Abstract
Photoproduction of H2 and activation of H2 for CO2 reduction (photoreduction) by Rhodopseudomonas capsulata are catalyzed by different enzyme systems. Formation of H2 from organic compounds is mediated by nitrogenase and is nto inhibited by an atmosphere of 99% H2. Cells grown photoheterotrophically on C4 dicarboxylic acids (with glutamate as N source) evolve H2 from the C4 acids and also from lactate and pyruvate; cells grown on C3 carbon sources, however, are inactive with the C4 acids, presumably because they lack inducible transport systems. Ammonia is known to inhibit N2 fixation by photosynthetic bacteria, and it also effectively prevents photoproduction of H2; these effects are due to inhibition and, in part, inactivation of nitrogenase. Biosynthesis of the latter, as measured by both H2 production and acetylene reduction assays, is markedly increased when cells are grown at high light intensity; synthesis of the photoreduction system, on the other hand, is not appreciably influenced by light intensity during photoheterotrophic growth. The photoreduction activity of cells grown on lactate + glutamate (which contain active nitrogenase) is greatly activated by NH4+, but this effect is not observed in cells grown with NH4+ as N source (nitrogenase repressed) or in a Nif- mutant that is unable to produce H2. Lactate, malate, and succinate, which are readily used as growth substrates by R. capsulata and are excellent H donors for photoproduction of H2, abolish photoreduction activity. The physiological significances of this phenomenon and of the reciprocal regulatory effects of NH4+ on H2 production and photoreduction are discussed.
Full text
PDF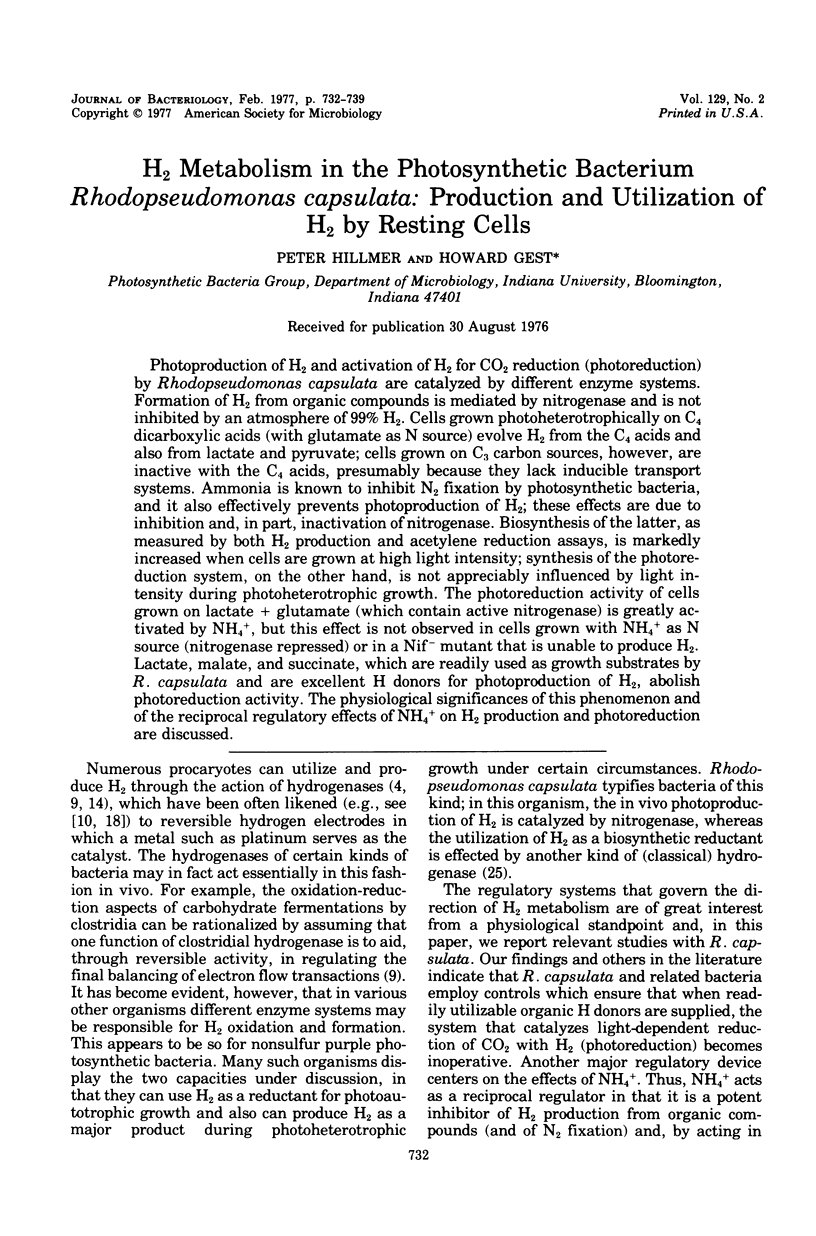
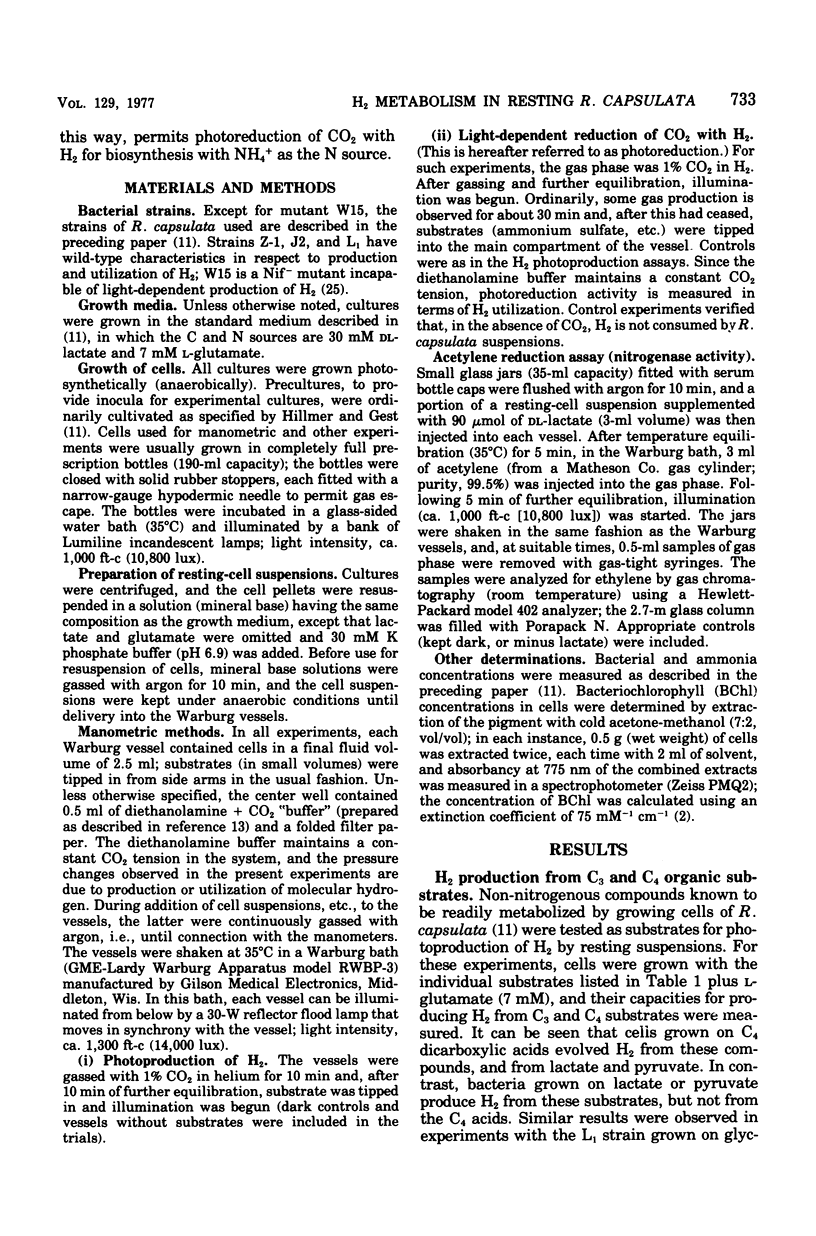
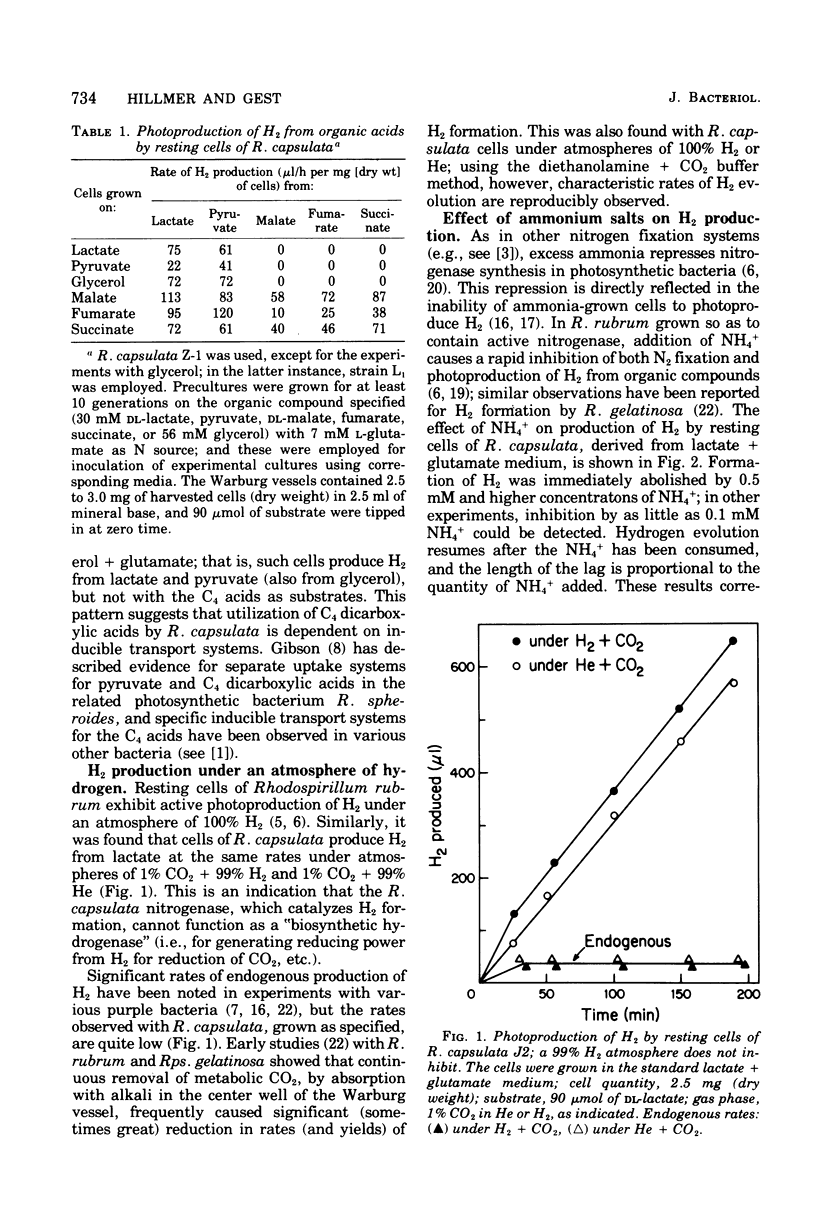
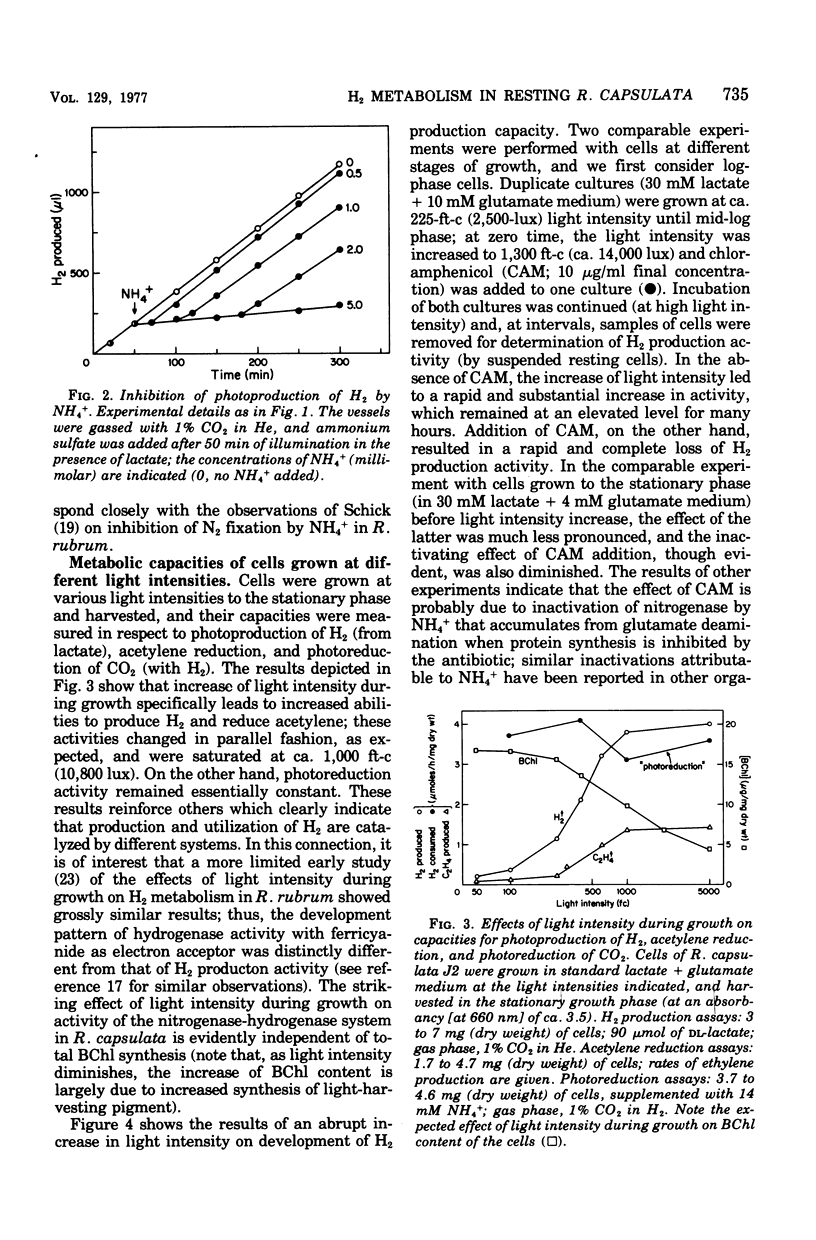
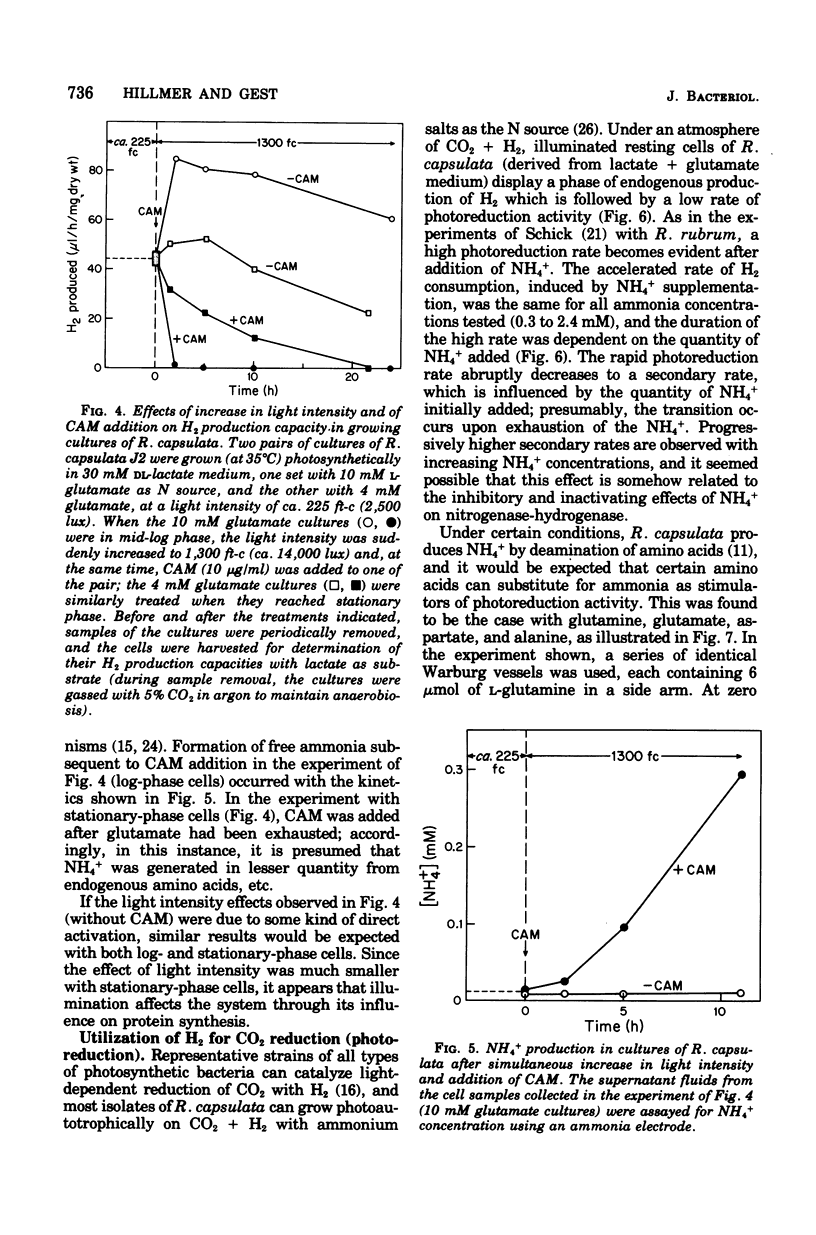
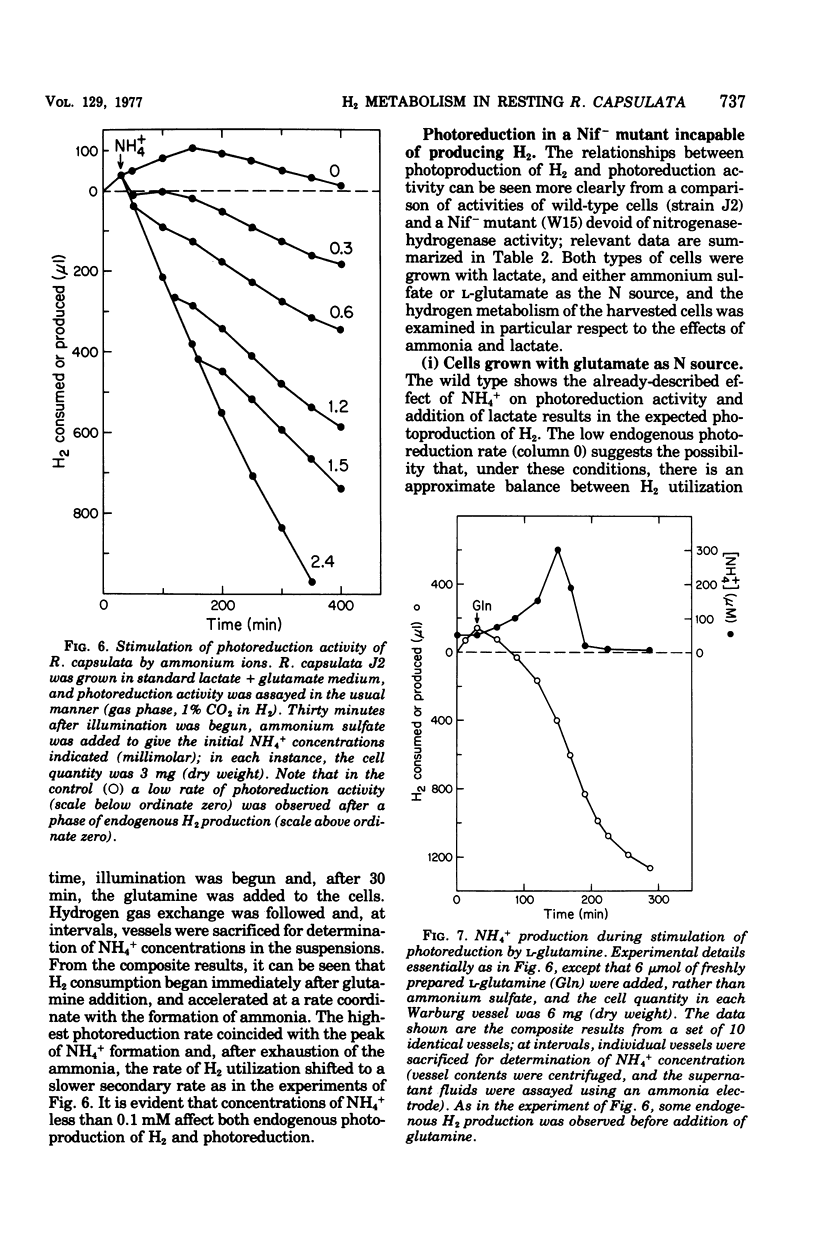
