Abstract
In Escherichia coli K-12 the naturally occurring hexitols D-mannitol, D-glucitol, and galactitol are taken up and phosphorylated via three distinct transport systems by a mechanism called either group translocation or vectorial phosphorylation. For every system, a membrane-bound enzyme II-complex of the phosphoenolpyruvate-dependent phosphotransferase system has been found, each requiring phosphoenolpyruvate, enzyme I, and HPr or alternatively P-HPr as the phosphate donor. Cells with a constitutive synthesis of all hexitol transport systems but with low P-HPr levels have very low transport and phosphorylating activities in vivo, although 40 to 90% of the enzyme II-complex activities are detected in cell extracts of such mutants. No indications for additional hexitol transport systems, especially for systems able to transport and accumulate free hexitols as in Klebsiella aerogenes, have been found. Substrate Km, and Vmax of the three transport systems for several hexitols and hexitol analogues have been determined by growth rates, transport activities, and in vitro phosphorylating activities. Each system was found to take up several hexitols, but only one hexitol serves as the inducer. This inducer invariably is the substrate with the highest affinity. Since bacterial transport systems, as a general rule, seem to have a relatively broad substrate specificity, in contrast to a more restricted inducer specificity, we propose to name the system inducible by D-mannitol and coded by the gene mtlA the D-mannitol transport system, the system inducible by D-glucitol and coded by gutA the D-glucitol transport system, and the system inducible by galactitol and coded by gatA the galactitol transport system.
Full text
PDF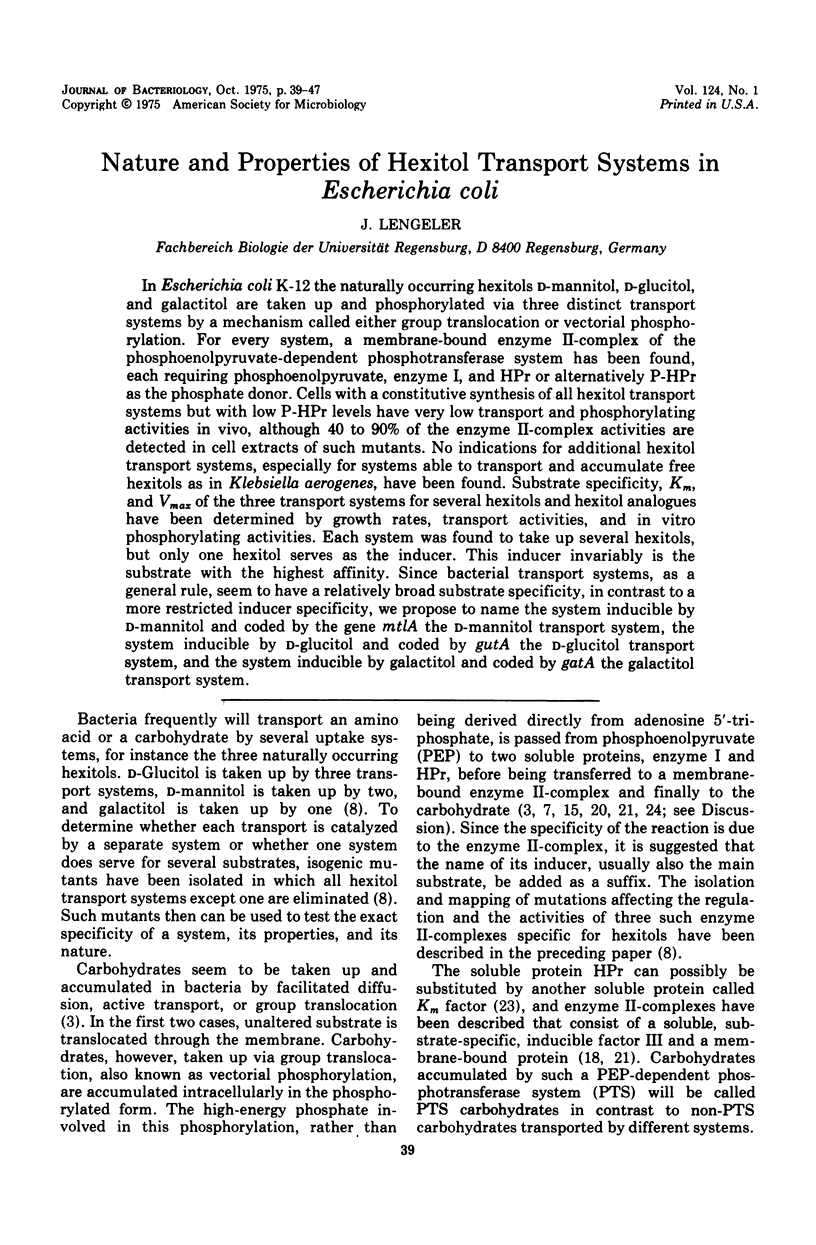
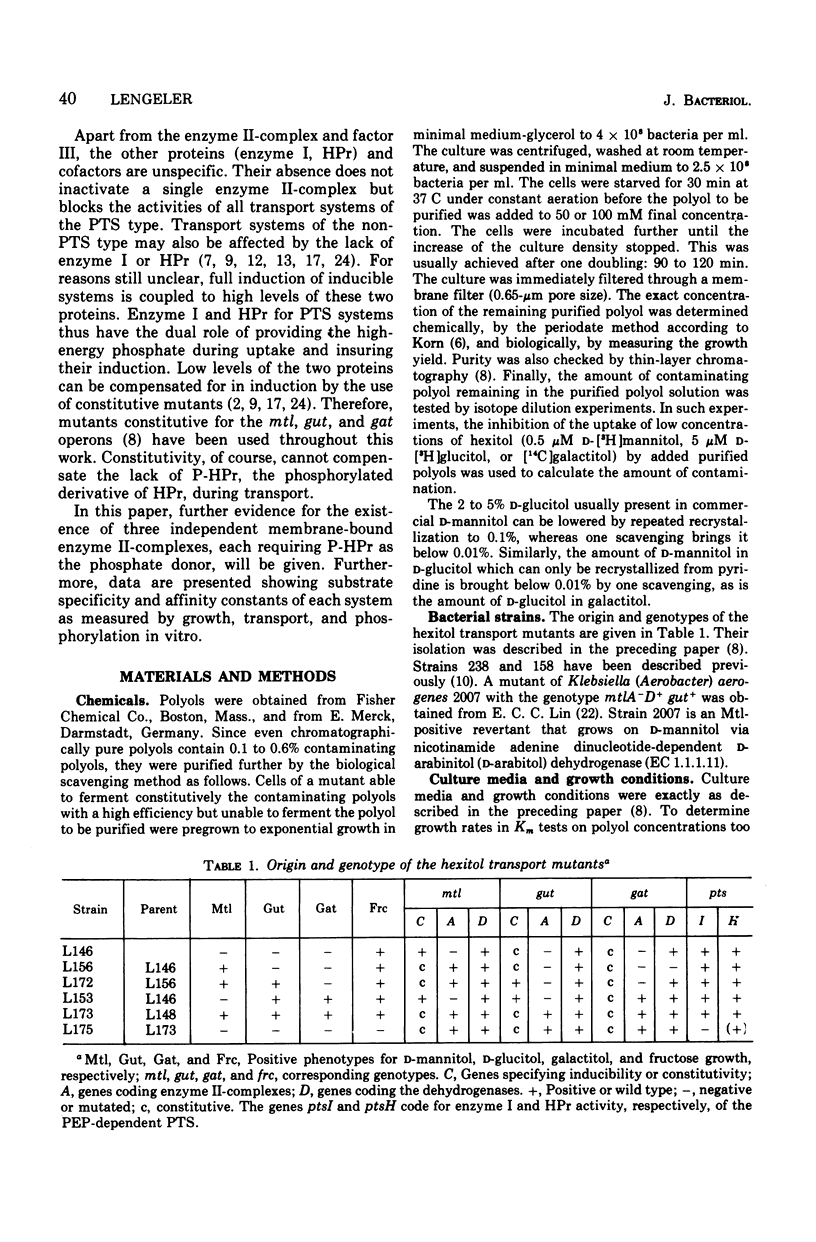
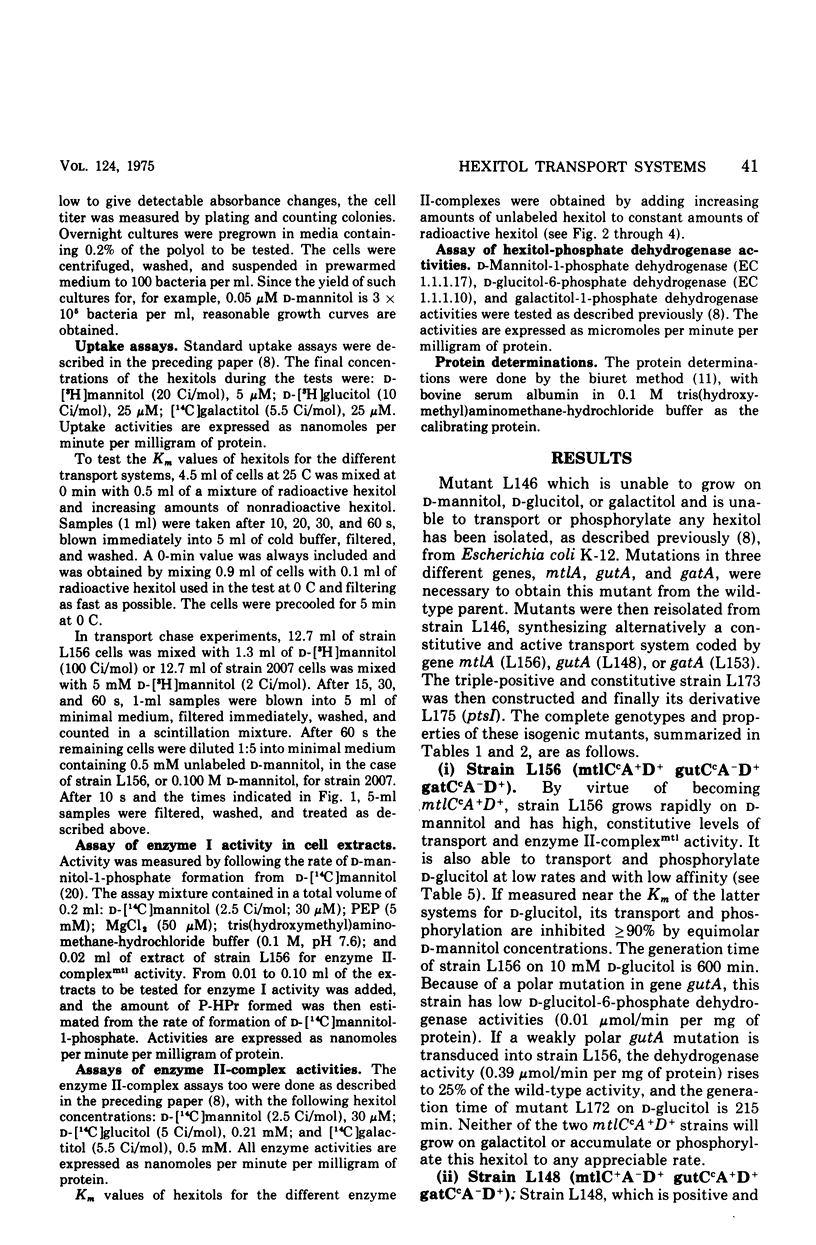
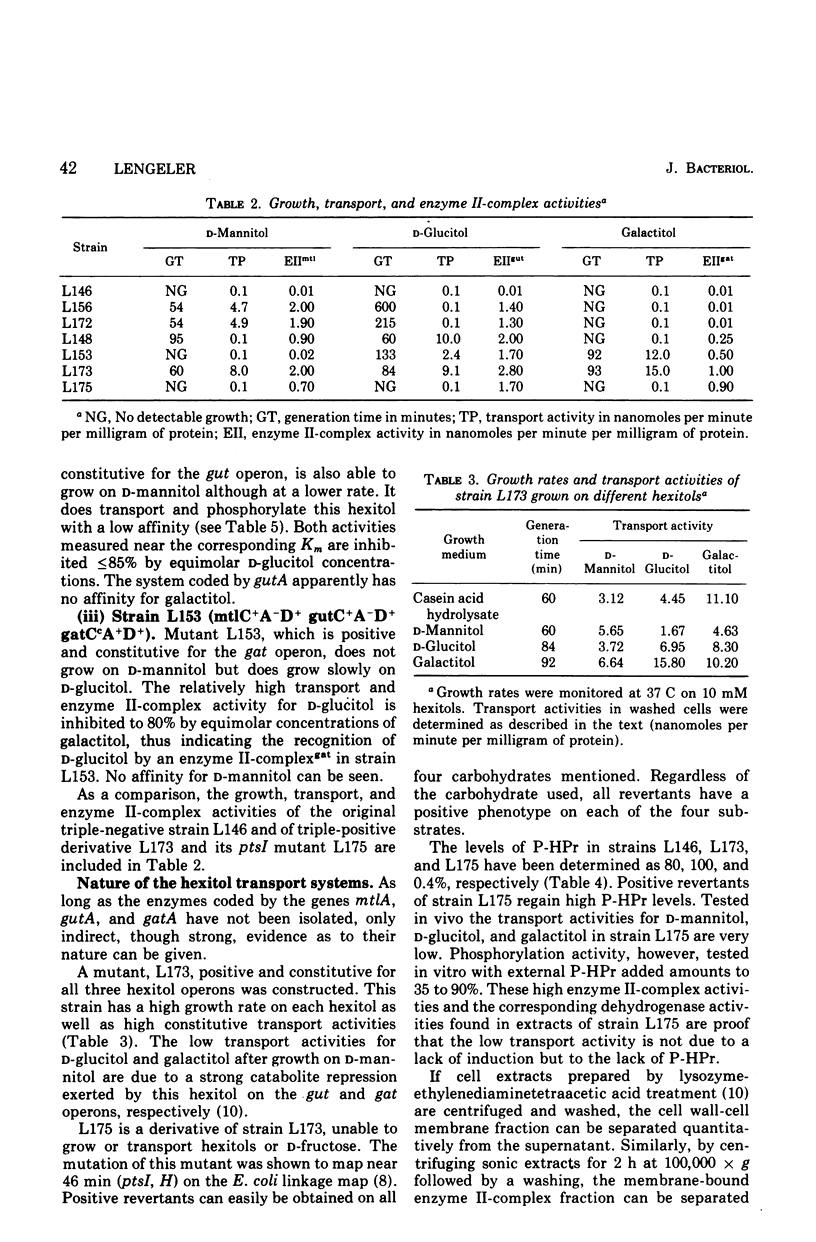
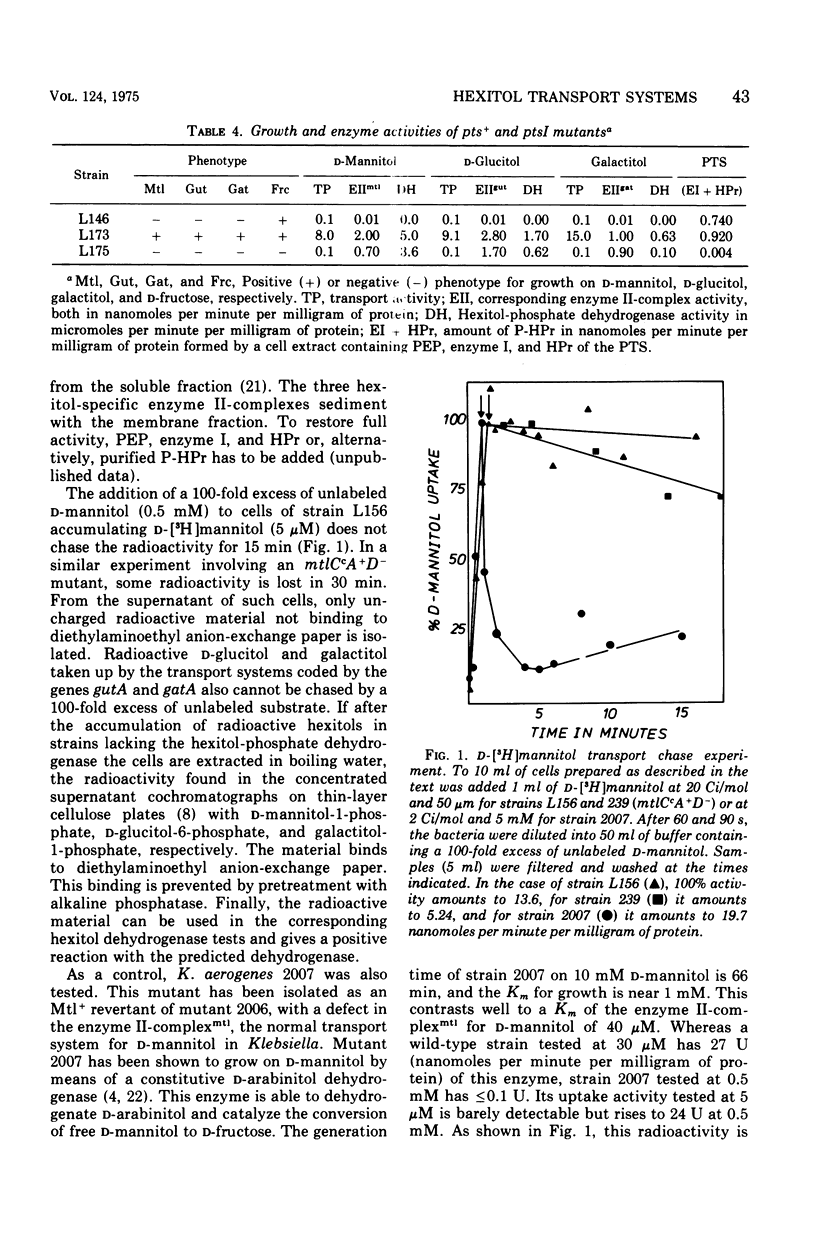
