Abstract
The obligate intracellular bacterium Rickettsia typhi was examined for its ability to generate and maintain an adenylate energy charge in an extracellular environment. Freshly purified organisms were incubated, at 34°C and pH 7.4, with or without glutamate and various other metabolites, and the levels of ATP, ADP, and AMP were determined. Of the metabolites tested, glutamate and glutamine were the most effective for the generation of ATP. In the presence of glutamate, there was a rapid increase in the level of ATP, followed by a moderate decrease during 150 min of incubation. The energy charge increased from a level of 0.2 to 0.5 to about 0.7 to 0.75, and then slowly declined to about 0.45 to 0.6. In the absence of glutamate, after an occasional initial surge in ATP level as the temperature was changed from 4 to 34°C, there was a sharp decline in both ATP and energy charge (to 0.1 and sometimes to 0.01). The rickettsiae maintained their ability to regenerate their energy charge upon the addition of glutamate for about 30 min, but this ability declined with further incubation. In contrast to Escherichia coli, the decline in ATP in R. typhi was accompanied by a sharp increase in the level of AMP and the total adenylate pool. No adenine or adenosine was recovered from rickettsiae incubated with labeled AMP, ADP, or ATP. From these experiments and the demonstration reported elsewhere that rickettsiae transport the adenine nucleotides, it can be concluded that the adenylate energy charge in R. typhi is governed by the salvage of the adenine nucleotides rather than their unphosphorylated precursors. Thus, R. typhi undergoes greater shifts in energy charge than other bacteria, a phenomenon which may account for