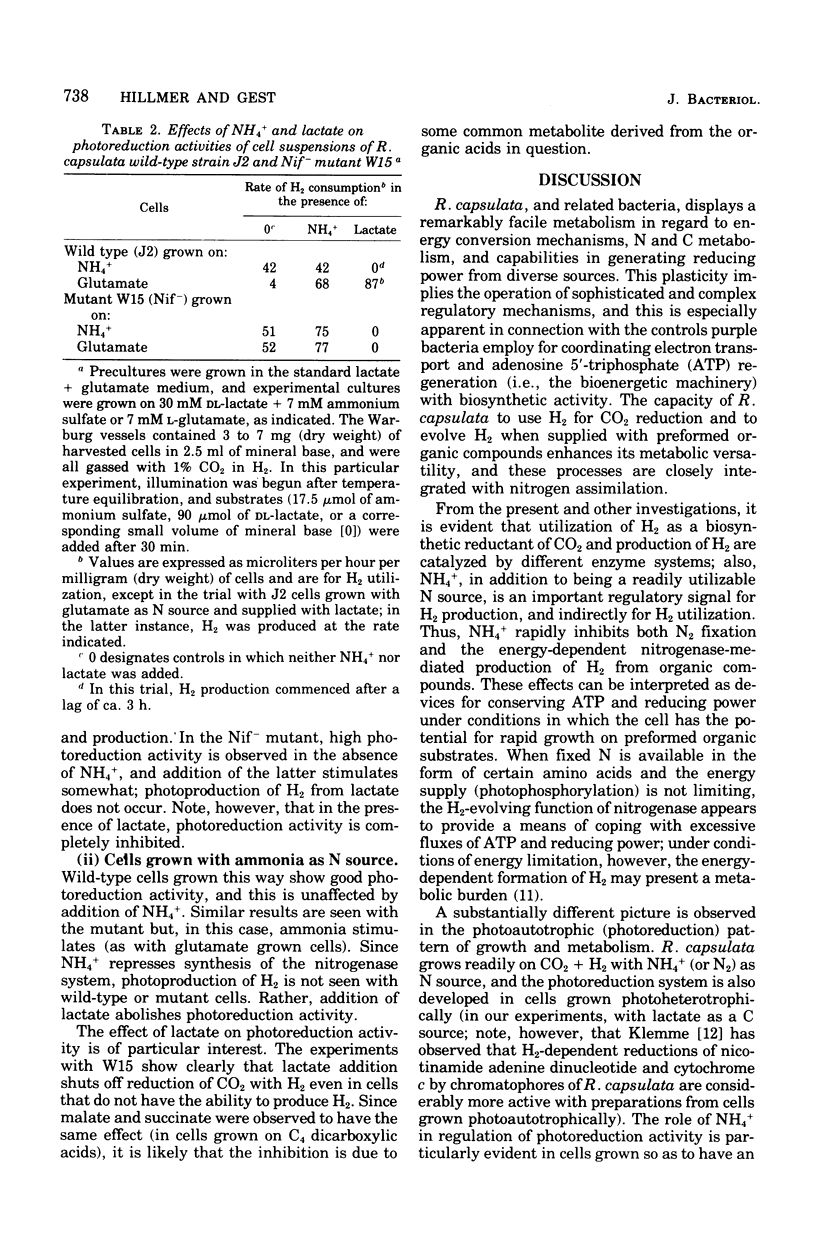
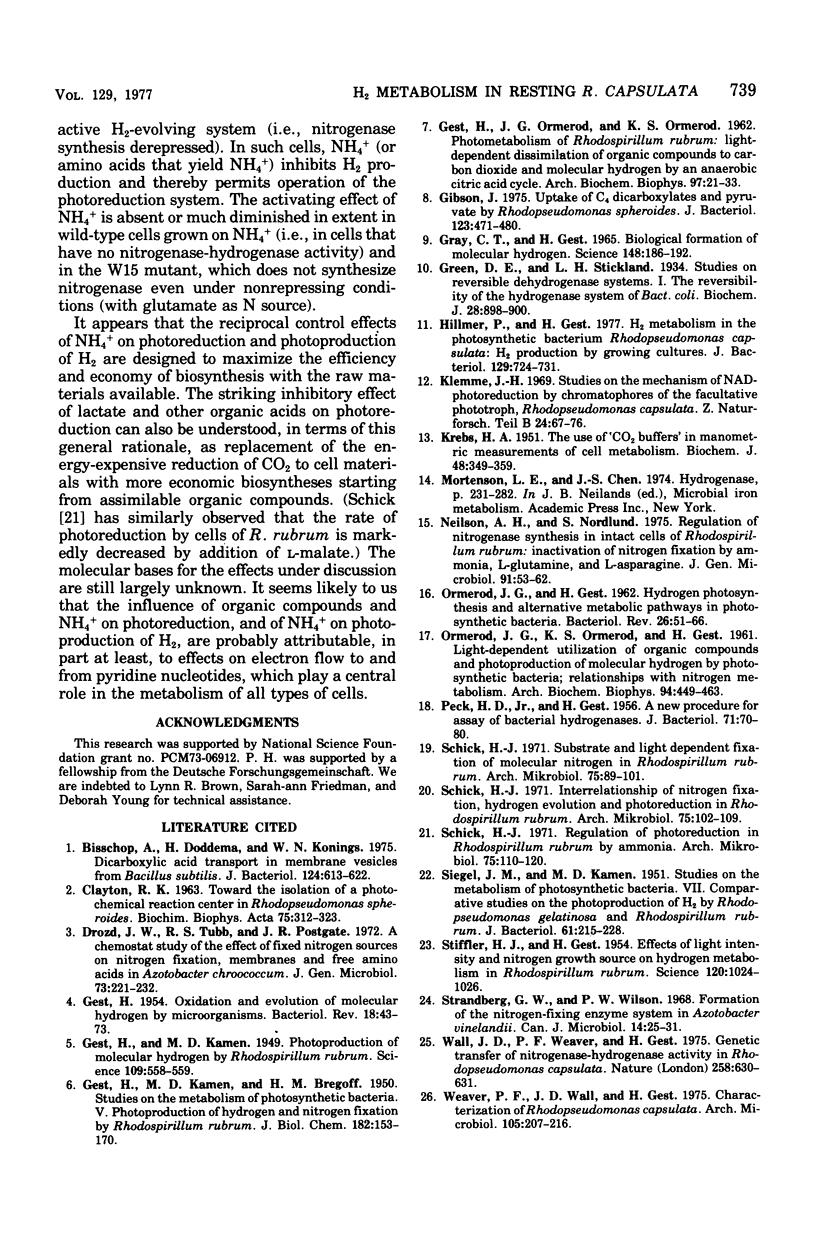
Selected References
These references are in PubMed. This may not be the complete list of references from this article.
- Bisschop A., Doddema H., Konings W. N. Dicarboxylic acid transport in membrane vesicles from Bacillus subtilis. J Bacteriol. 1975 Nov;124(2):613–622. doi: 10.1128/jb.124.2.613-622.1975. [DOI] [PMC free article] [PubMed] [Google Scholar]
- CLAYTON R. K. TOWARD THE ISOLATION OF A PHOTOCHEMICAL REACTION CENTER IN RHODOPSEUDOMONAS SPHEROIDES. Biochim Biophys Acta. 1963 Nov 29;75:312–323. doi: 10.1016/0006-3002(63)90618-8. [DOI] [PubMed] [Google Scholar]
- Drozd J. W., Tubb R. S., Postgate J. R. A chemostat study of the effect of fixed nitrogen sources on nitrogen fixation, membranes and free amino acids in Azotobacter chroococcum. J Gen Microbiol. 1972 Nov;73(2):221–232. doi: 10.1099/00221287-73-2-221. [DOI] [PubMed] [Google Scholar]
