Abstract
The expression of cell cycle events in Caulobacter crescentus CB13 has been shown to be associated with regulation of carbohydrate utilization. Growth on lactose and galactose depends on induction of specific enzymes. Prior growth on glucose results in a delay in enzyme expression and cell cycle arrest at the nonmotile, predivisional stage. Dibutyryl cyclic adenosine 3′,5′-monophosphate (AMP) was shown to stimulate expression of the inducible enzymes and, thus, the initiation of the cell cycle. β-Galactosidase-constitutive mutants did not exhibit a cell cycle arrest upon transfer of cultures from glucose to lactose. Furthermore, carbon source starvation results in accumulation of the cells at the predivisional stage. The cell cycle arrest therefore results from nutritional deprivation and is analogous to the general control system exhibited by yeast (Hartwell, Bacteriol. Rev. 38:164-198, 1974; Wolfner et al., J. Mol. Biol. 96:273-290, 1975), which coordinates cell cycle initiation with metabolic state. Transfer of C. crescentus CB13 from glucose to mannose did not result in a cell cycle arrest, and it was demonstrated that this carbon source is metabolized by constitutive enzymes. Growth on mannose, however, is stimulated by exogenous dibutyryl cyclic AMP without a concomitant increase in the specific activity of the mannose catabolic enzymes. The effect of cyclic AMP on growth on sugars metabolized by inducible enzymes, as well as on sugars metabolized by constitutive enzymes, may represent a regulatory system common to both types of sugar utilization, since they share features that differ from glucose