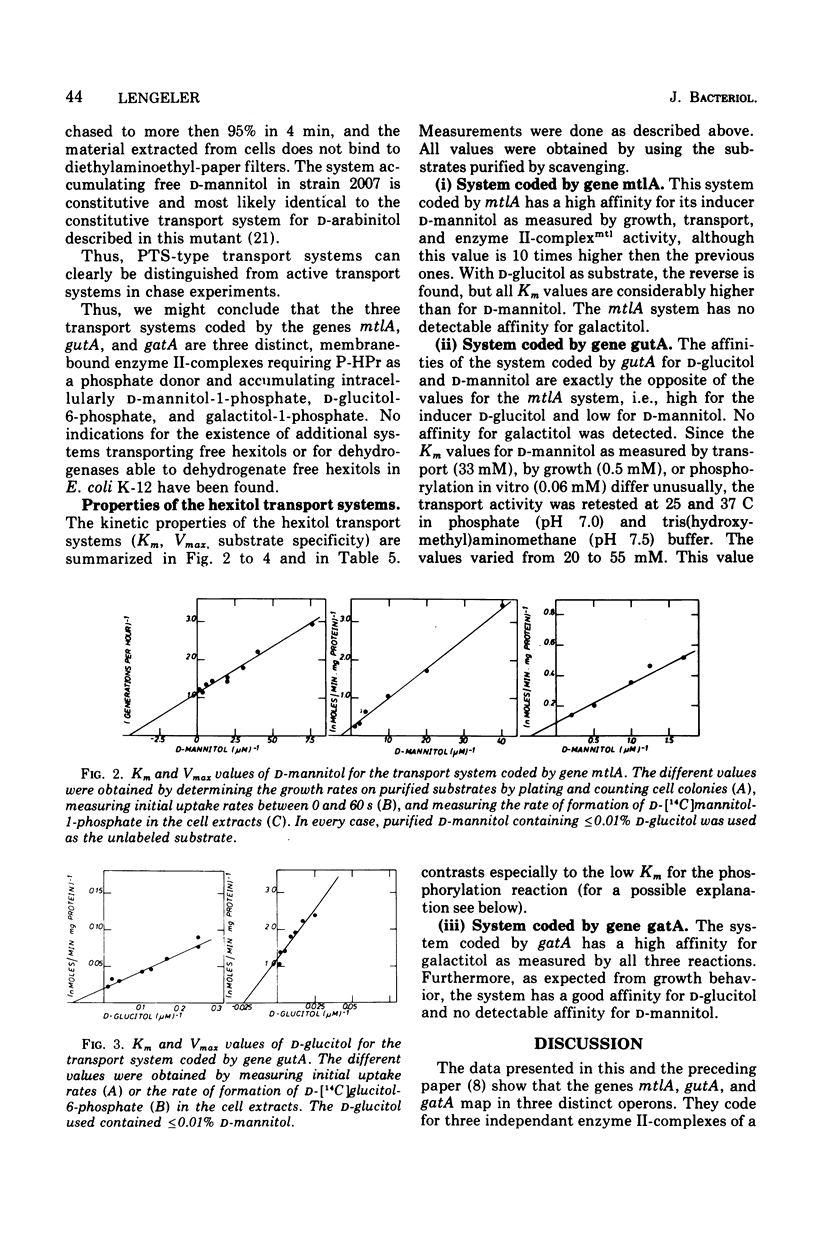
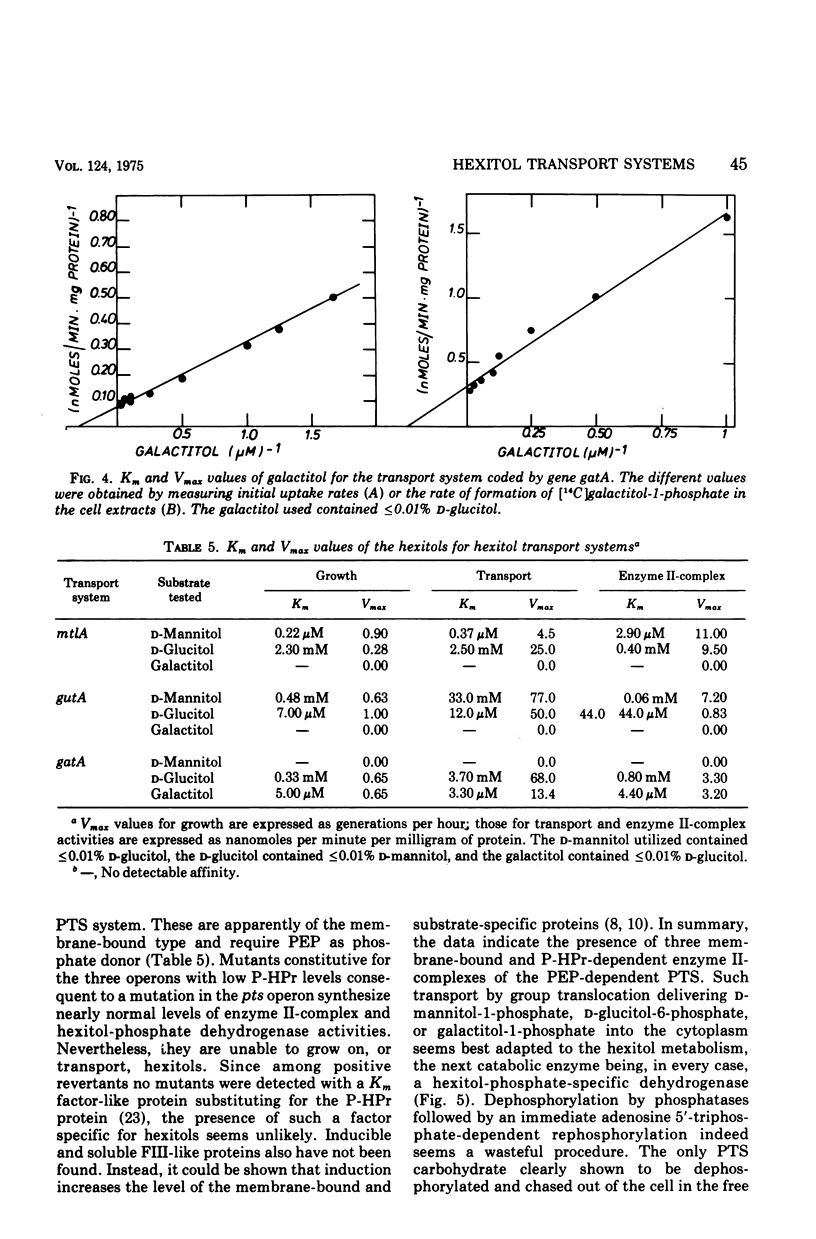
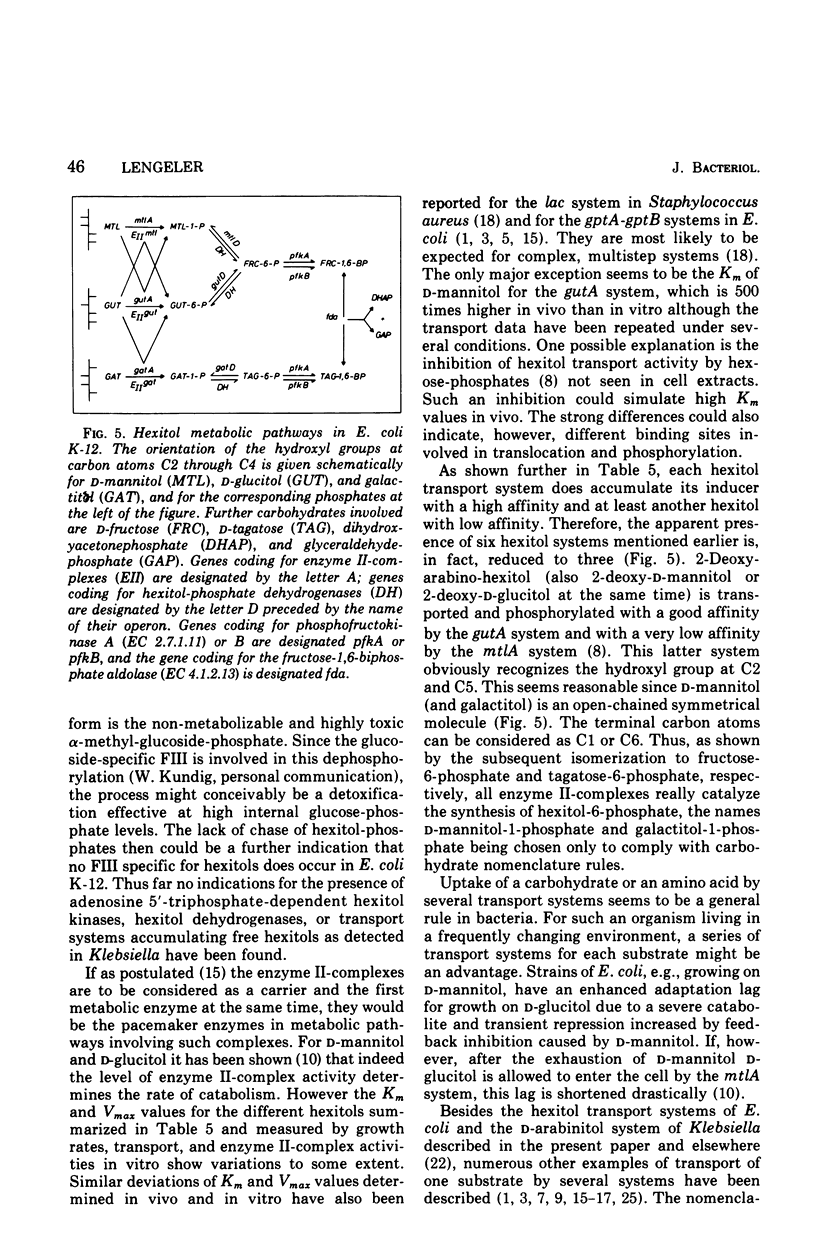
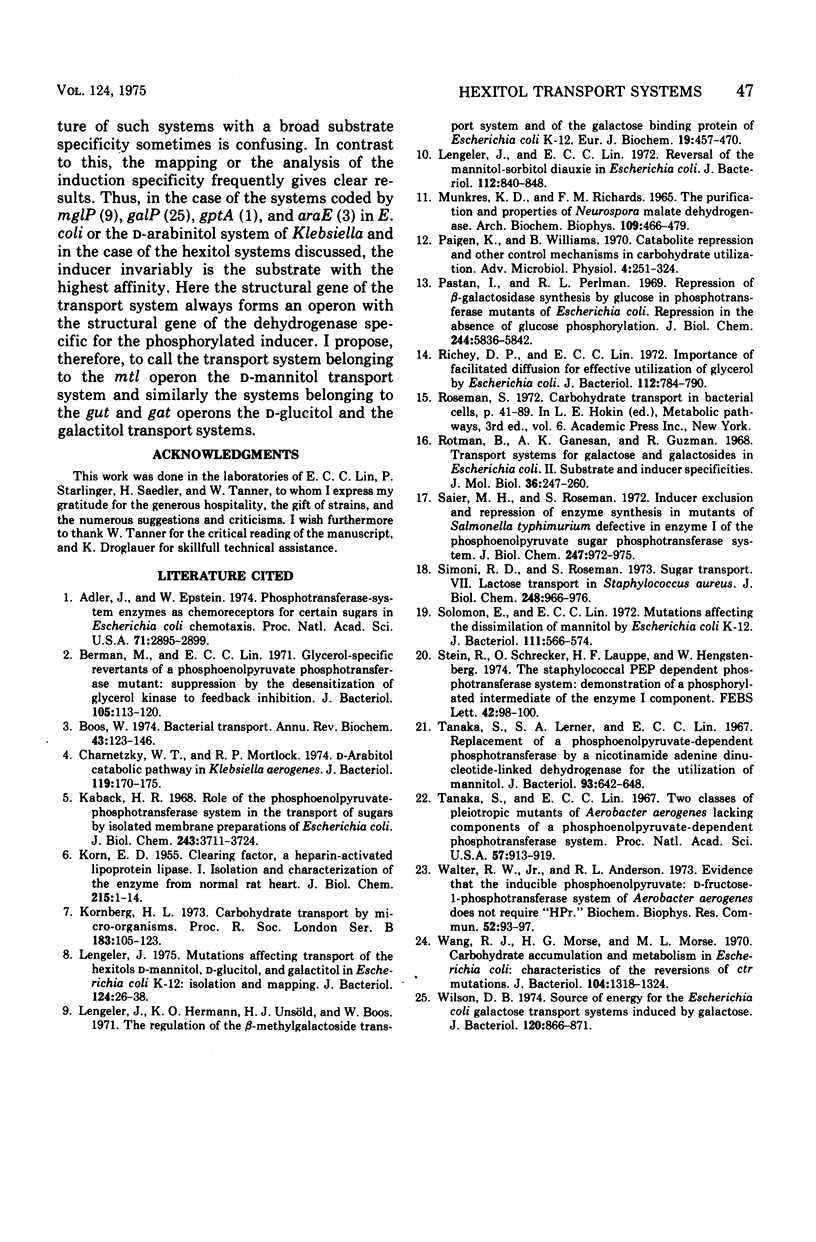
Selected References
These references are in PubMed. This may not be the complete list of references from this article.
- Adler J., Epstein W. Phosphotransferase-system enzymes as chemoreceptors for certain sugars in Escherichia coli chemotaxis. Proc Natl Acad Sci U S A. 1974 Jul;71(7):2895–2899. doi: 10.1073/pnas.71.7.2895. [DOI] [PMC free article] [PubMed] [Google Scholar]
- Berman M., Lin E. C. Glycerol-specific revertants of a phosphoenolpyruvate phosphotransferase mutant: suppression by the desensitization of glycerol kinase to feedback inhibition. J Bacteriol. 1971 Jan;105(1):113–120. doi: 10.1128/jb.105.1.113-120.1971. [DOI] [PMC free article] [PubMed] [Google Scholar]
- Boos W. Bacterial transport. Annu Rev Biochem. 1974;43(0):123–146. doi: 10.1146/annurev.bi.43.070174.001011. [DOI] [PubMed] [Google Scholar]