- GEST H., ORMEROD J. G., ORMEROD K. S. Photometabolism of Rhodospirillum rubrum: light-dependent dissimilation of organic compounds to carbon dioxide and molecular hydrogen by an anaerobic citric acid cycle. Arch Biochem Biophys. 1962 Apr;97:21–33. doi: 10.1016/0003-9861(62)90039-5. [DOI] [PubMed] [Google Scholar]
- GEST H. Oxidation and evolution of molecular hydrogen by microorganisms. Bacteriol Rev. 1954 Mar;18(1):43–73. doi: 10.1128/br.18.1.43-73.1954. [DOI] [PMC free article] [PubMed] [Google Scholar]
- GRAY C. T., GEST H. BIOLOGICAL FORMATION OF MOLECULAR HYDROGEN. Science. 1965 Apr 9;148(3667):186–192. doi: 10.1126/science.148.3667.186. [DOI] [PubMed] [Google Scholar]
- Gest H., Kamen M. D. Photoproduction of Molecular Hydrogen by Rhodospirillum rubrum. Science. 1949 Jun 3;109(2840):558–559. doi: 10.1126/science.109.2840.558. [DOI] [PubMed] [Google Scholar]
- Gibson J. Uptake of C4 dicarboxylates and pyruvate by Rhodopseudomonas spheroides. J Bacteriol. 1975 Aug;123(2):471–480. doi: 10.1128/jb.123.2.471-480.1975. [DOI] [PMC free article] [PubMed] [Google Scholar]
- Green D. E., Stickland L. H. Studies on reversible dehydrogenase systems: The reversibility of the hydrogenase system of Bact. coli. Biochem J. 1934;28(3):898–900. doi: 10.1042/bj0280898. [DOI] [PMC free article] [PubMed] [Google Scholar]
- Hillmer P., Gest H. H2 metabolism in the photosynthetic bacterium Rhodopseudomonas capsulata: H2 production by growing cultures. J Bacteriol. 1977 Feb;129(2):724–731. doi: 10.1128/jb.129.2.724-731.1977. [DOI] [PMC free article] [PubMed] [Google Scholar]
- KREBS H. A. The use of 'CO2 buffers' in manometric measurements of cell metabolism. Biochem J. 1951 Mar;48(3):349–359. doi: 10.1042/bj0480349. [DOI] [PMC free article] [PubMed] [Google Scholar]
- Klemme J. H. Studies on the mechanism of NAD-photoreduction by chromatophores of the facultative phototroph, Rhodopseudomonas capsulata. Z Naturforsch B. 1969 Jan;24(1):67–76. doi: 10.1515/znb-1969-0117. [DOI] [PubMed] [Google Scholar]