utilization, namely, temperature-sensitive growth and low intracellular concentrations of cyclic guanosine 3′,5′-monophosphate.
Full text
PDF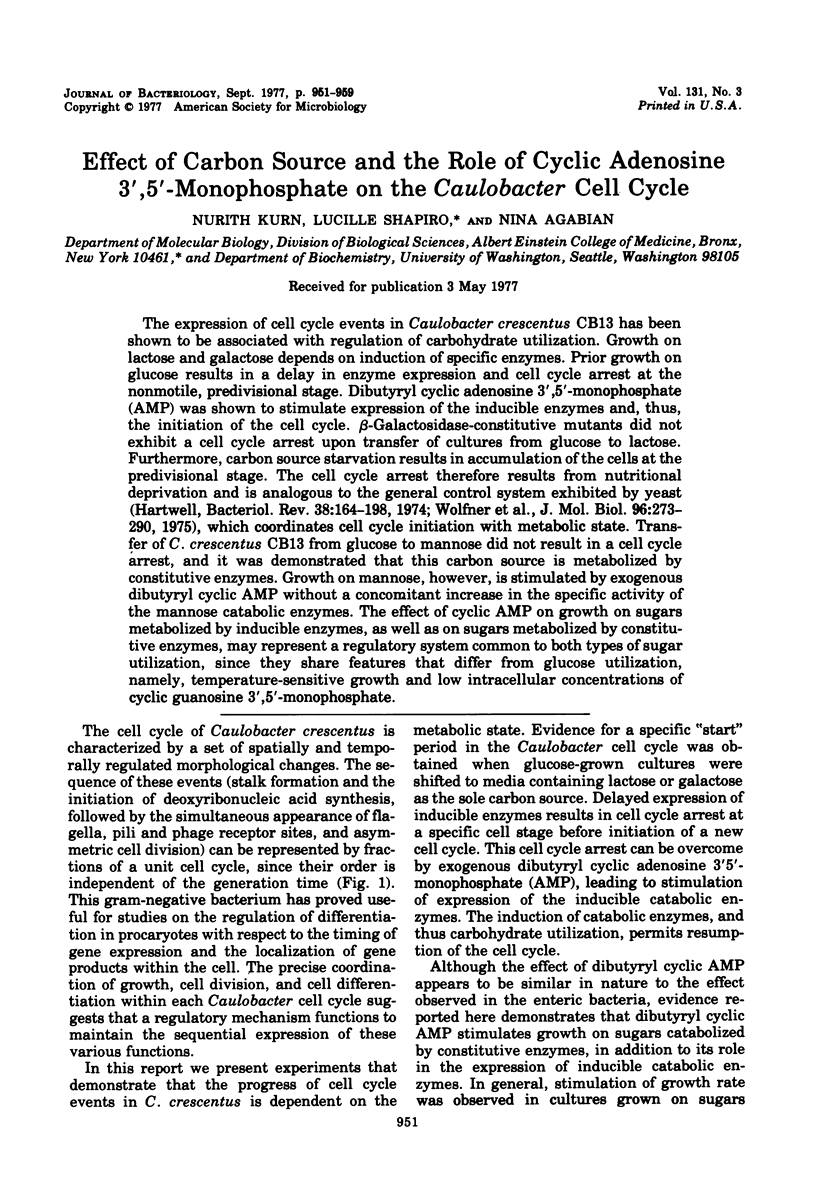
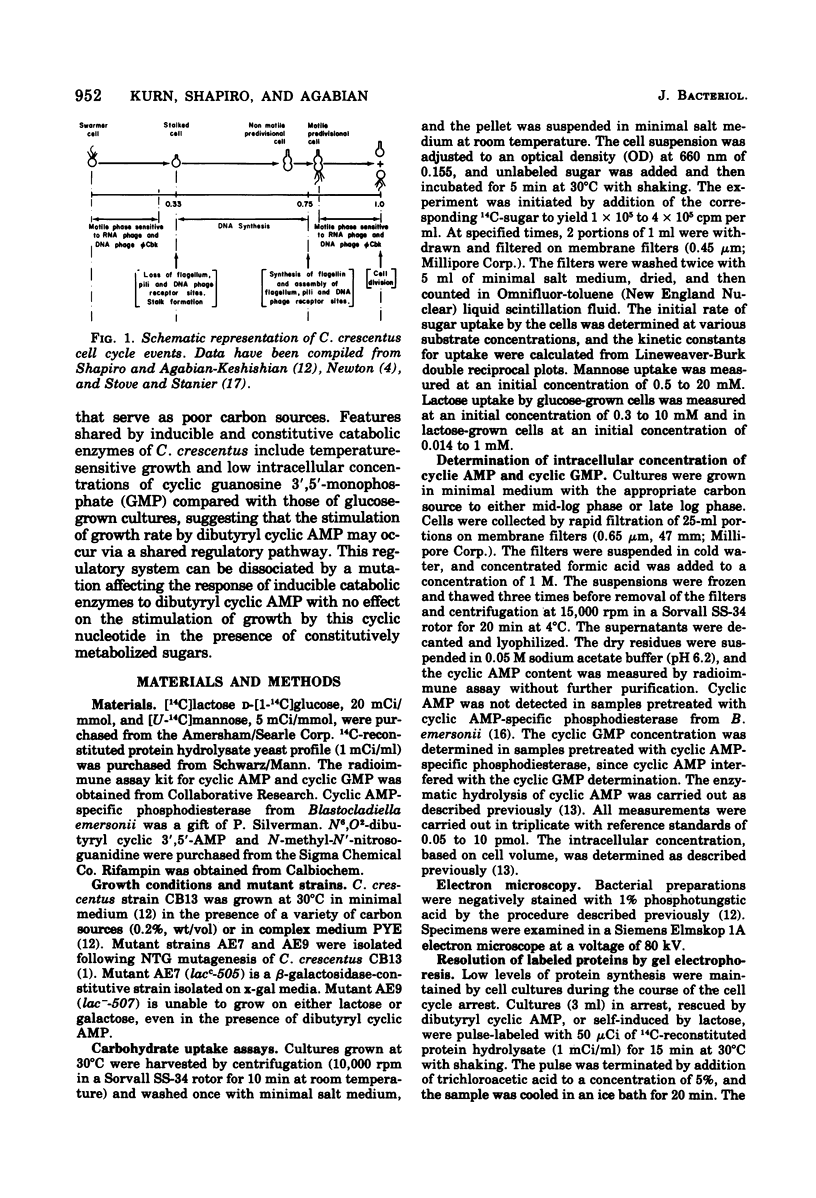
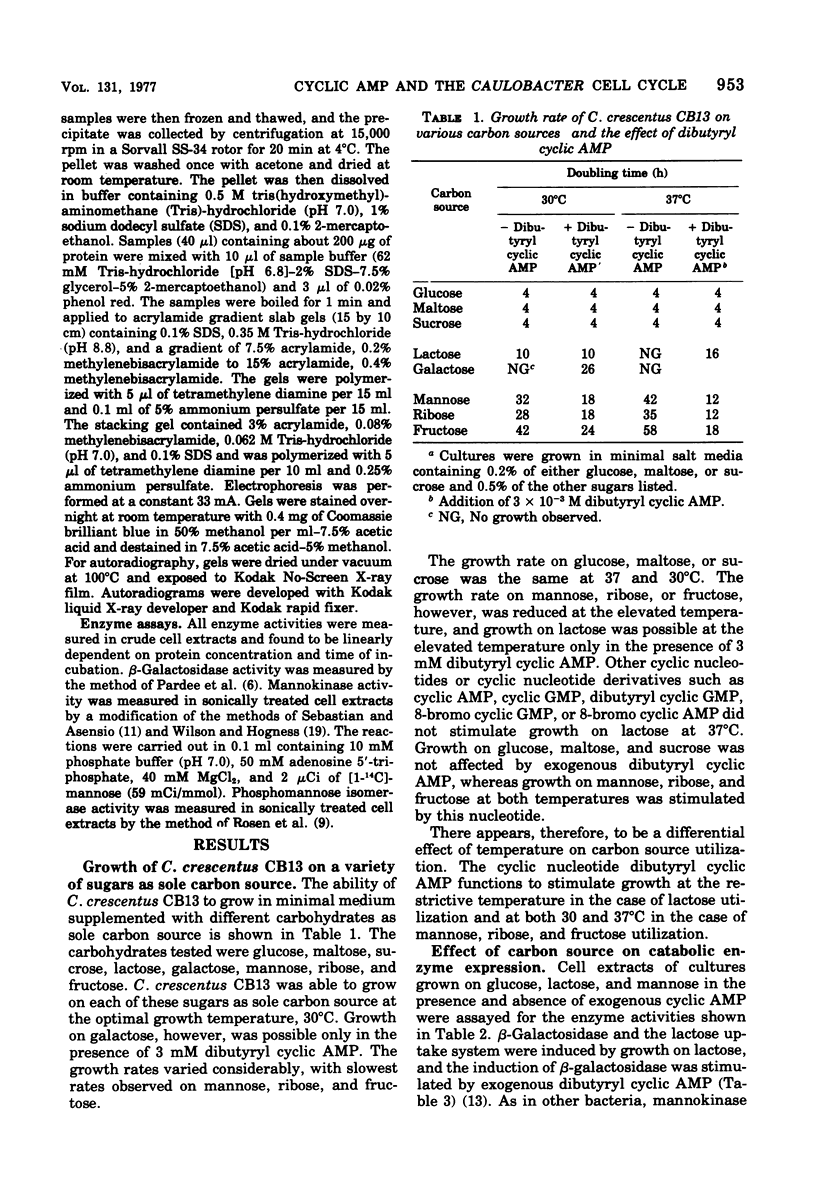
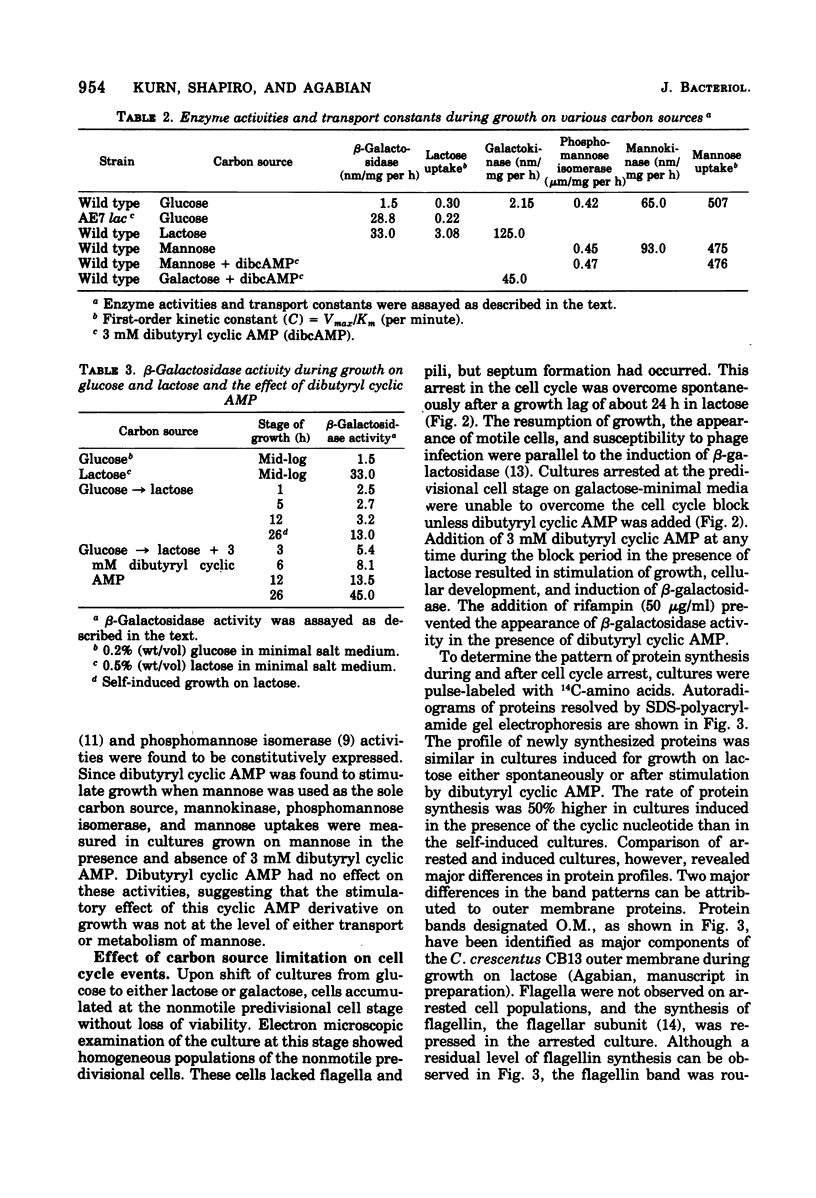
