Abstract
Galactose-grown cells of Streptococcus lactis ML3 acculated alpha-aminoisobutyric acid (AIB) by using energy derived from glycolysis and arginine catabolism. The transport system displayed low-affinity Michaelis-Menten saturation kinetics. Using galactose or arginine as energy sources, similar V max and K m values for AIB entry were obtained, but on prolonged incubation the intracellular steady-state concentration of AIB in cells metabolizing arginine was only 65 to 70% that attained by glycolyzing cells. Efflux of AIB FROM PRELOADED CElls was temperature dependent and exhibited the characteristics of a first-order reaction. The rate of AIB exit was accelerated two- to threefold in the presence of metabolizable energy sources. Metabolic inhibitors including p-chloromercuribenzoate, dinitrophenol, azide, arsentate, and N, N'-dicyclohexylcarbodiimide either prevented or greatly reduced AIB uptake. Fluoride, iodoacetate and N-ethylmaleimide abolished galactose-dependent, but not arginine-energized, AIB uptake. K+ and Rb+ reduced the steady-state intracellular AIB concentration by approximately 40%, and these cations also induced rapid efflux of solute from actively transporting cells. Equivalent concentrations (10 mM) of Na+, Li+, or NH4+ were much less inhibitory. The proton-conducting ionophores tetrachlorosalicylanilide and carbonylcyanide m-chlorophenlyhydrazone abolished uptake and induced AIB efflux even though glycolysis and arginine catabolism continued at 60 and 140%, respectively, of control rates. A proton motive force is most likely involved in the active transport of AIB, whereas data from efflux studies suggest that energy is coupled to AIB exit in cells of S. lactis ML3.
Full text
PDF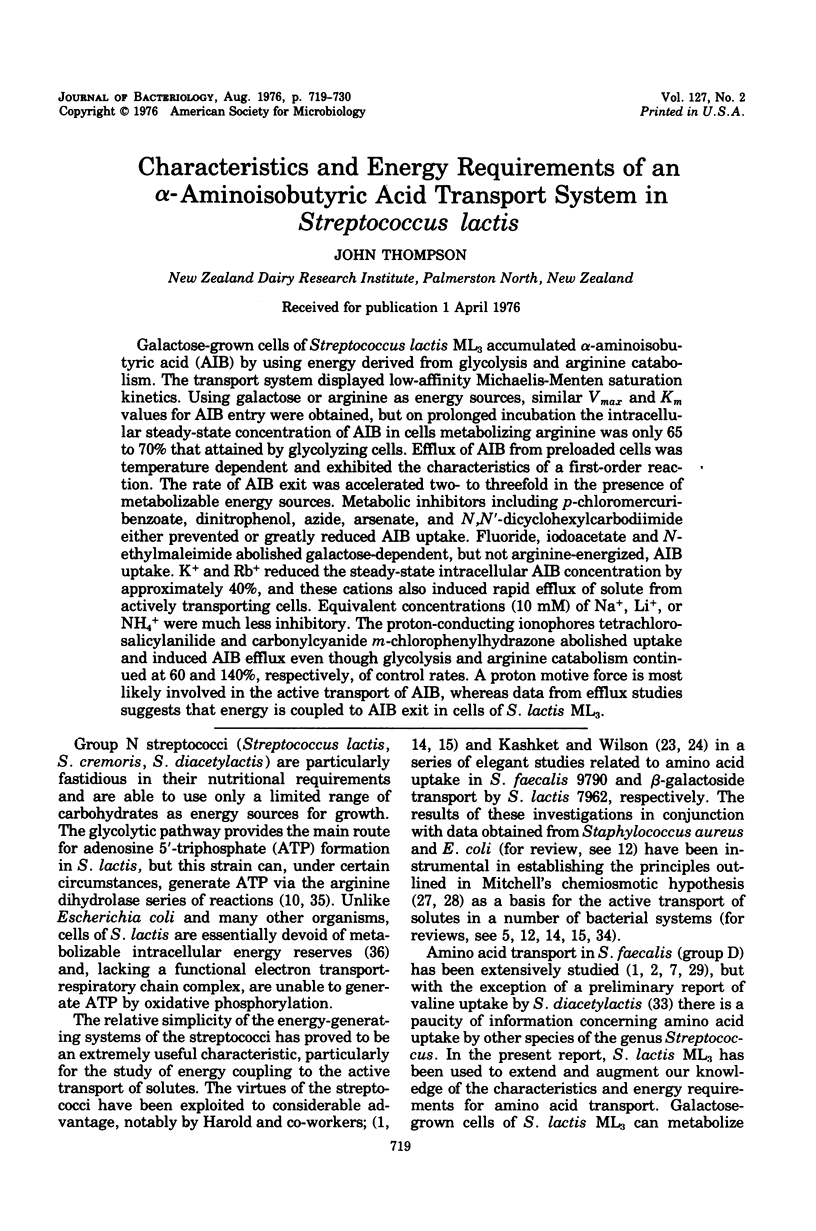
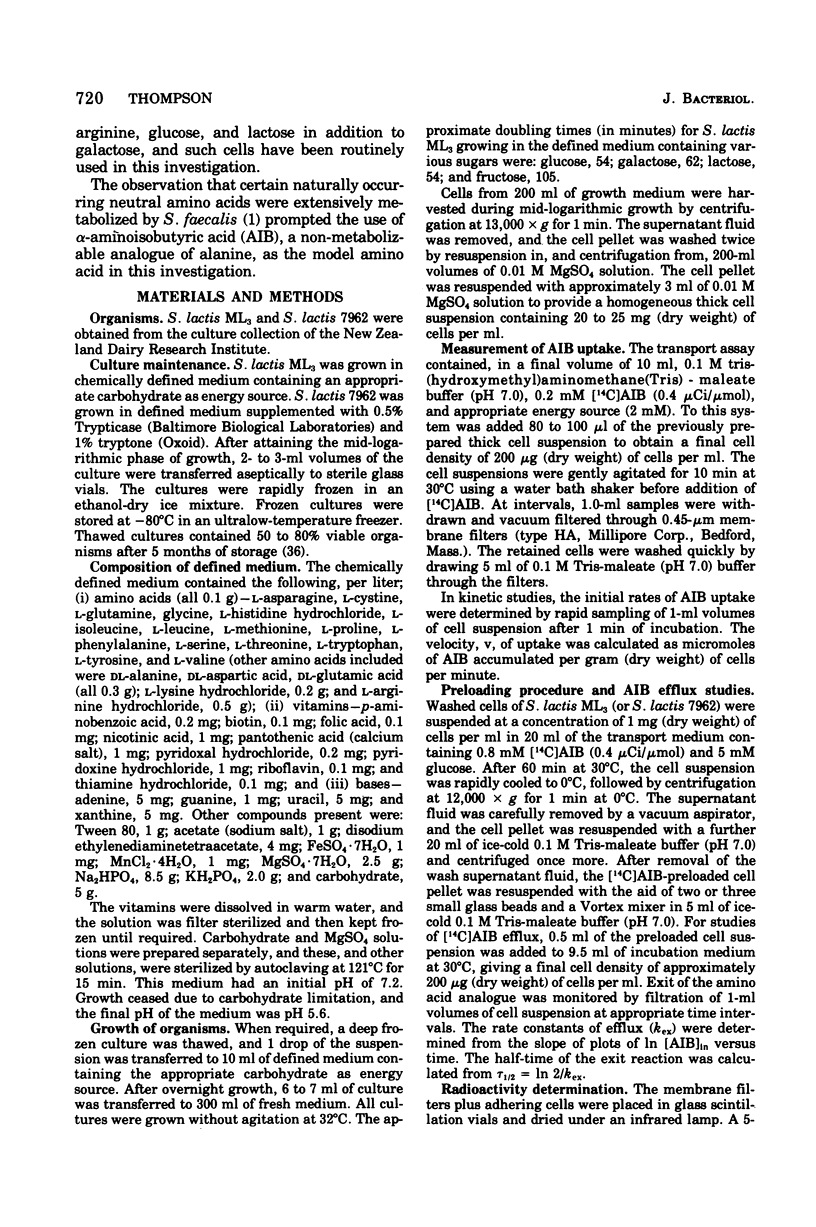
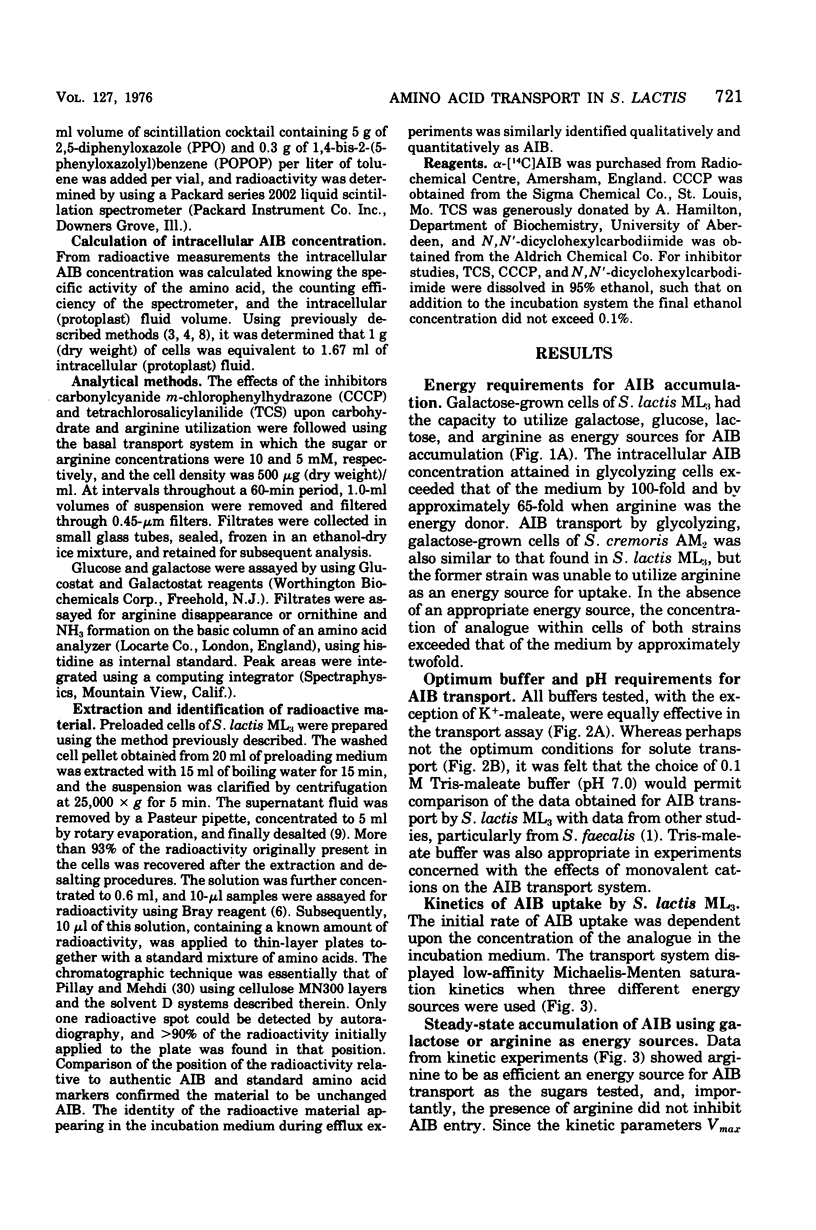
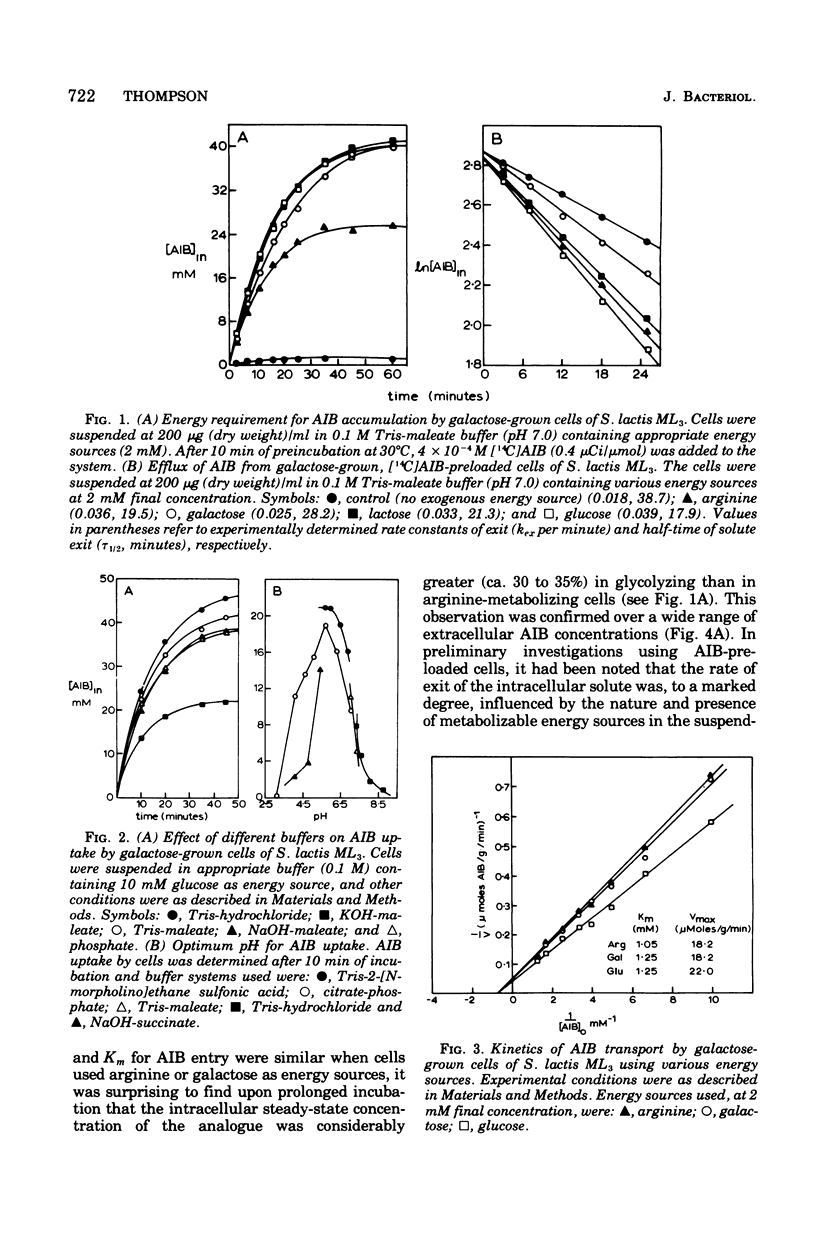
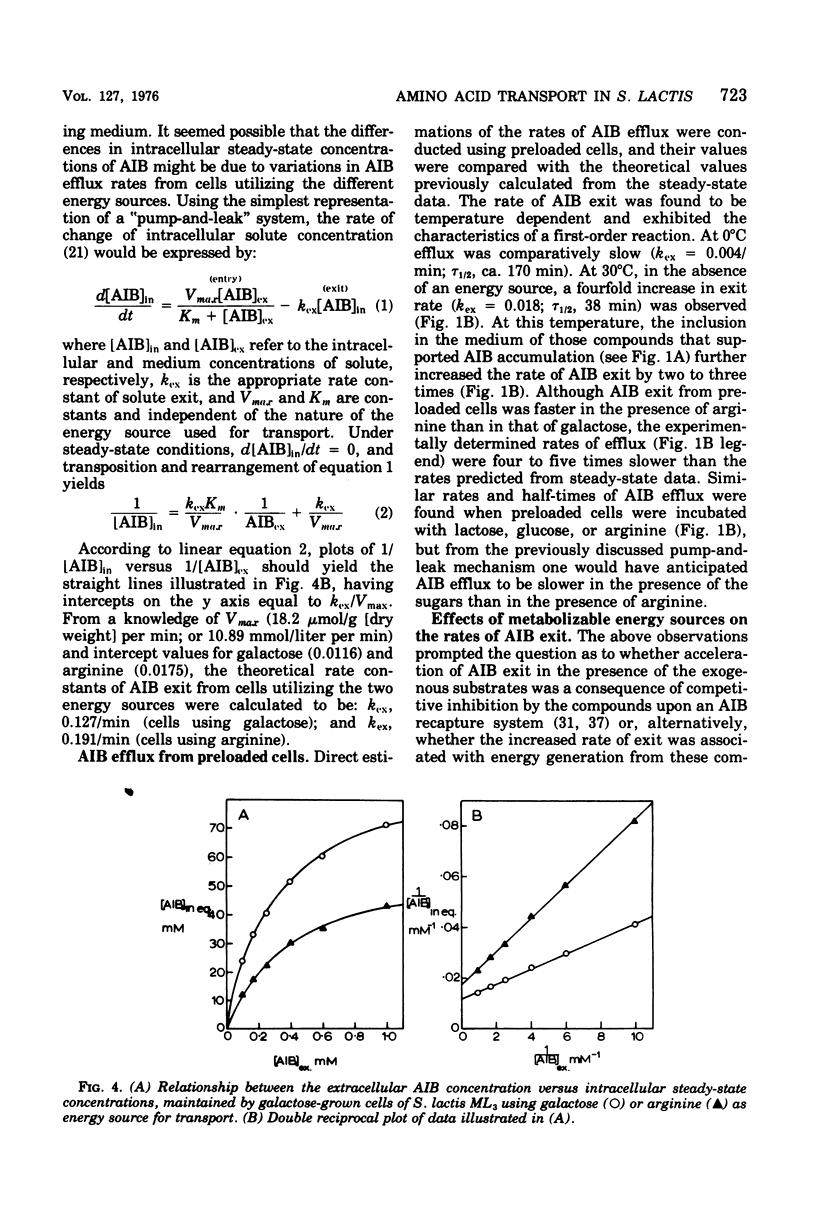
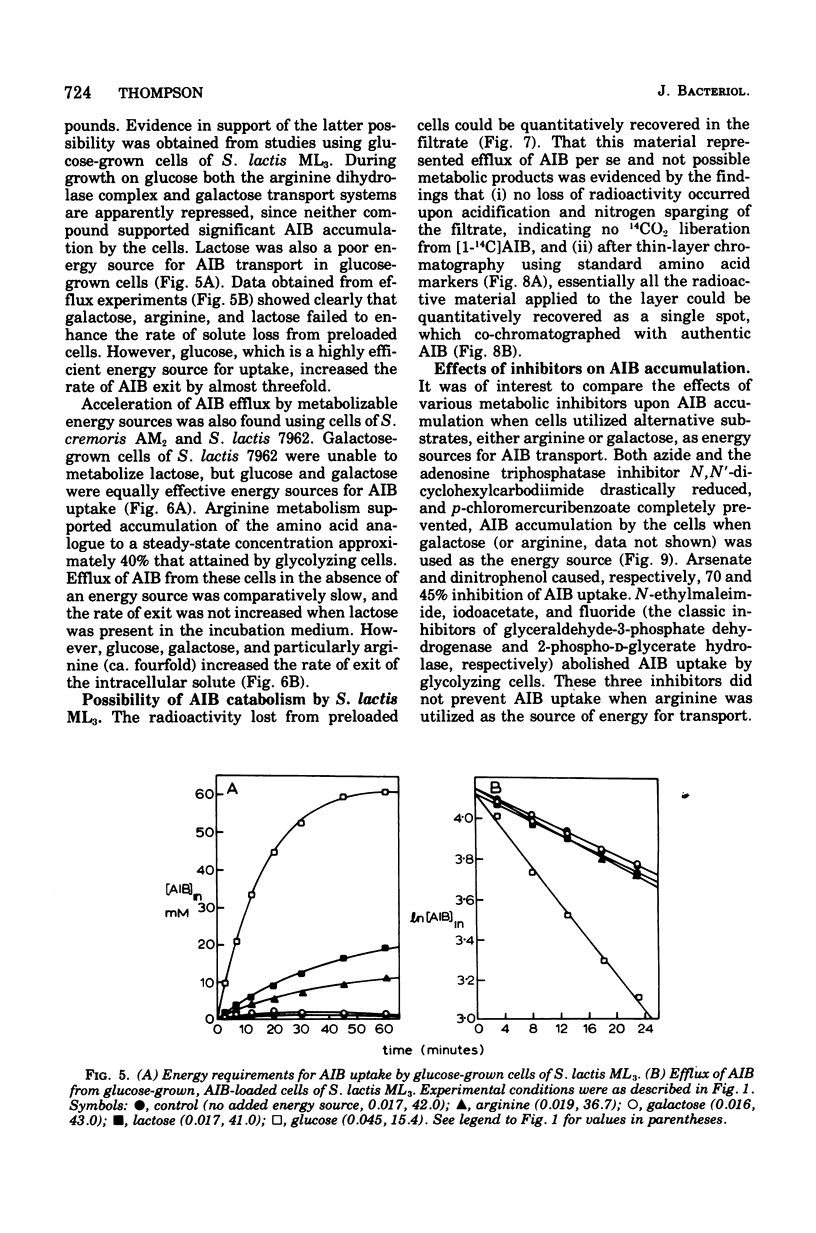
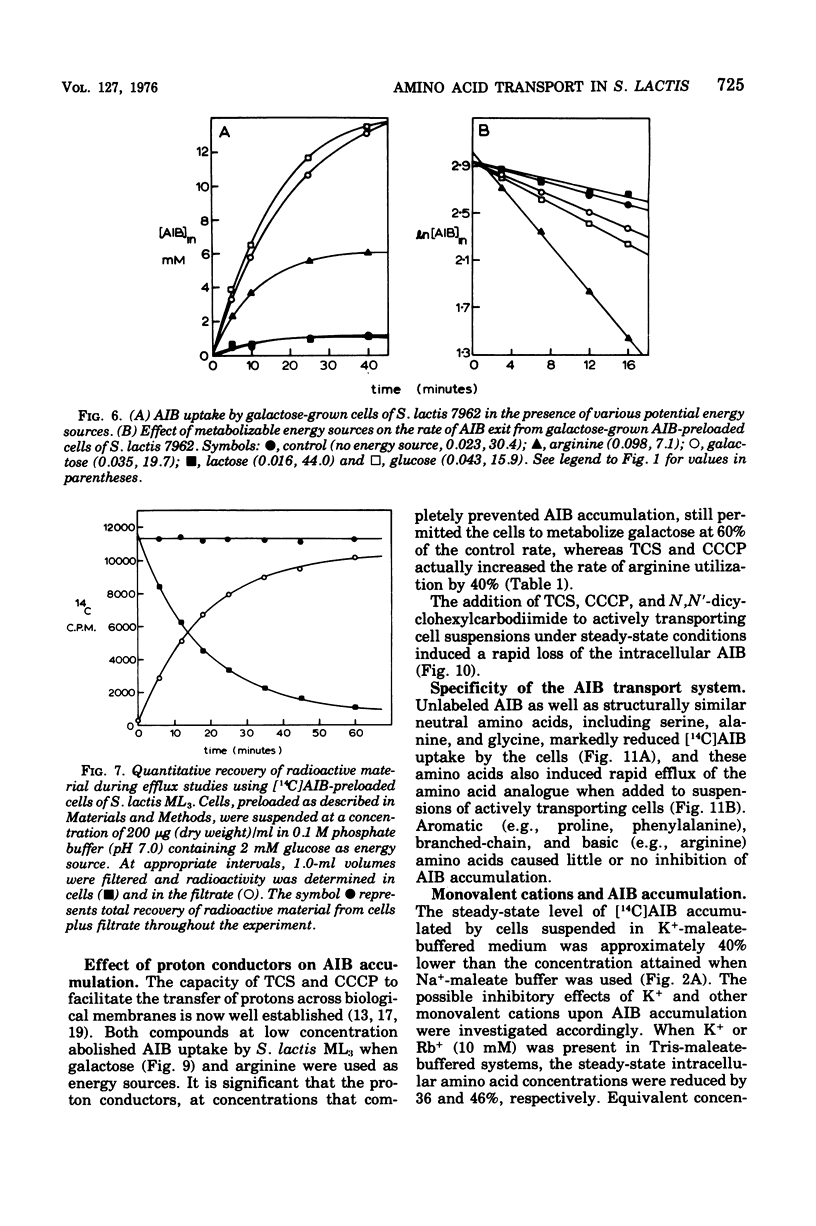
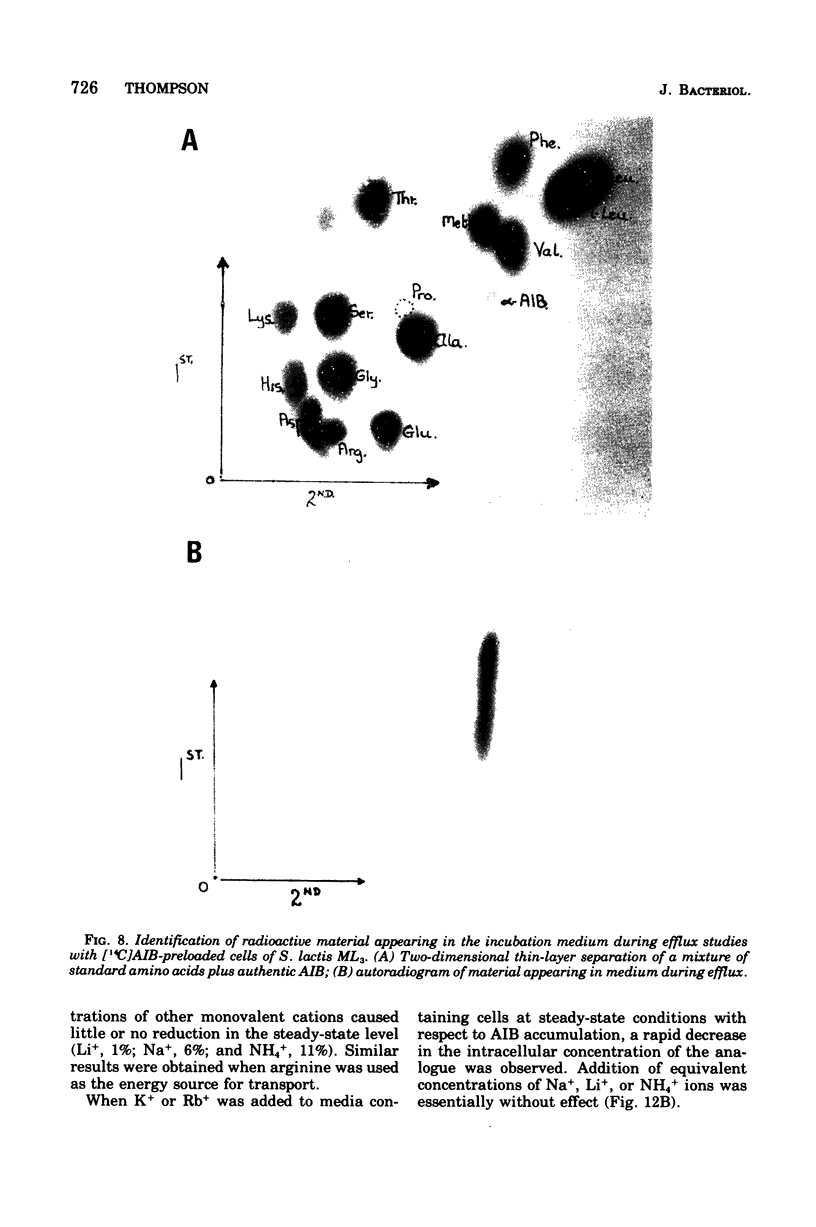
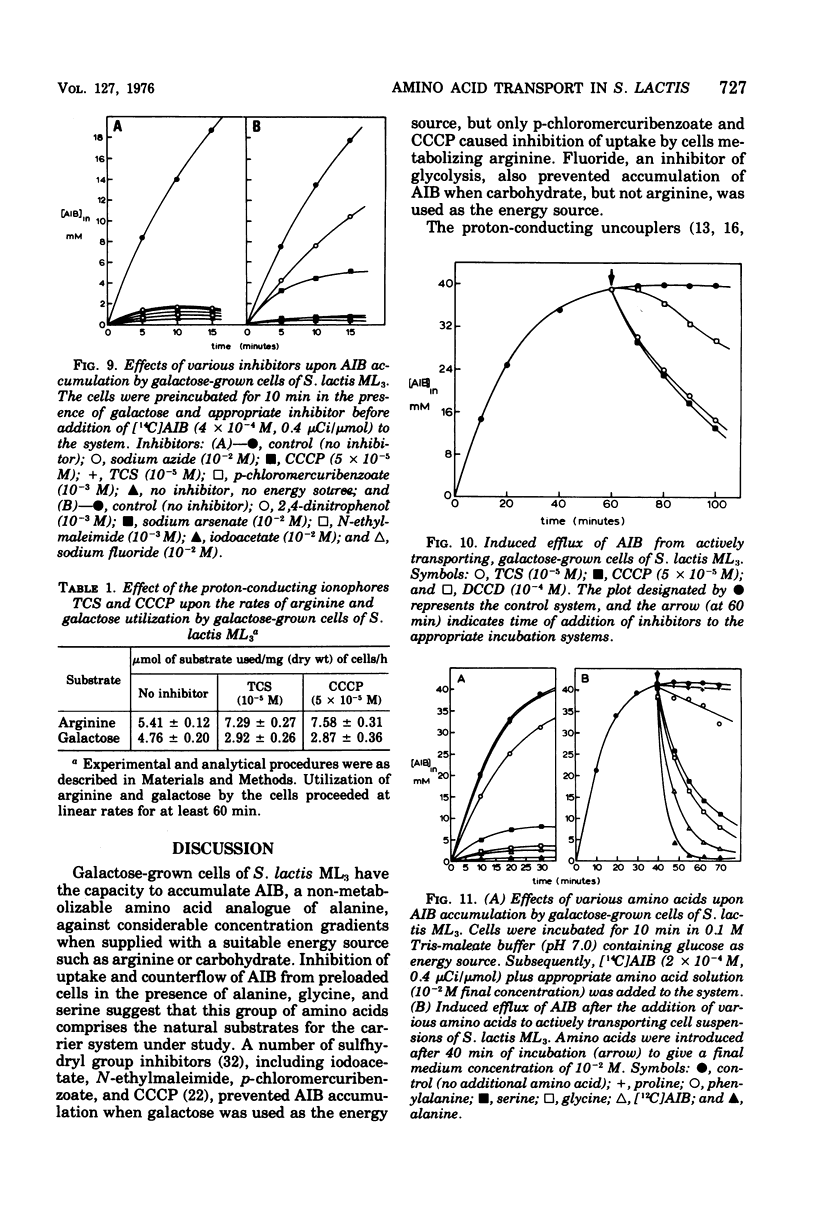
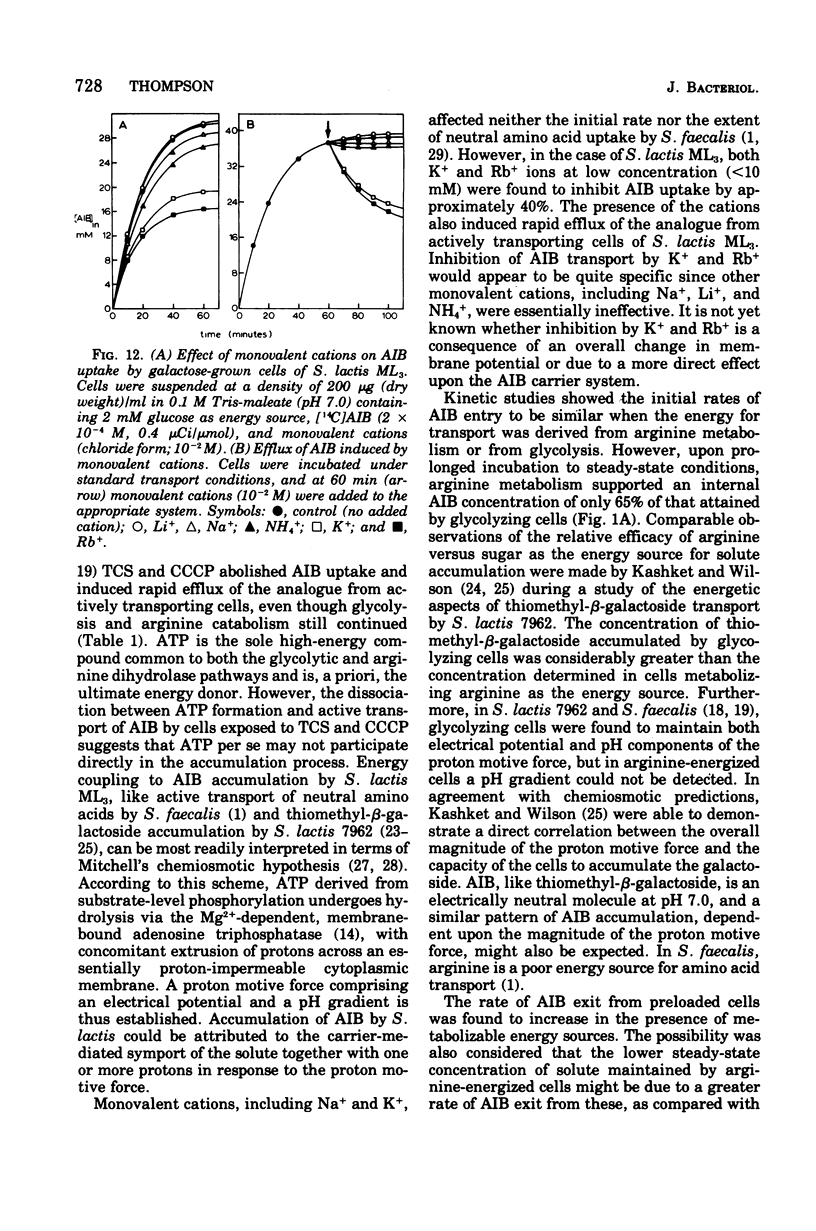