- Neilson A. H., Nordlund S. Regulation of nitrogenase synthesis in intact cells of Rhodospirillum rubrum: inactivation of nitrogen fixation by ammonia, L-glutamine and L-asparagine. J Gen Microbiol. 1975 Nov;91(1):53–62. doi: 10.1099/00221287-91-1-53. [DOI] [PubMed] [Google Scholar]
- ORMEROD J. G., GEST H. Symposium on metabolism of inorganic compounds. IV. Hydrogen photosynthesis and alternative metabolic pathways in photosynthetic bacteria. Bacteriol Rev. 1962 Mar;26:51–66. doi: 10.1128/br.26.1.51-66.1962. [DOI] [PMC free article] [PubMed] [Google Scholar]
- ORMEROD J. G., ORMEROD K. S., GEST H. Light-dependent utilization of organic compounds and photoproduction of molecular hydrogen by photosynthetic bacteria; relationships with nitrogen metabolism. Arch Biochem Biophys. 1961 Sep;94:449–463. doi: 10.1016/0003-9861(61)90073-x. [DOI] [PubMed] [Google Scholar]
- PECK H. D., Jr, GEST H. A new procedure for assay of bacterial hydrogenases. J Bacteriol. 1956 Jan;71(1):70–80. doi: 10.1128/jb.71.1.70-80.1956. [DOI] [PMC free article] [PubMed] [Google Scholar]
- SIEGEL J. M., KAMEN M. D. Studies on the metabolism of photosynthetic bacteria. VII. Comparative studies on the photoproduction of H2 by Rhodo-pseudomonas gelatinosa and Rhodo-spirillum rubrum. J Bacteriol. 1951 Feb;61(2):215–228. doi: 10.1128/jb.61.2.215-228.1951. [DOI] [PMC free article] [PubMed] [Google Scholar]
- STIFFLER H. J., GEST H. Effects of light intensity and nitrogen growth source on hydrogen metabolism in Rhodospirillum rubrum. Science. 1954 Dec 17;120(3129):1024–1026. doi: 10.1126/science.120.3129.1024. [DOI] [PubMed] [Google Scholar]
- Schick H. J. Interrelationship of nitrogen fixation, hydrogen evolution and photoreduction in Rhodospirillum rubrum. Arch Mikrobiol. 1971;75(2):102–109. doi: 10.1007/BF00407998. [DOI] [PubMed] [Google Scholar]