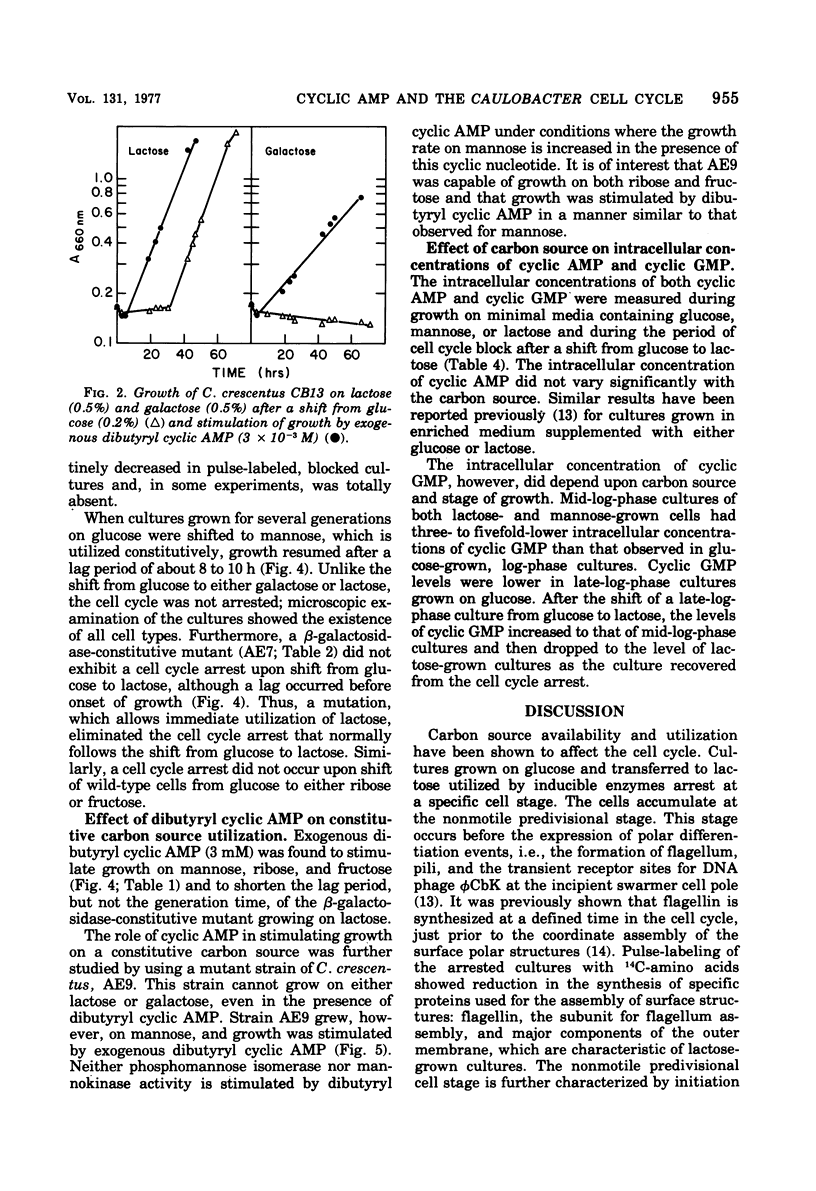
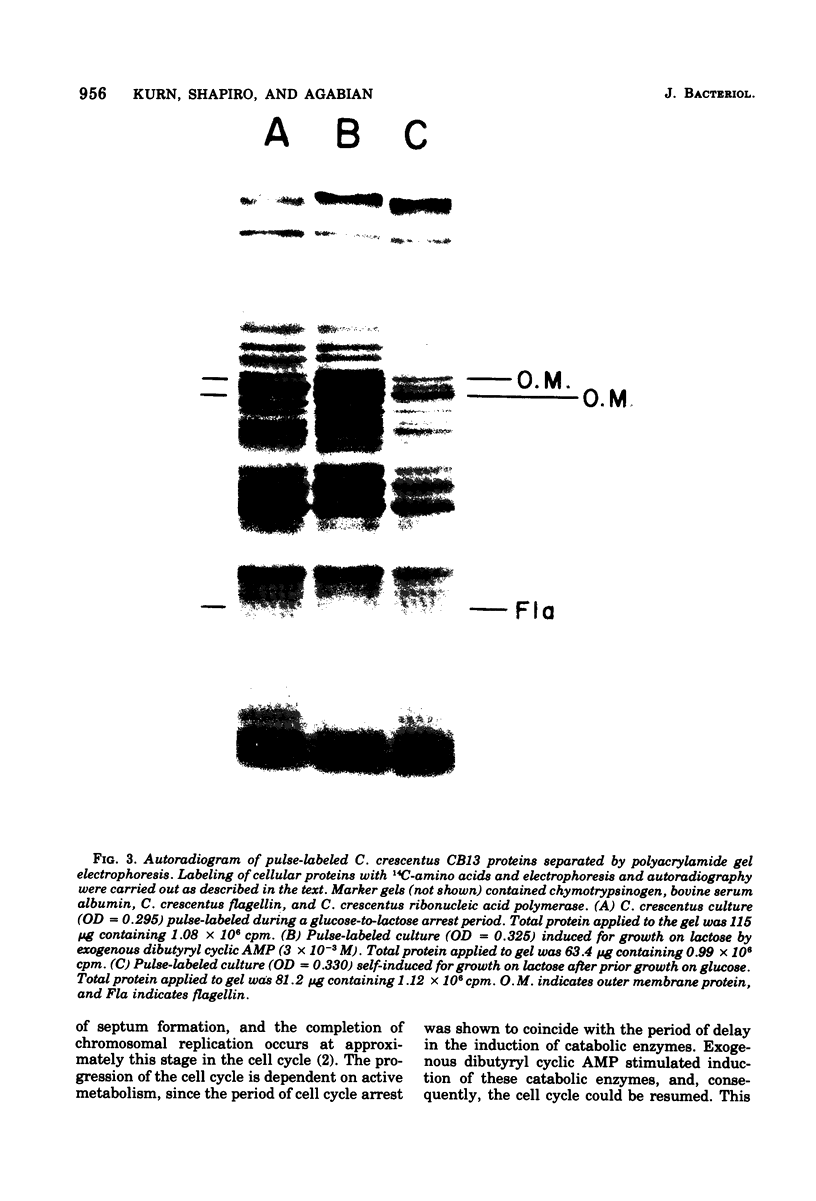
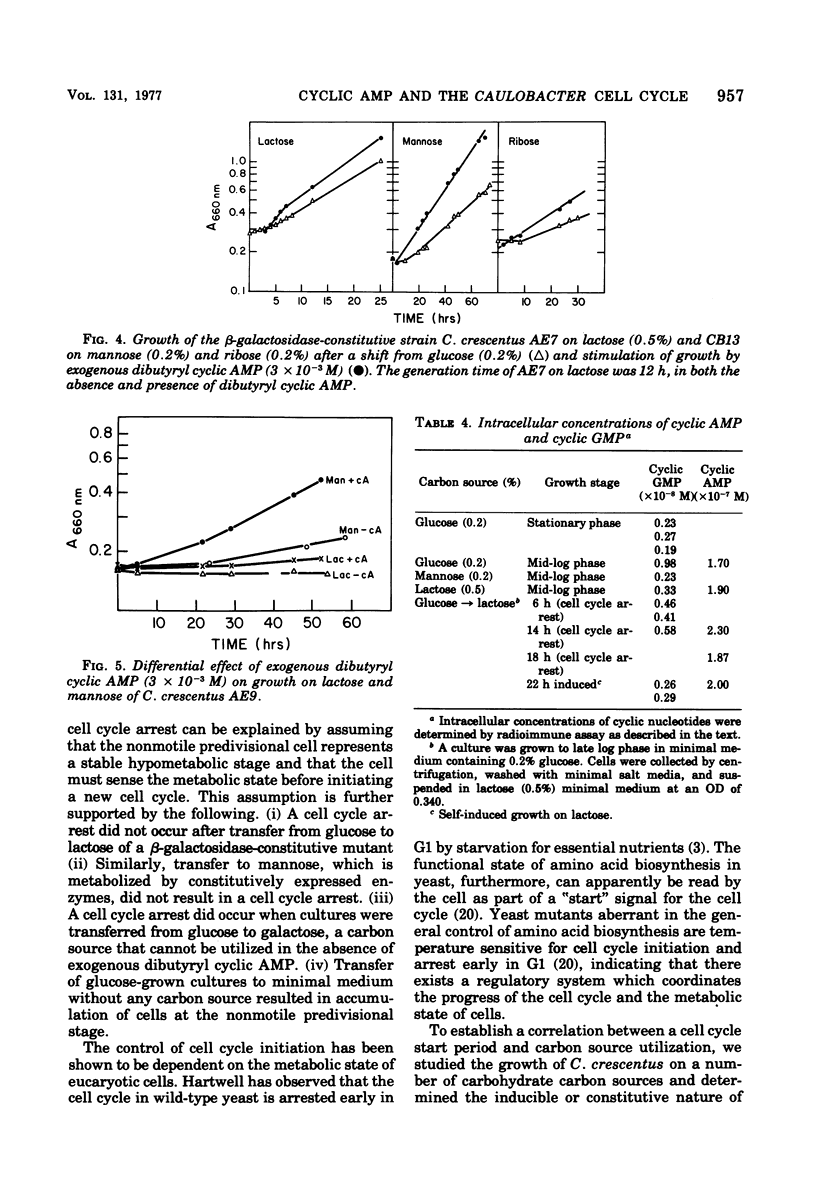
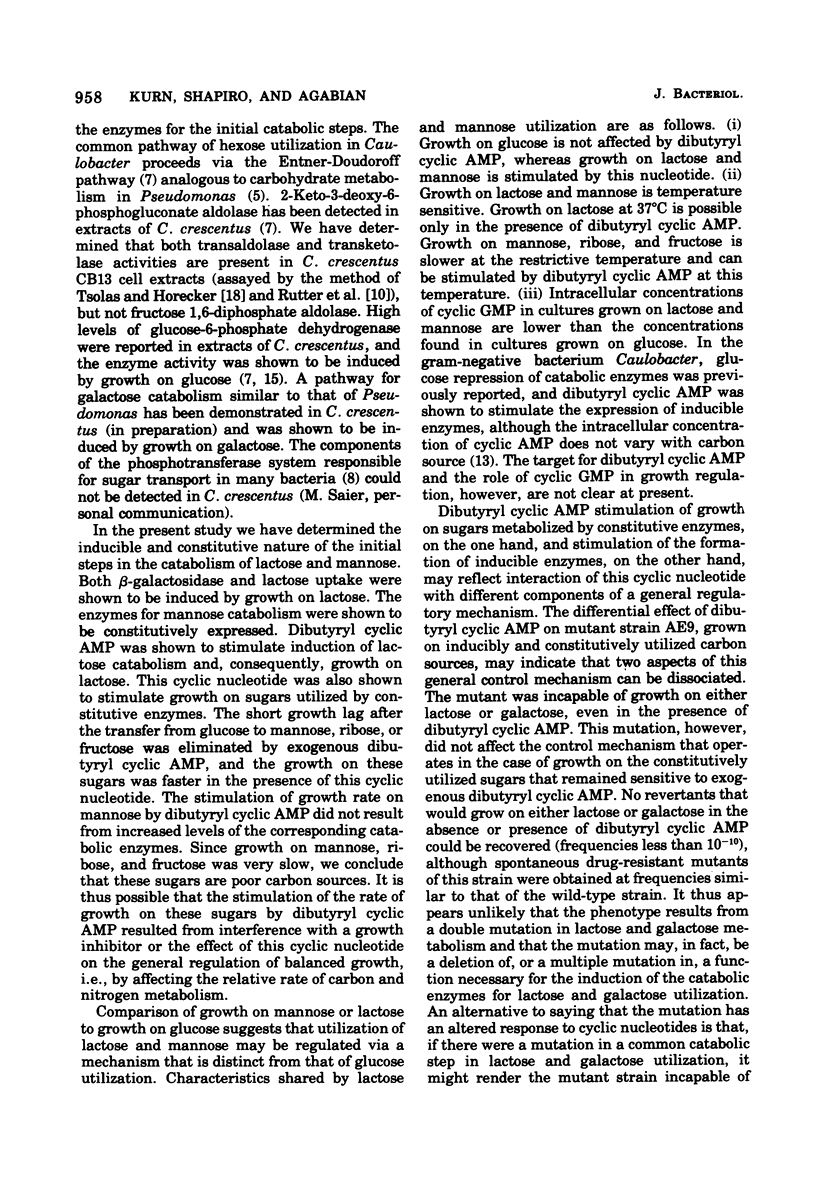
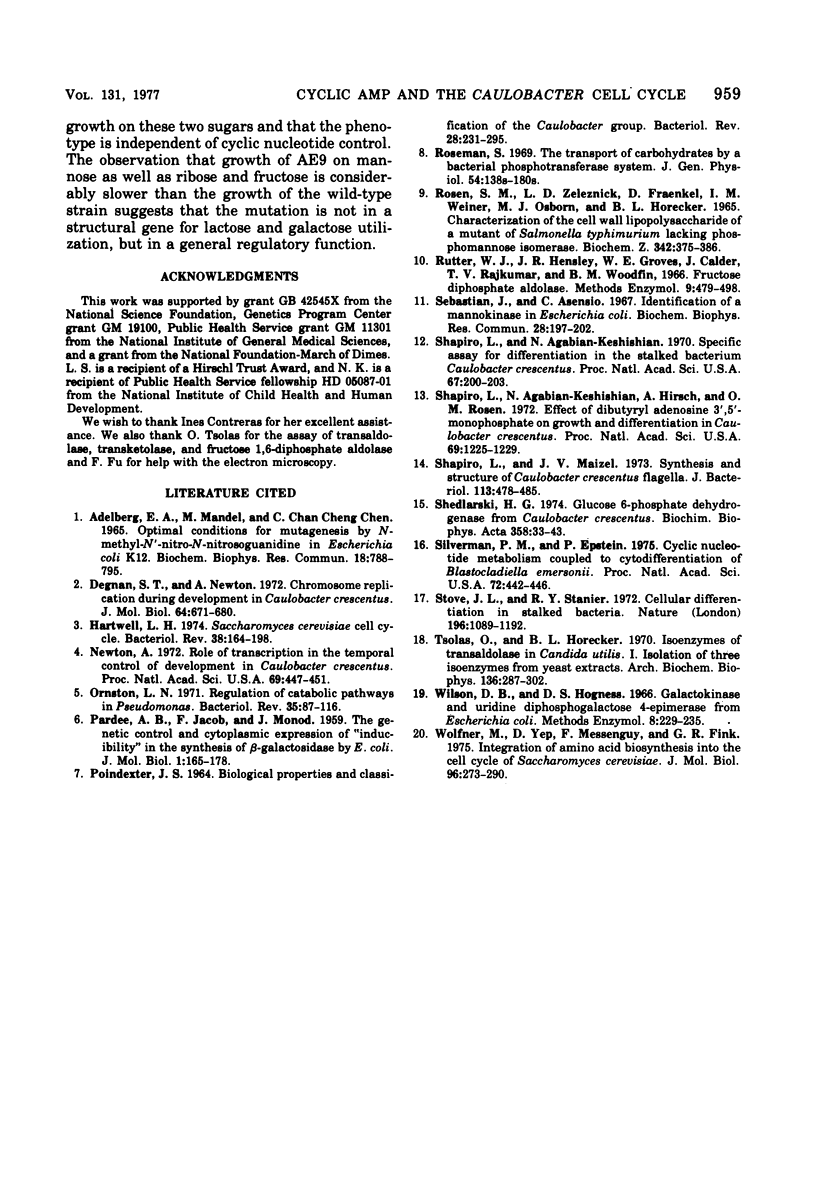
Images in this article
Selected References
These references are in PubMed. This may not be the complete list of references from this article.
