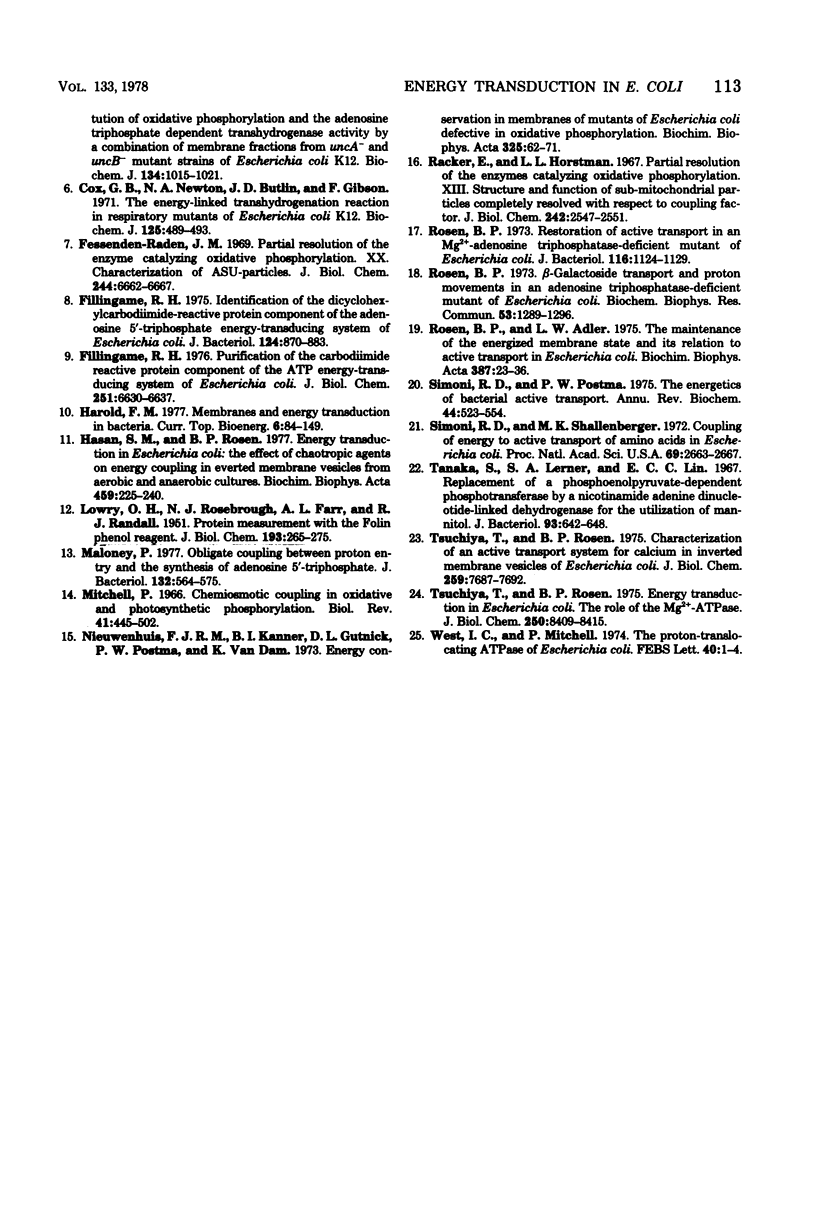
Selected References
These references are in PubMed. This may not be the complete list of references from this article.
- Bragg P. D., Hou C. Reconstitution of energy-dependent transhydrogenase in ATPase-negative mutants of Escherichia coli. Biochem Biophys Res Commun. 1973 Feb 5;50(3):729–736. doi: 10.1016/0006-291x(73)91305-3. [DOI] [PubMed] [Google Scholar]
- Butlin J. D., Cox G. B., Gibson F. Oxidative phosphorylation in Escherichia coli K-12: the genetic and biochemical characterisations of a strain carrying a mutation in the uncB gene. Biochim Biophys Acta. 1973 Feb 22;292(2):366–375. doi: 10.1016/0005-2728(73)90043-1. [DOI] [PubMed] [Google Scholar]
- Butlin J. D., Cox G. B., Gibson F. Oxidative phosphorylation in Escherichia coli K12. Mutations affecting magnesium ion- or calcium ion-stimulated adenosine triphosphatase. Biochem J. 1971 Aug;124(1):75–81. doi: 10.1042/bj1240075. [DOI] [PMC free article] [PubMed] [Google Scholar]
- Cox G. B., Gibson F. Studies on electron transport and energy-linked reactions using mutants of Escherichia coli. Biochim Biophys Acta. 1974 Apr 30;346(1):1–25. doi: 10.1016/0304-4173(74)90010-x. [DOI] [PubMed] [Google Scholar]
- Cox G. B., Newton N. A., Butlin J. D., Gibson F. The energy-linked transhydrogenase reaction in respiratory mutants of Escherichia coli K12. Biochem J. 1971 Nov;125(2):489–493. doi: 10.1042/bj1250489. [DOI] [PMC free article] [PubMed] [Google Scholar]
- Fessenden-Raden J. M. Partial resolution of the enzymes catalyzing oxidative phosphorylation. XX. Characterization of ASU-particles. J Biol Chem. 1969 Dec 25;244(24):6662–6667. [PubMed] [Google Scholar]
- Hasan S. M., Rosen B. P. Energy transduction in Escherichia coli. The effect of chaotropic agents on energy coupling in everted membrane vesicles from aerobic and anaerobic cultures. Biochim Biophys Acta. 1977 Feb 7;459(2):225–240. doi: 10.1016/0005-2728(77)90024-x. [DOI] [PubMed] [Google Scholar]
- LOWRY O. H., ROSEBROUGH N. J., FARR A. L., RANDALL R. J. Protein measurement with the Folin phenol reagent. J Biol Chem. 1951 Nov;193(1):265–275. [PubMed] [Google Scholar]
- Maloney P. C. Obligatory coupling between proton entry and the synthesis of adenosine 5'-triphosphate in Streptococcus lactis. J Bacteriol. 1977 Nov;132(2):564–575. doi: 10.1128/jb.132.2.564-575.1977. [DOI] [PMC free article] [PubMed] [Google Scholar]
- Mitchell P. Chemiosmotic coupling in oxidative and photosynthetic phosphorylation. Biol Rev Camb Philos Soc. 1966 Aug;41(3):445–502. doi: 10.1111/j.1469-185x.1966.tb01501.x. [DOI] [PubMed] [Google Scholar]
