Abstract
Membrane vesicles isolated from Bacillus subtilis W23 catalyze active transport of the C4 dicarboxylic acids L-malate, fumarate, and succinate under aerobic conditions in the presence of the electron donor reduced beta-nicotinamide adenine dinucleotide or the non-physiological electron donor system ascorbate-phenazine methosulfate. The dicarboxylic acids are accumulated in unmodified form. Inhibitors of the respiratory chain, sulfhydryl reagents, and uncoupling agents inhibit the accumulation of the dicarboxylic acids. The affinity constants for transport of L-malate, fumarate, and succinate are 13.5, 7.5, and 4.3 muM, respectively; these values are severalfold lower than those reported previously for whole cells. Active transport of these dicarboxylic acids occurs via one highly specific transport system as is indicated by the following observations. (i) Each dicarboxylic acid inhibits the transport of the other two dicarboxylic acids competitively. (ii) The affinity constants determined for the inhibitory action are very similar to those determined for the transport process. (iii) Each dicarboxylic acid exchanges rapidly with a previously accumulated dicarboxylic acid. (iv) Other metabolically and structurally related compounds do not inhibit transport of these dicarboxylic acids significantly, except for L-aspartate and L-glutamate. However, transport of these dicarboxylic amino acids is mediated by independent system because membrane vesicles from B. subtilis 60346, lacking functional dicarboxylic amino acid transport activity, accumulate the C4 dicarboxylic acids at even higher rates than