their instability in an extracellular environment. Under optimal conditions the adenylate energy charge of R. typhi approaches levels that border on those generally regarded as adequate for growth.
Full text
PDF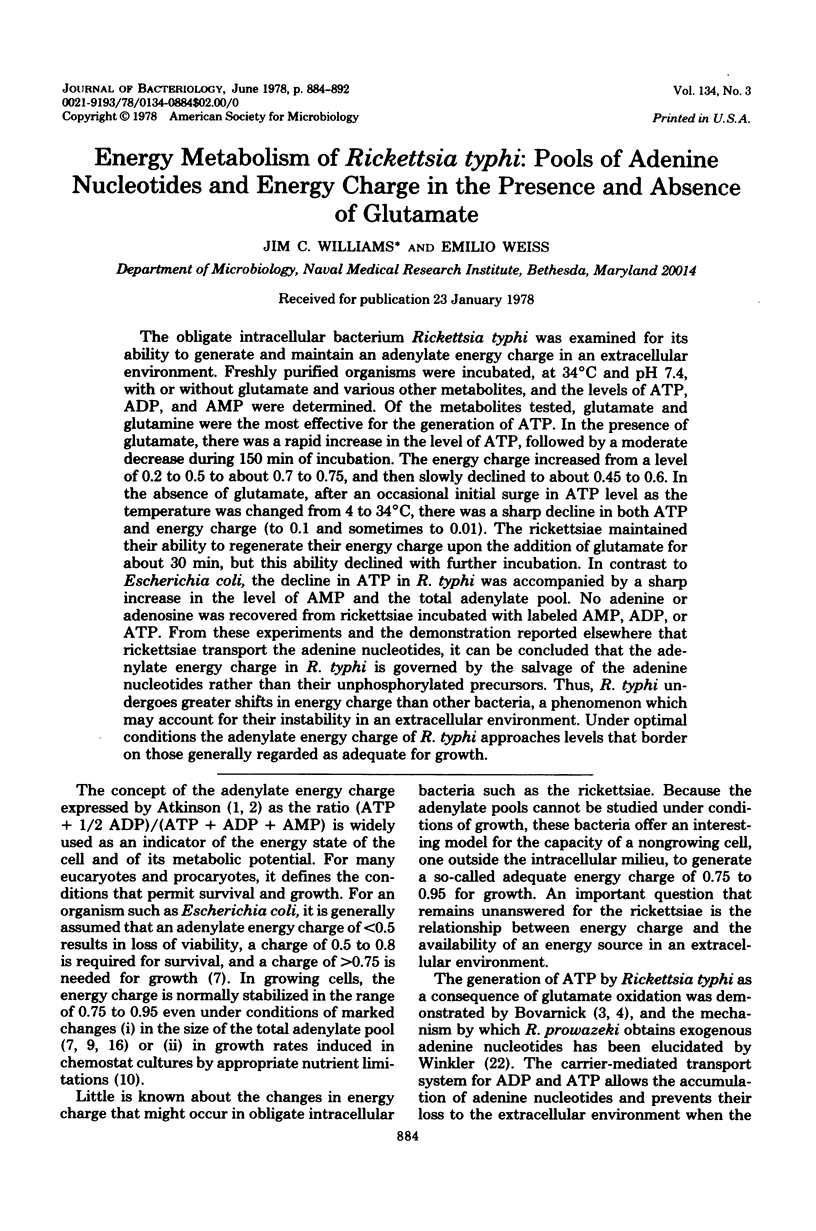
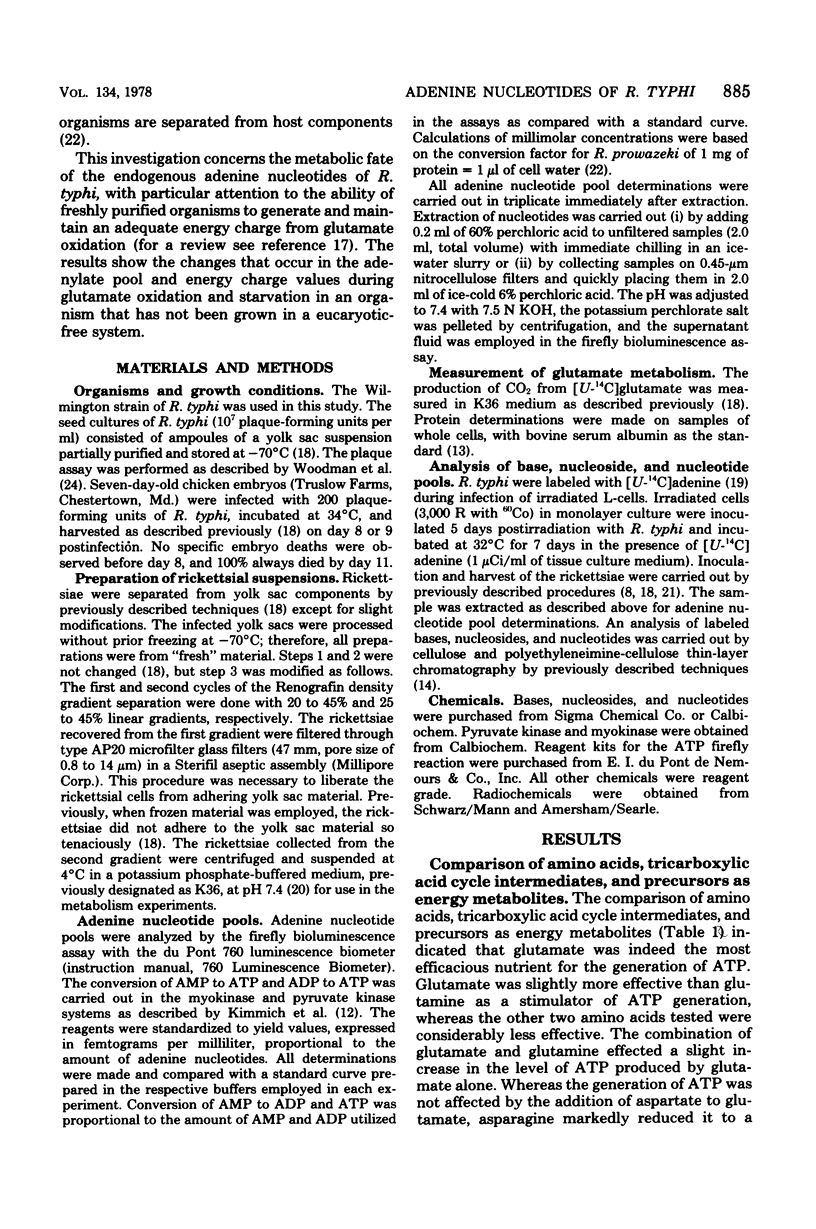
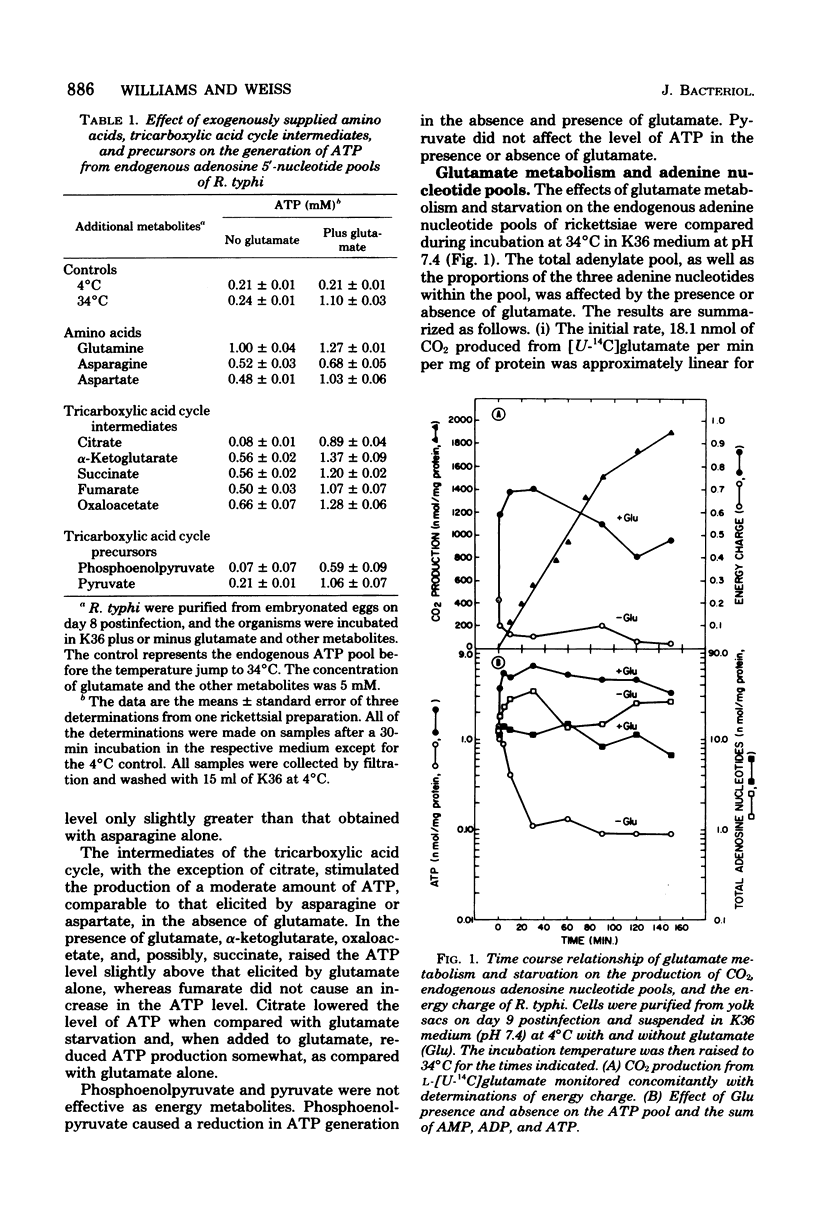