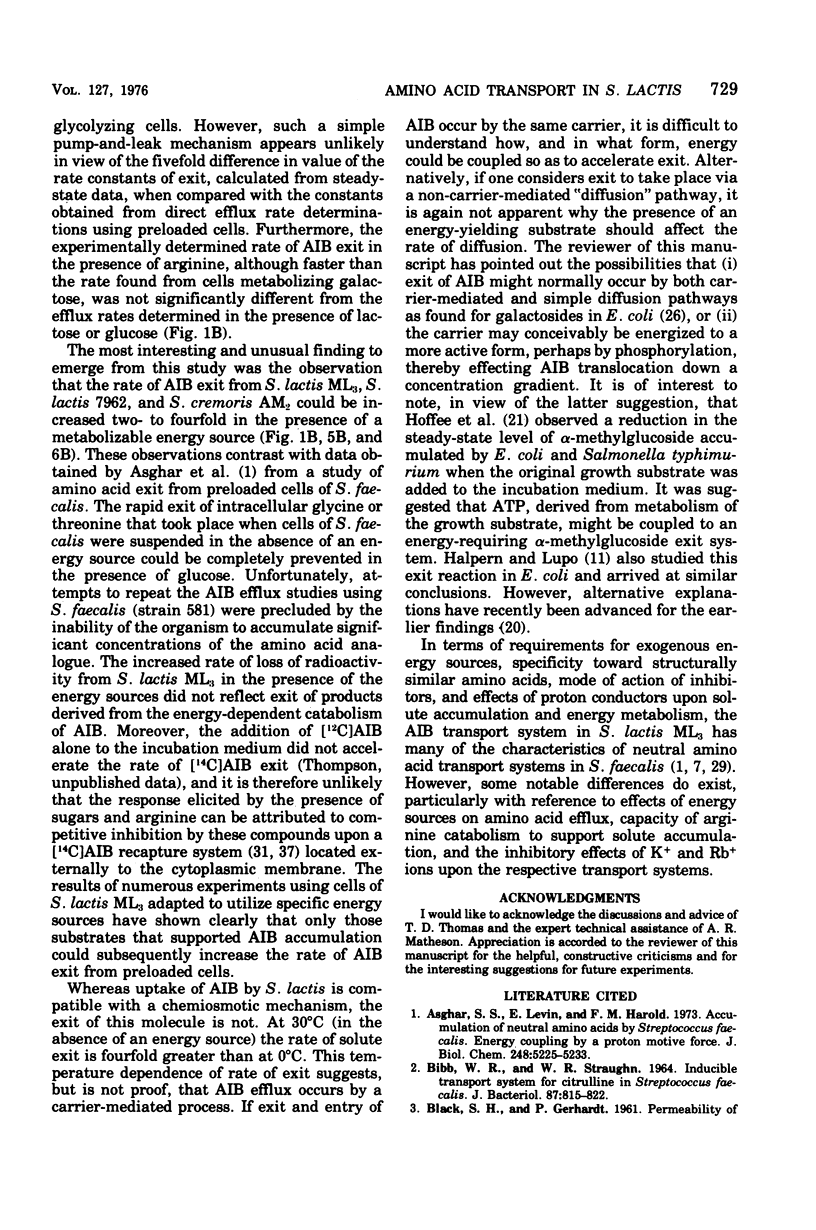
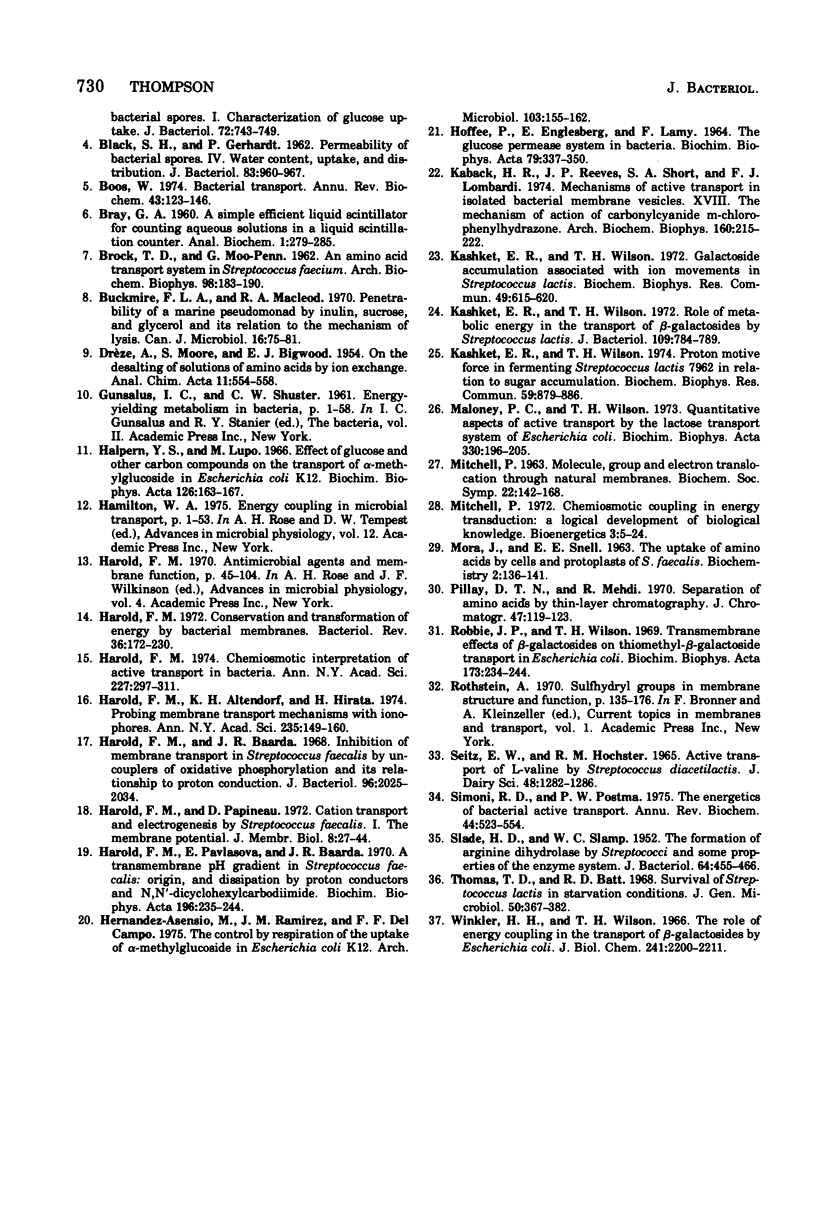
Images in this article
Selected References
These references are in PubMed. This may not be the complete list of references from this article.
- Asghar S. S., Levin E., Harold F. M. Accumulation of neutral amino acids by Streptococcus faecalis. Energy coupling by a proton-motive force. J Biol Chem. 1973 Aug 10;248(15):5225–5233. [PubMed] [Google Scholar]
- BIBB W. R., STRAUGHN W. R. INDUCIBLE TRANSPORT SYSTEM FOR CITRULLINE IN STREPTOCOCCUS FAECALIS. J Bacteriol. 1964 Apr;87:815–822. doi: 10.1128/jb.87.4.815-822.1964. [DOI] [PMC free article] [PubMed] [Google Scholar]
- BLACK S. H., GERHARDT P. Permeability of bacterial spores. IV. Water content, uptake, and distribution. J Bacteriol. 1962 May;83:960–967. doi: 10.1128/jb.83.5.960-967.1962. [DOI] [PMC free article] [PubMed] [Google Scholar]
- BROCK T. D., MOO-PENN G. An amino acid transport system in Streptococcus faecium. Arch Biochem Biophys. 1962 Aug;98:183–190. doi: 10.1016/0003-9861(62)90171-6. [DOI] [PubMed] [Google Scholar]