- Degnen S. T., Newton A. Chromosome replication during development in Caulobacter crescentus. J Mol Biol. 1972 Mar 14;64(3):671–680. doi: 10.1016/0022-2836(72)90090-3. [DOI] [PubMed] [Google Scholar]
- Hartwell L. H. Saccharomyces cerevisiae cell cycle. Bacteriol Rev. 1974 Jun;38(2):164–198. doi: 10.1128/br.38.2.164-198.1974. [DOI] [PMC free article] [PubMed] [Google Scholar]
- Newton A. Role of transcription in the temporal control of development in Caulobacter crescentus (stalk-rifampin-RNA synthesis-DNA synthesis-motility). Proc Natl Acad Sci U S A. 1972 Feb;69(2):447–451. doi: 10.1073/pnas.69.2.447. [DOI] [PMC free article] [PubMed] [Google Scholar]
- Ornston L. N. Regulation of catabolic pathways in Pseudomonas. Bacteriol Rev. 1971 Jun;35(2):87–116. doi: 10.1128/br.35.2.87-116.1971. [DOI] [PMC free article] [PubMed] [Google Scholar]
- POINDEXTER J. S. BIOLOGICAL PROPERTIES AND CLASSIFICATION OF THE CAULOBACTER GROUP. Bacteriol Rev. 1964 Sep;28:231–295. doi: 10.1128/br.28.3.231-295.1964. [DOI] [PMC free article] [PubMed] [Google Scholar]
- Rosen S. M., Zeleznick L. D., Fraenkel D., Wiener I. M., Osborn M. J., Horecker B. L. Characterization of the cell wall lipopolysaccharide of a mutant of Salmonella typhimurium lacking phosphomannose isomerase. Biochem Z. 1965 Aug 19;342(4):375–386. [PubMed] [Google Scholar]
- Sebastián J., Asensio C. Identification of mannokinase in Escherichia coli. Biochem Biophys Res Commun. 1967 Jul 21;28(2):197–202. doi: 10.1016/0006-291x(67)90429-9. [DOI] [PubMed] [Google Scholar]
- Shapiro L., Agabian-Keshishian N., Hirsch A., Rosen O. M. Effect of dibutyryladenosine 3':5'-cyclic monophosphate on growth and differentiation in Caulobacter crescentus. Proc Natl Acad Sci U S A. 1972 May;69(5):1225–1229. doi: 10.1073/pnas.69.5.1225. [DOI] [PMC free article] [PubMed] [Google Scholar]
- Shapiro L., Agabian-Keshishian N. Specific Assay for Differentiation in the Stalked Bacterium Caulobacter crescentus. Proc Natl Acad Sci U S A. 1970 Sep;67(1):200–203. doi: 10.1073/pnas.67.1.200. [DOI] [PMC free article] [PubMed] [Google Scholar]
- Shapiro L., Maizel J. V., Jr Synthesis and structure of Caulobacter crescentus flagella. J Bacteriol. 1973 Jan;113(1):478–485. doi: 10.1128/jb.113.1.478-485.1973. [DOI] [PMC free article] [PubMed] [Google Scholar]
- Shedlarski J. G., Jr Glucose-6-phosphate dehydrogenase from Caulobacter crescentus. Biochim Biophys Acta. 1974 Jul 17;358(1):33–43. doi: 10.1016/0005-2744(74)90255-1. [DOI] [PubMed] [Google Scholar]
- Silverman P. M., Epstein P. M. Cyclic nucleotide metabolism coupled to cytodifferentiation of Blastocladiella emersonii. Proc Natl Acad Sci U S A. 1975 Feb;72(2):442–446. doi: 10.1073/pnas.72.2.442. [DOI] [PMC free article] [PubMed] [Google Scholar]
- Tsolas O., Horecker B. L. Isoenzymes of transaldolase in Candida utilis. I. Isolation of three isoenzymes from yeast extracts. Arch Biochem Biophys. 1970 Jan;136(1):287–302. doi: 10.1016/0003-9861(70)90353-x. [DOI] [PubMed] [Google Scholar]
- Wolfner M., Yep D., Messenguy F., Fink G. R. Integration of amino acid biosynthesis into the cell cycle of Saccharomyces cerevisiae. J Mol Biol. 1975 Aug 5;96(2):273–290. doi: 10.1016/0022-2836(75)90348-4. [DOI] [PubMed] [Google Scholar]