- Nieuwenhuis F. J., Kanner B. I., Gutnick D. L., Postma P. W., van Dam K. Energy conservation in membranes of mutants of Escherichia coli defective in oxidative phosphorylation. Biochim Biophys Acta. 1973 Oct 19;325(1):62–71. doi: 10.1016/0005-2728(73)90151-5. [DOI] [PubMed] [Google Scholar]
- Racker E., Horstman L. L. Partial resolution of the enzymes catalyzing oxidative phosphorylation. 13. Structure and function of submitochondrial particles completely resolved with respect to coupling factor. J Biol Chem. 1967 May 25;242(10):2547–2551. [PubMed] [Google Scholar]
- Rosen B. P., Adler L. W. The maintenance of the energized membrane state and its relation to active transport in Escherichia coli. Biochim Biophys Acta. 1975 Apr 14;387(1):23–36. doi: 10.1016/0005-2728(75)90049-3. [DOI] [PubMed] [Google Scholar]
- Rosen B. P. Beta-galactoside transport and proton movements in an adenosine triphosphatase-deficient mutant of Escherichia coli. Biochem Biophys Res Commun. 1973 Aug 21;53(4):1289–1296. doi: 10.1016/0006-291x(73)90605-0. [DOI] [PubMed] [Google Scholar]
- Rosen B. P. Restoration of active transport in an Mg2+-adenosine triphosphatase-deficient mutant of Escherichia coli. J Bacteriol. 1973 Dec;116(3):1124–1129. doi: 10.1128/jb.116.3.1124-1129.1973. [DOI] [PMC free article] [PubMed] [Google Scholar]
- Simoni R. D., Postma P. W. The energetics of bacterial active transport. Annu Rev Biochem. 1975;44:523–554. doi: 10.1146/annurev.bi.44.070175.002515. [DOI] [PubMed] [Google Scholar]
- Simoni R. D., Shallenberger M. K. Coupling of energy to active transport of amino acids in Escherichia coli. Proc Natl Acad Sci U S A. 1972 Sep;69(9):2663–2667. doi: 10.1073/pnas.69.9.2663. [DOI] [PMC free article] [PubMed] [Google Scholar]
- Tanaka S., Lerner S. A., Lin E. C. Replacement of a phosphoenolpyruvate-dependent phosphotransferase by a nicotinamide adenine dinucleotide-linked dehydrogenase for the utilization of mannitol. J Bacteriol. 1967 Feb;93(2):642–648. doi: 10.1128/jb.93.2.642-648.1967. [DOI] [PMC free article] [PubMed] [Google Scholar]
- Tsuchiya T., Rosen B. P. Characterization of an active transport system for calcium in inverted membrane vesicles of Escherichia coli. J Biol Chem. 1975 Oct 10;250(19):7687–7692. [PubMed] [Google Scholar]
- Tsuchiya T., Rosen B. P. Energy transduction in Escherichia coli. The role of the Mg2+ATPase. J Biol Chem. 1975 Nov 10;250(21):8409–8415. [PubMed] [Google Scholar]
- West I. C., Mitchell P. The proton-translocating ATPase of Escherichia coli. FEBS Lett. 1974 Mar 15;40(1):1–4. doi: 10.1016/0014-5793(74)80880-x. [DOI] [PubMed] [Google Scholar]