- Boos W. Bacterial transport. Annu Rev Biochem. 1974;43(0):123–146. doi: 10.1146/annurev.bi.43.070174.001011. [DOI] [PubMed] [Google Scholar]
- Buckmire F. L., MacLeod R. A. Penetrability of a marine pseudomonad by inulin, sucrose, and glycerol and its relation to the mechanism of lysis. Can J Microbiol. 1970 Feb;16(2):75–81. doi: 10.1139/m70-014. [DOI] [PubMed] [Google Scholar]
- HOFFEE P., ENGLESBERG E., LAMY F. THE GLUCOSE PERMEASE SYSTEM IN BACTERIA. Biochim Biophys Acta. 1964 Mar 30;79:337–350. [PubMed] [Google Scholar]
- Halpern Y. S., Lupo M. Effect of glucose and other carbon compounds on the transport of alpha-methylglucoside in Escherichia coli K12. Biochim Biophys Acta. 1966 Sep 5;126(1):163–167. doi: 10.1016/0926-6585(66)90046-x. [DOI] [PubMed] [Google Scholar]
- Harold F. M., Altendorf K. H., Hirata H. Probing membrane transport mechanisms with inophores. Ann N Y Acad Sci. 1974 May 10;235(0):149–160. doi: 10.1111/j.1749-6632.1974.tb43264.x. [DOI] [PubMed] [Google Scholar]