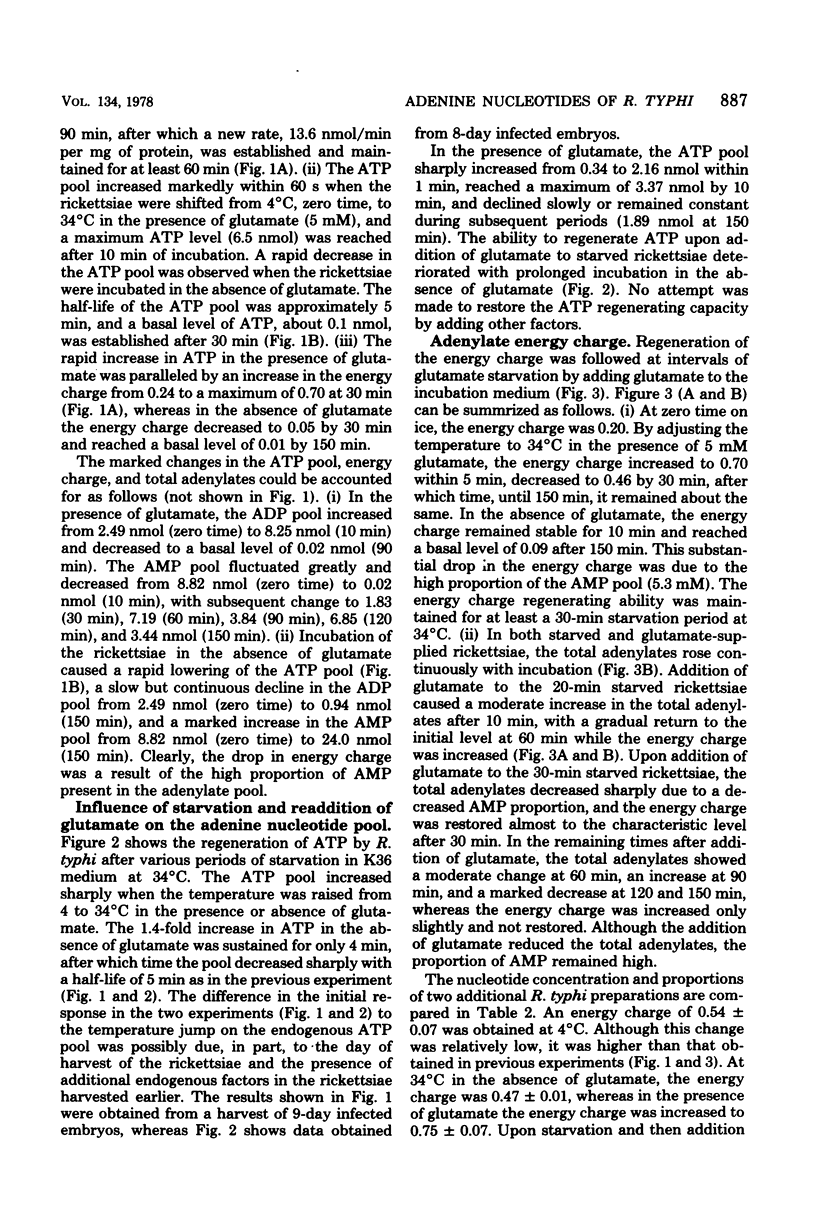
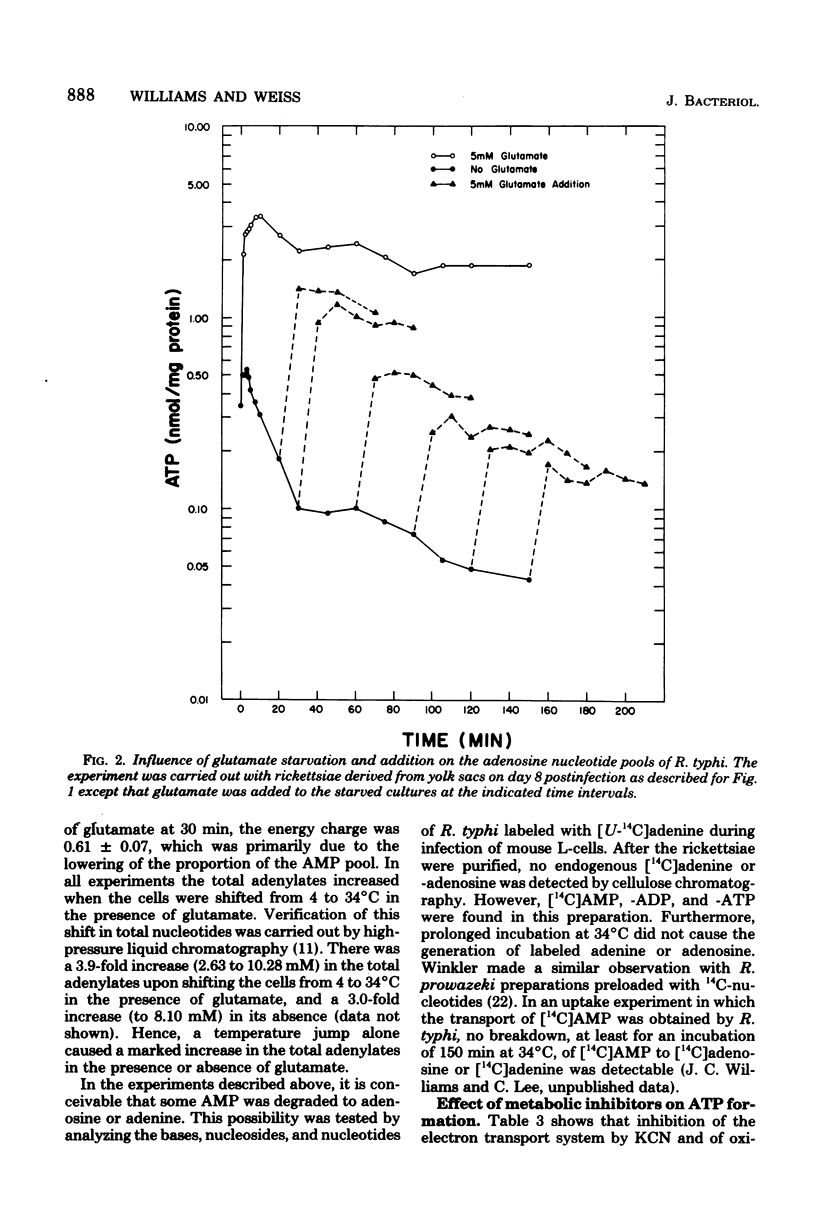
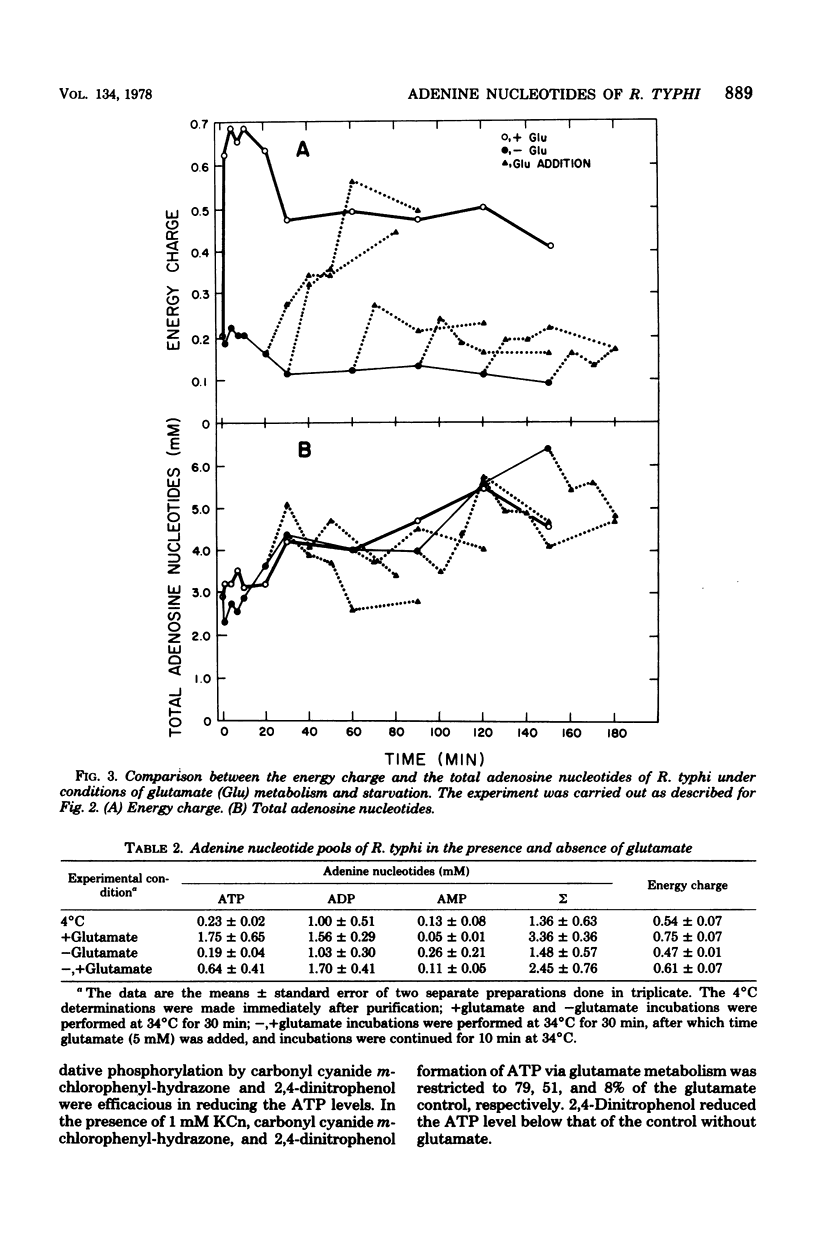
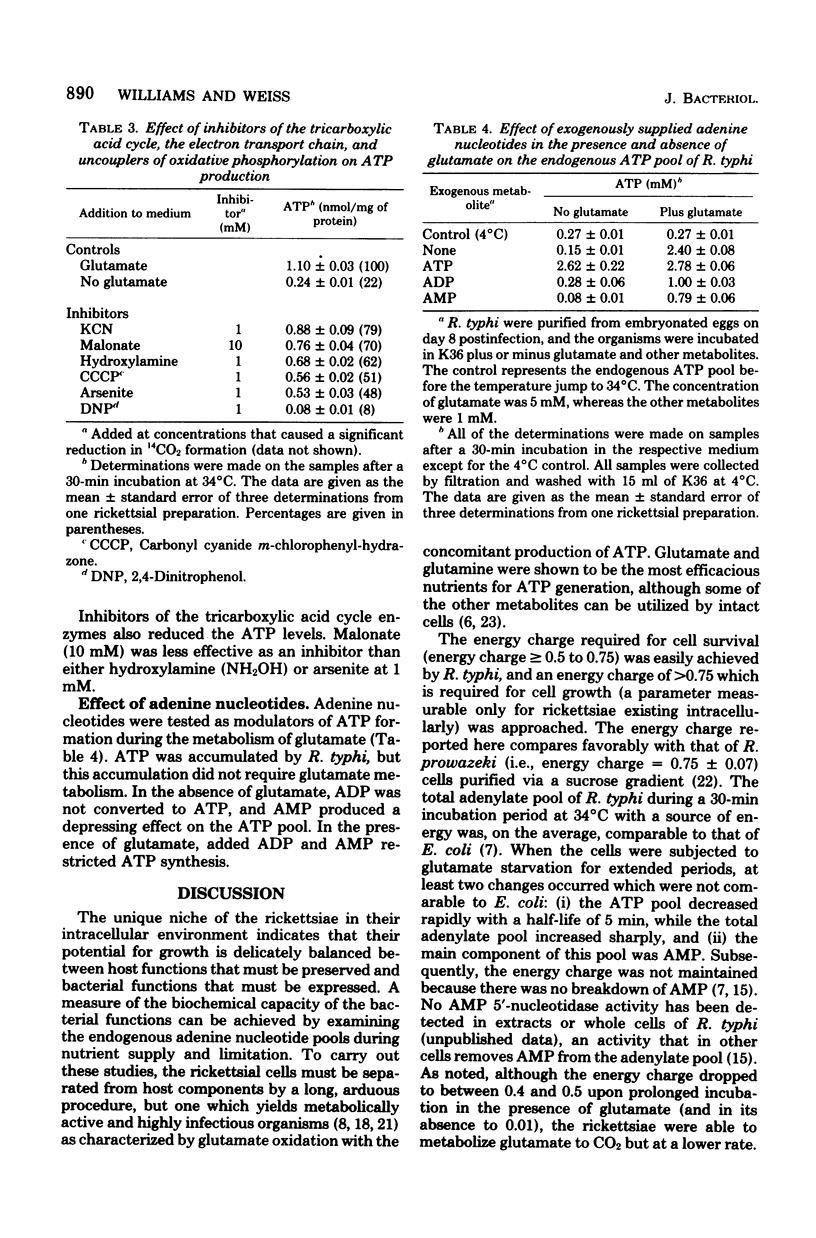
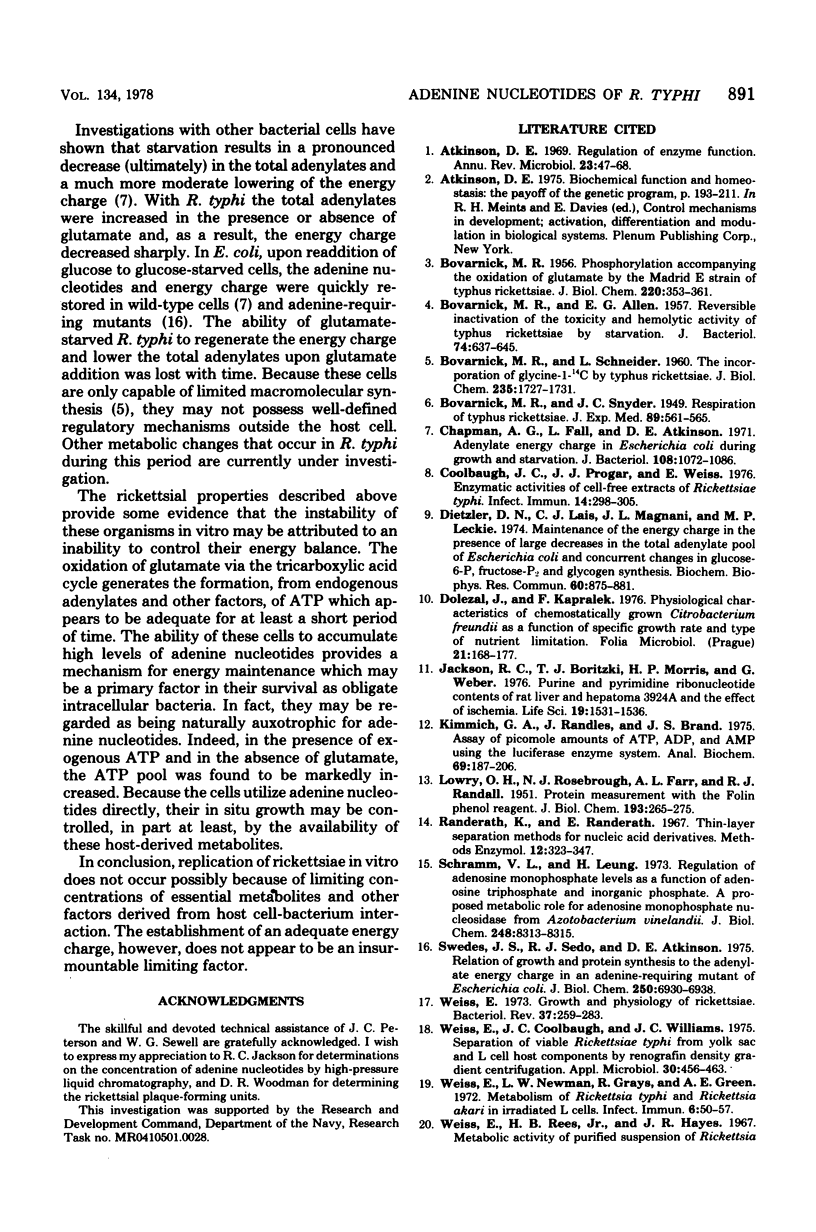
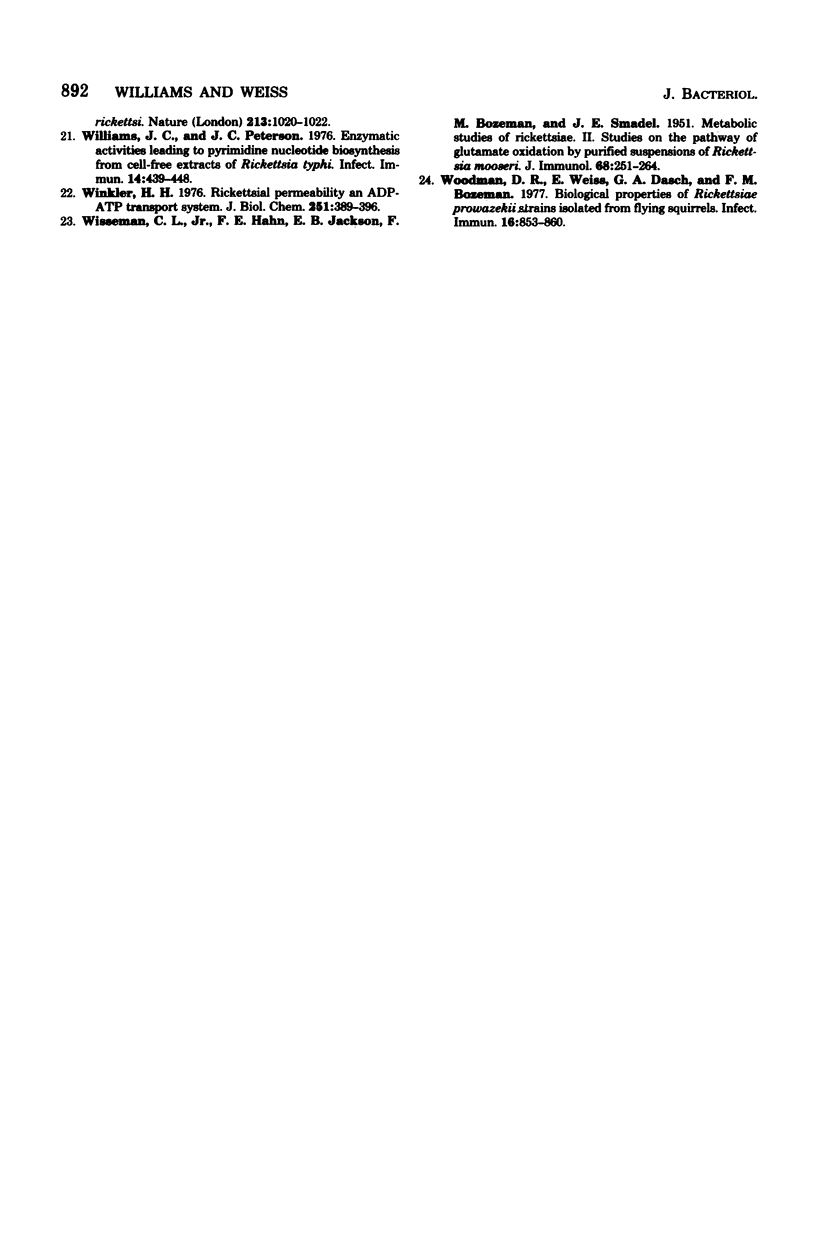
Selected References
These references are in PubMed. This may not be the complete list of references from this article.