- Boos W., Lengeler J., Hermann K. O., Unsöld H. J. The regulation of the beta-methylgalactoside transport system and of the galactose binding protein of Escherichia coli K12. Eur J Biochem. 1971 Apr 30;19(4):457–470. doi: 10.1111/j.1432-1033.1971.tb01336.x. [DOI] [PubMed] [Google Scholar]
- Charnetzky W. T., Mortlock R. P. D-Arabitol catabolic pathway in Klebsiella aerogenes. J Bacteriol. 1974 Jul;119(1):170–175. doi: 10.1128/jb.119.1.170-175.1974. [DOI] [PMC free article] [PubMed] [Google Scholar]
- KORN E. D. Clearing factor, a heparin-activated lipoprotein lipase. I. Isolation and characterization of the enzyme from normal rat heart. J Biol Chem. 1955 Jul;215(1):1–14. [PubMed] [Google Scholar]
- Kaback H. R. The role of the phosphoenolpyruvate-phosphotransferase system in the transport of sugars by isolated membrane preparations of Escherichia coli. J Biol Chem. 1968 Jul 10;243(13):3711–3724. [PubMed] [Google Scholar]
- Kornberg H. L. Carbohydrate transport by micro-organisms. Proc R Soc Lond B Biol Sci. 1973 Mar 13;183(1071):105–123. doi: 10.1098/rspb.1973.0008. [DOI] [PubMed] [Google Scholar]
- Lengeler J., Lin E. C. Reversal of the mannitol-sorbitol diauxie in Escherichia coli. J Bacteriol. 1972 Nov;112(2):840–848. doi: 10.1128/jb.112.2.840-848.1972. [DOI] [PMC free article] [PubMed] [Google Scholar]
- Lengeler J. Mutations affecting transport of the hexitols D-mannitol, D-glucitol, and galactitol in Escherichia coli K-12: isolation and mapping. J Bacteriol. 1975 Oct;124(1):26–38. doi: 10.1128/jb.124.1.26-38.1975. [DOI] [PMC free article] [PubMed] [Google Scholar]
- MUNKRES K. D., RICHARDS F. M. THE PURIFICATION AND PROPERTIES OF NEUROSPORA MALATE DEHYDROGENASE. Arch Biochem Biophys. 1965 Mar;109:466–479. doi: 10.1016/0003-9861(65)90391-7. [DOI] [PubMed] [Google Scholar]
- Pastan I., Perlman R. L. Repression of beta-galactosidase synthesis by glucose in phosphotransferase mutants of Escherichia coli. Repression in the absence of glucose phosphorylation. J Biol Chem. 1969 Nov 10;244(21):5836–5842. [PubMed] [Google Scholar]