- Harold F. M., Baarda J. R. Inhibition of membrane transport in Streptococcus faecalis by uncouplers of oxidative phosphorylation and its relationship to proton conduction. J Bacteriol. 1968 Dec;96(6):2025–2034. doi: 10.1128/jb.96.6.2025-2034.1968. [DOI] [PMC free article] [PubMed] [Google Scholar]
- Harold F. M. Chemiosmotic interpretation of active transport in bacteria. Ann N Y Acad Sci. 1974 Feb 18;227:297–311. doi: 10.1111/j.1749-6632.1974.tb14395.x. [DOI] [PubMed] [Google Scholar]
- Harold F. M. Conservation and transformation of energy by bacterial membranes. Bacteriol Rev. 1972 Jun;36(2):172–230. doi: 10.1128/br.36.2.172-230.1972. [DOI] [PMC free article] [PubMed] [Google Scholar]
- Harold F. M., Papineau D. Cation transport and electrogenesis by Streptococcus faecalis. I. The membrane potential. J Membr Biol. 1972;8(1):27–44. doi: 10.1007/BF01868093. [DOI] [PubMed] [Google Scholar]
- Harold F. M., Pavlasová E., Baarda J. R. A transmembrane pH gradient in Streptococcus faecalis: origin, and dissipation by proton conductors and N,N'-dicyclohexylcarbodimide. Biochim Biophys Acta. 1970;196(2):235–244. doi: 10.1016/0005-2736(70)90011-8. [DOI] [PubMed] [Google Scholar]