- Atkinson D. E. Biochemical function and homeostasis: the payoff of the genetic program. Adv Exp Med Biol. 1975;62:193–211. doi: 10.1007/978-1-4684-3255-8_11. [DOI] [PubMed] [Google Scholar]
- Atkinson D. E. Regulation of enzyme function. Annu Rev Microbiol. 1969;23:47–68. doi: 10.1146/annurev.mi.23.100169.000403. [DOI] [PubMed] [Google Scholar]
- BOVARNICK M. R., ALLEN E. G. Reversible inactivation of the toxicity and hemolytic activity of typhus rickettsiae by starvation. J Bacteriol. 1957 Nov;74(5):637–645. doi: 10.1128/jb.74.5.637-645.1957. [DOI] [PMC free article] [PubMed] [Google Scholar]
- BOVARNICK M. R. Phosphorylation accompanying the oxidation of glutamate by the Madrid E strain of typhus rickettsiae. J Biol Chem. 1956 May;220(1):353–361. [PubMed] [Google Scholar]
- BOVARNICK M. R., SCHNEIDER L. The incorporation of glycine-1-C14 by typhus rickettsiae. J Biol Chem. 1960 Jun;235:1727–1731. [PubMed] [Google Scholar]
- Chapman A. G., Fall L., Atkinson D. E. Adenylate energy charge in Escherichia coli during growth and starvation. J Bacteriol. 1971 Dec;108(3):1072–1086. doi: 10.1128/jb.108.3.1072-1086.1971. [DOI] [PMC free article] [PubMed] [Google Scholar]
- Coolbaugh J. C., Progar J. J., Weiss E. Enzymatic activities of cell-free extracts of Rickettsia typhi. Infect Immun. 1976 Jul;14(1):298–305. doi: 10.1128/iai.14.1.298-305.1976. [DOI] [PMC free article] [PubMed] [Google Scholar]
- Dolezal J., Kaprálek F. Physiological characteristics of chemostatically grown Citrobacter freundii as a function of the specific growth rate and type of nutrient limitation. Folia Microbiol (Praha) 1976;21(3):168–177. doi: 10.1007/BF02883152. [DOI] [PubMed] [Google Scholar]
- Goodwin C. S., Tyrrell D. A., Head B., Rees R. J. Inhibition of haemaggregation by lepromin and other mycobacterial substances. Nature. 1967 Dec 9;216(5119):1019–1020. doi: 10.1038/2161019a0. [DOI] [PubMed] [Google Scholar]
- Jackson R. C., Boritzki T. J., Morris H. P., Weber G. Purine and pyrimidine ribonucleotide contents of rat liver and hepatoma 3924A and the effect of ischemia. Life Sci. 1976 Nov 15;19(10):1531–1536. doi: 10.1016/0024-3205(76)90098-9. [DOI] [PubMed] [Google Scholar]
- Kimmich G. A., Randles J., Brand J. S. Assay of picomole amounts of ATP, ADP, and AMP using the luciferase enzyme system. Anal Biochem. 1975 Nov;69(1):187–206. doi: 10.1016/0003-2697(75)90580-1. [DOI] [PubMed] [Google Scholar]
- LOWRY O. H., ROSEBROUGH N. J., FARR A. L., RANDALL R. J. Protein measurement with the Folin phenol reagent. J Biol Chem. 1951 Nov;193(1):265–275. [PubMed] [Google Scholar]
- Schramm V. L., Leung H. Regulation of adenosine monophosphate levels as a function of adenosine triphosphate and inorganic phosphate. A proposed metabolic role for adenosine monophosphate nucleosidase from Azotobacter vinelandii. J Biol Chem. 1973 Dec 10;248(23):8313–8315. [PubMed] [Google Scholar]
- Swedes J. S., Sedo R. J., Atkinson D. E. Relation of growth and protein synthesis to the adenylate energy charge in an adenine-requiring mutant of Escherichia coli. J Biol Chem. 1975 Sep 10;250(17):6930–6938. [PubMed] [Google Scholar]
- WISSEMAN C. L., Jr, HAHN F. E., JACKSON E. B., BOZEMAN M. F., SMADEL J. E. Metabolic studies of rickettsiae. II. Studies on the pathway of glutamate oxidation by purified suspensions of Rickettsia mooseri. J Immunol. 1952 Mar;68(3):251–264. [PubMed] [Google Scholar]
- Weiss E., Coolbaugh J. C., Williams J. C. Separation of viable Rickettsia typhi from yolk sac and L cell host components by renografin density gradient centrifugation. Appl Microbiol. 1975 Sep;30(3):456–463. doi: 10.1128/am.30.3.456-463.1975. [DOI] [PMC free article] [PubMed] [Google Scholar]
- Weiss E. Growth and physiology of rickettsiae. Bacteriol Rev. 1973 Sep;37(3):259–283. doi: 10.1128/br.37.3.259-283.1973. [DOI] [PMC free article] [PubMed] [Google Scholar]
- Weiss E., Newman L. W., Grays R., Green A. E. Metabolism of Rickettsia typhi and Rickettsia akari in irradiated L cells. Infect Immun. 1972 Jul;6(1):50–57. doi: 10.1128/iai.6.1.50-57.1972. [DOI] [PMC free article] [PubMed] [Google Scholar]
- Williams J. C., Peterson J. C. Enzymatic activities leading to pyrimidine nucleotide biosynthesis from cell-free extracts of Rickettsia typhi. Infect Immun. 1976 Aug;14(2):439–448. doi: 10.1128/iai.14.2.439-448.1976. [DOI] [PMC free article] [PubMed] [Google Scholar]
- Winkler H. H. Rickettsial permeability. An ADP-ATP transport system. J Biol Chem. 1976 Jan 25;251(2):389–396. [PubMed] [Google Scholar]
- Woodman D. R., Weiss E., Dasch G. A., Bozeman F. M. Biological properties of Rickettsia prowazekii strains isolated from flying squirrels. Infect Immun. 1977 Jun;16(3):853–860. doi: 10.1128/iai.16.3.853-860.1977. [DOI] [PMC free article] [PubMed] [Google Scholar]