- Schick H. J. Regulation of photoreduction in Rhodospirillum rubrum by ammonia. Arch Mikrobiol. 1971;75(2):110–120. doi: 10.1007/BF00407999. [DOI] [PubMed] [Google Scholar]
- Schick H. J. Substrate and light dependent fixation of molecular nitrogen in Rhodospirillum rubrum. Arch Mikrobiol. 1971;75(2):89–101. doi: 10.1007/BF00407997. [DOI] [PubMed] [Google Scholar]
- Strandberg G. W., Wilson P. W. Formation of the nitrogen-fixing enzyme system in Azotobacter vinelandii. Can J Microbiol. 1968 Jan;14(1):25–31. doi: 10.1139/m68-005. [DOI] [PubMed] [Google Scholar]
- Wall J. D., Weaver P. F., Gest H. Genetic transfer of nitrogenase-hydrogenase activity in Rhodopseudomonas capsulata. Nature. 1975 Dec 18;258(5536):630–631. doi: 10.1038/258630a0. [DOI] [PubMed] [Google Scholar]
- Weaver P. F., Wall J. D., Gest H. Characterization of Rhodopseudomonas capsulata. Arch Microbiol. 1975 Nov 7;105(3):207–216. doi: 10.1007/BF00447139. [DOI] [PubMed] [Google Scholar]