- Hernandez-Asensio M., Ramirez J. M., Del Campo F. F. The control by respiration of the uptake of alpha-methyl glucoside in Escherichia coli K12. Arch Microbiol. 1975 Apr 7;103(2):155–162. doi: 10.1007/BF00436343. [DOI] [PubMed] [Google Scholar]
- Kaback H. R., Reeves J. P., Short S. A., Lombardi F. J. Mechanisms of active transport in isolated bacterial membrane vesicles. 18. The mechanism of action of carbonylcyanide m-chlorophenylhydrazone. Arch Biochem Biophys. 1974 Jan;160(1):215–222. doi: 10.1016/s0003-9861(74)80028-7. [DOI] [PubMed] [Google Scholar]
- Kashket E. R., Wilson T. H. Galactoside accumulation associated with ion movements in Streptococcus lactis. Biochem Biophys Res Commun. 1972 Nov 1;49(3):615–620. doi: 10.1016/0006-291x(72)90455-x. [DOI] [PubMed] [Google Scholar]
- Kashket E. R., Wilson T. H. Protonmotive force in fermenting Streptococcus lactis 7962 in relation to sugar accumulation. Biochem Biophys Res Commun. 1974 Aug 5;59(3):879–886. doi: 10.1016/s0006-291x(74)80061-6. [DOI] [PubMed] [Google Scholar]