- Richey D. P., Lin E. C. Importance of facilitated diffusion for effective utilization of glycerol by Escherichia coli. J Bacteriol. 1972 Nov;112(2):784–790. doi: 10.1128/jb.112.2.784-790.1972. [DOI] [PMC free article] [PubMed] [Google Scholar]
- Rotman B., Ganesan A. K., Guzman R. Transport systems for galactose and galactosides in Escherichia coli. II. Substrate and inducer specificities. J Mol Biol. 1968 Sep 14;36(2):247–260. doi: 10.1016/0022-2836(68)90379-3. [DOI] [PubMed] [Google Scholar]
- Saier M. H., Roseman S. Inducer exclusion and repression of enzyme synthesis in mutants of Salmonella typhimurium defective in enzyme I of the phosphoenolpyruvate: sugar phosphotransferase system. J Biol Chem. 1972 Feb 10;247(3):972–975. [PubMed] [Google Scholar]
- Simoni R. D., Roseman S. Sugar transport. VII. Lactose transport in Staphylococcus aureus. J Biol Chem. 1973 Feb 10;248(3):966–974. [PubMed] [Google Scholar]
- Solomon E., Lin E. C. Mutations affecting the dissimilation of mannitol by Escherichia coli K-12. J Bacteriol. 1972 Aug;111(2):566–574. doi: 10.1128/jb.111.2.566-574.1972. [DOI] [PMC free article] [PubMed] [Google Scholar]
- Stein R., Schrecker O., Lauppe H. F., Hengstenberg H. The staphylococcal PEP dependent phosphotransferase system: demonstration of a phosphorylated intermediate of the enzyme I component. FEBS Lett. 1974 May 15;42(1):98–100. doi: 10.1016/0014-5793(74)80288-7. [DOI] [PubMed] [Google Scholar]
- Tanaka S., Lerner S. A., Lin E. C. Replacement of a phosphoenolpyruvate-dependent phosphotransferase by a nicotinamide adenine dinucleotide-linked dehydrogenase for the utilization of mannitol. J Bacteriol. 1967 Feb;93(2):642–648. doi: 10.1128/jb.93.2.642-648.1967. [DOI] [PMC free article] [PubMed] [Google Scholar]