- Kashket E. R., Wilson T. H. Role of metabolic energy in the transport of -galactosides by Streptococcus lactis. J Bacteriol. 1972 Feb;109(2):784–789. doi: 10.1128/jb.109.2.784-789.1972. [DOI] [PMC free article] [PubMed] [Google Scholar]
- Maloney P. C., Wilson T. H. Quantitative aspects of active transport by the lactose transport system of Escherichia coli. Biochim Biophys Acta. 1973 Dec 13;330(2):196–205. doi: 10.1016/0005-2736(73)90225-3. [DOI] [PubMed] [Google Scholar]
- Mitchell P. Chemiosmotic coupling in energy transduction: a logical development of biochemical knowledge. J Bioenerg. 1972 May;3(1):5–24. doi: 10.1007/BF01515993. [DOI] [PubMed] [Google Scholar]
- Pillay D. T., Mehdi R. Separation of amino acids by thin-layer chromatography. J Chromatogr. 1970 Feb 18;47(1):119–123. doi: 10.1016/0021-9673(70)80018-8. [DOI] [PubMed] [Google Scholar]
- Robbie J. P., Wilson T. H. Transmembrane effects of beta-galactosides on thiomethyl-beta-galactoside transport in Escherichia coli. Biochim Biophys Acta. 1969 Mar 11;173(2):234–244. doi: 10.1016/0005-2736(69)90107-2. [DOI] [PubMed] [Google Scholar]
- SLADE H. D., SLAMP W. C. The formation of arginine dihydrolase by streptococci and some properties of the enzyme system. J Bacteriol. 1952 Oct;64(4):455–466. doi: 10.1128/jb.64.4.455-466.1952. [DOI] [PMC free article] [PubMed] [Google Scholar]
- Seitz E. W., Hochster R. M. Active transport of L-valine by Streptococcus diacetilactis. J Dairy Sci. 1965 Oct;48(10):1282–1286. doi: 10.3168/jds.S0022-0302(65)88448-X. [DOI] [PubMed] [Google Scholar]
- Simoni R. D., Postma P. W. The energetics of bacterial active transport. Annu Rev Biochem. 1975;44:523–554. doi: 10.1146/annurev.bi.44.070175.002515. [DOI] [PubMed] [Google Scholar]
- Thomas T. D., Batt R. D. Survival of Streptococcus lactis in starvation conditions. J Gen Microbiol. 1968 Mar;50(3):367–382. doi: 10.1099/00221287-50-3-367. [DOI] [PubMed] [Google Scholar]
- Winkler H. H., Wilson T. H. The role of energy coupling in the transport of beta-galactosides by Escherichia coli. J Biol Chem. 1966 May 25;241(10):2200–2211. [PubMed] [Google Scholar]