- Tanaka S., Lin E. C. Two classes of pleiotropic mutants of Aerobacter aerogenes lacking components of a phosphoenolpyruvate-dependent phosphotransferase system. Proc Natl Acad Sci U S A. 1967 Apr;57(4):913–919. doi: 10.1073/pnas.57.4.913. [DOI] [PMC free article] [PubMed] [Google Scholar]
- Walter R. W., Jr, Anderson R. L. Evidence that the inducible phosphoenolpyruvate:D-fructose 1-phosphotransferase system of Aerobacter aerogenes does not require "HPr". Biochem Biophys Res Commun. 1973 May 1;52(1):93–97. doi: 10.1016/0006-291x(73)90958-3. [DOI] [PubMed] [Google Scholar]
- Wang R. J., Morse H. G., Morse M. L. Carbohydrate Accumulation and Metabolism in Escherichia coli: Characteristics of the Reversions of ctr Mutations. J Bacteriol. 1970 Dec;104(3):1318–1324. doi: 10.1128/jb.104.3.1318-1324.1970. [DOI] [PMC free article] [PubMed] [Google Scholar]
- Wilson D. B. Source of energy for the Escherichia coli galactose transport systems induced by galactose. J Bacteriol. 1974 Nov;120(2):866–871. doi: 10.1128/jb.120.2.866-871.1974. [DOI] [PMC free article] [PubMed] [Google Scholar]