vesicles from B. subtilis W 23. (v) A constant ratio exists between the initial rates of transport of L-malate, fumarate, and succinate in all membrane vesicle preparations isolated from cells grown on various media. This high-affinity dicarboxylic acid transport system seems to be present constitutively in B. subtilis W23.
Full text
PDF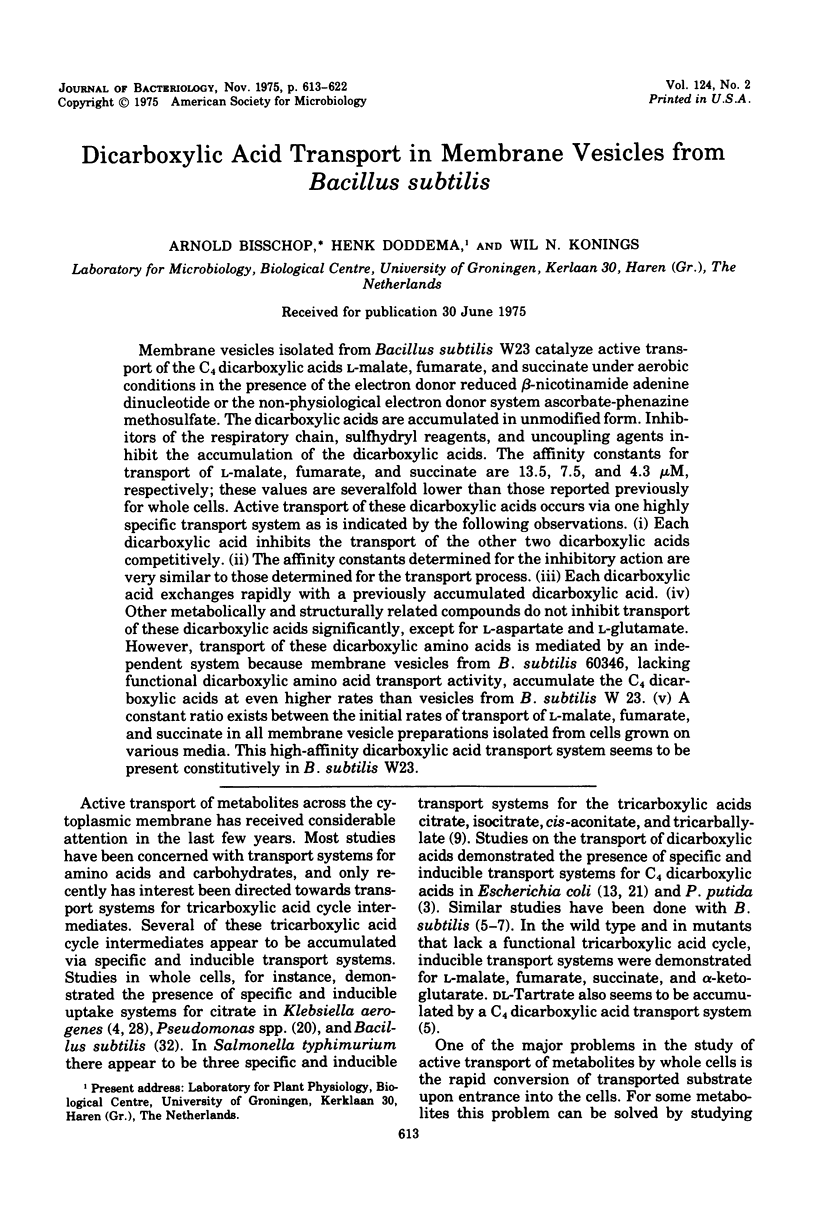
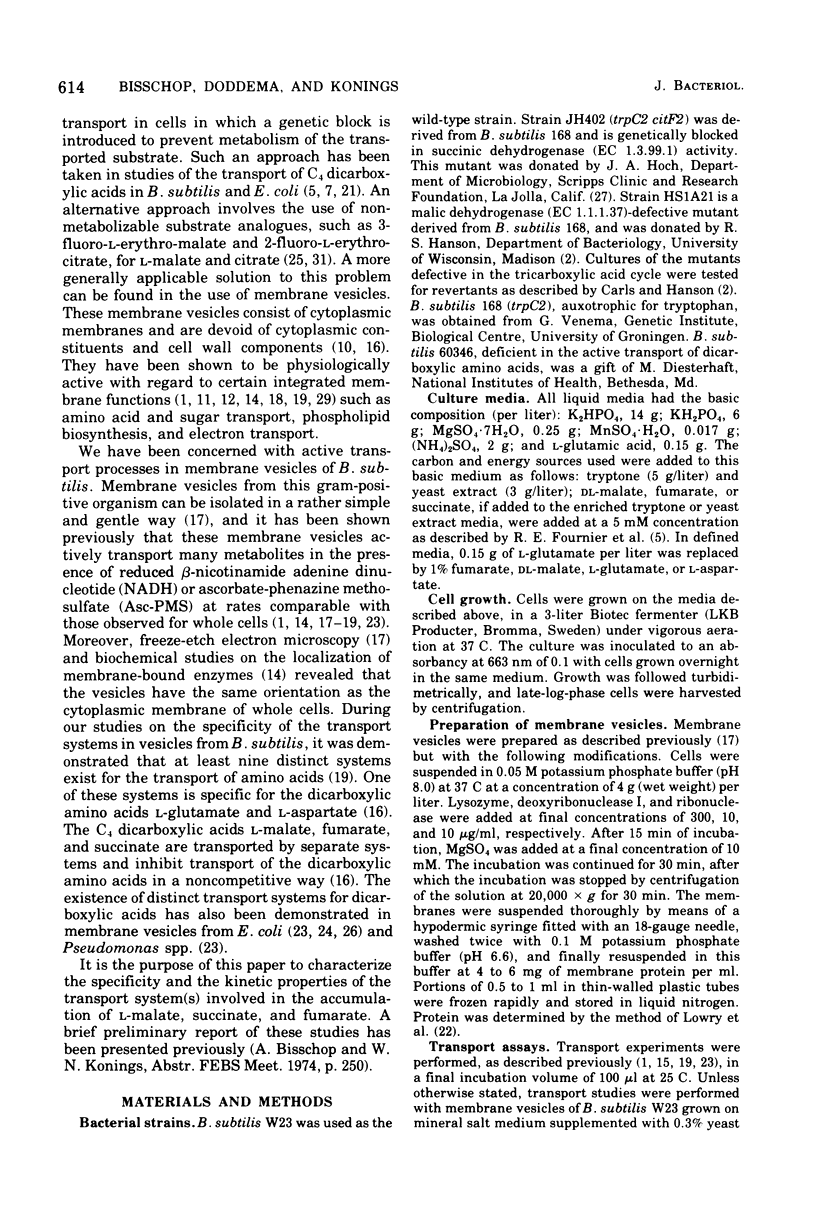
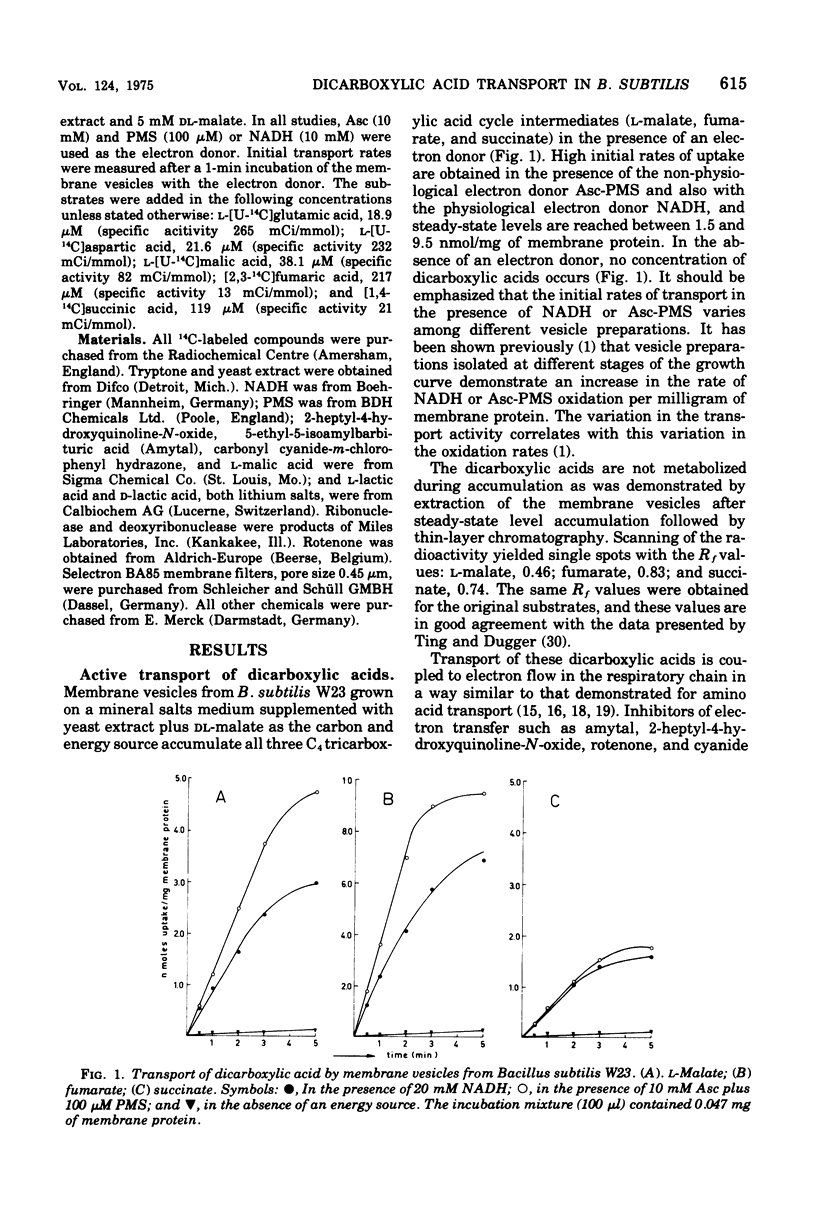
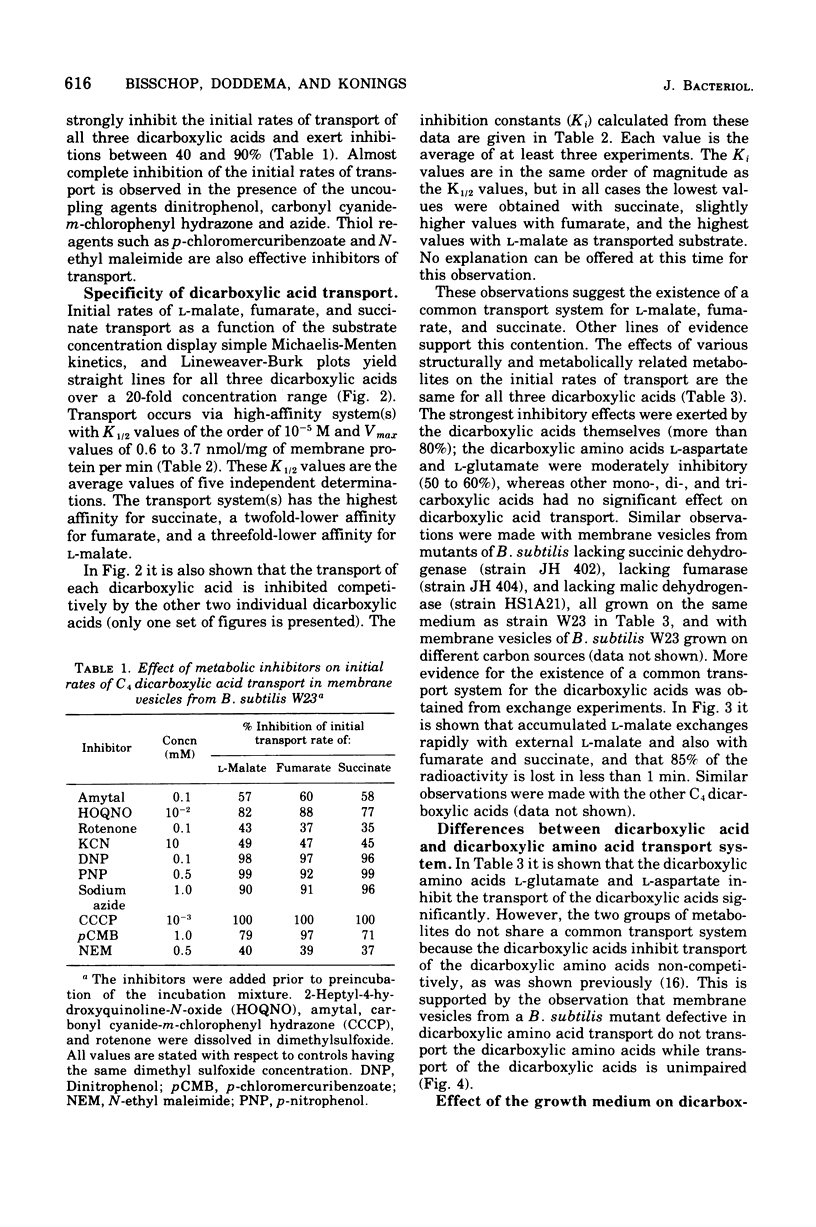
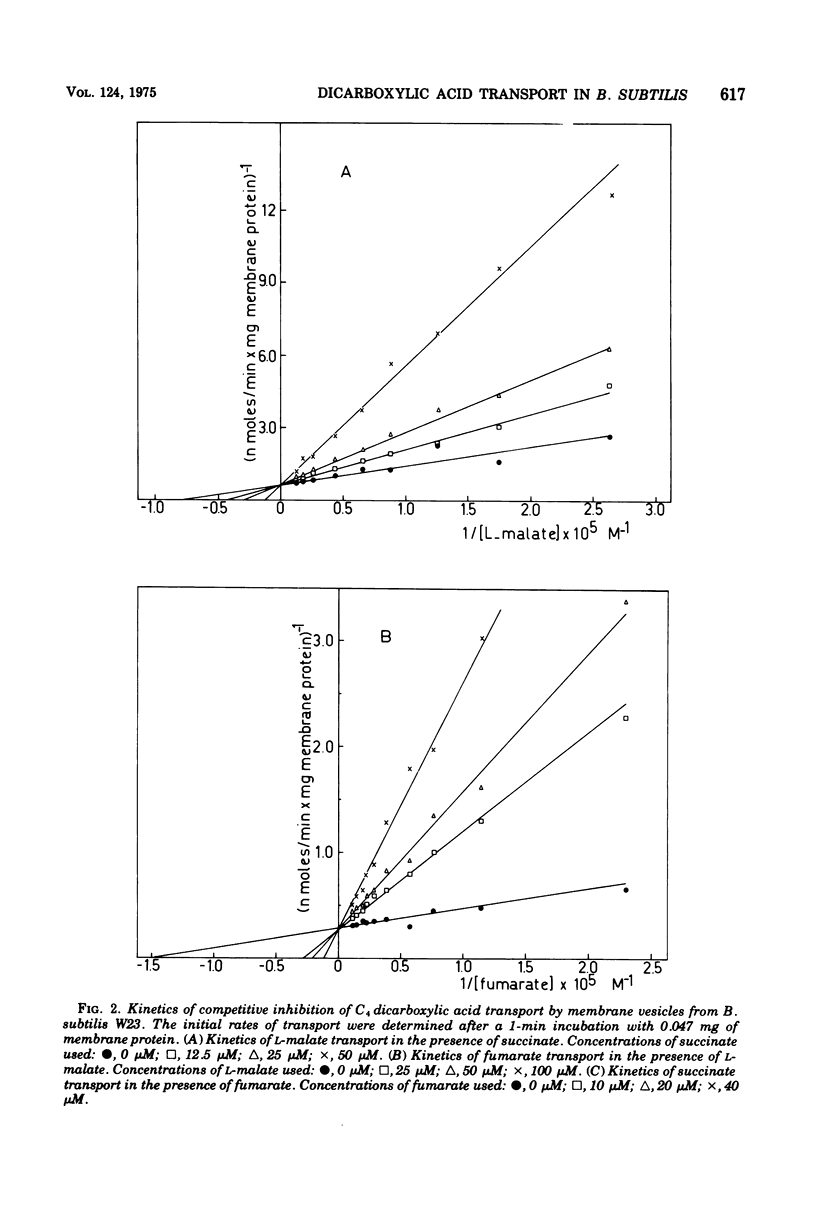
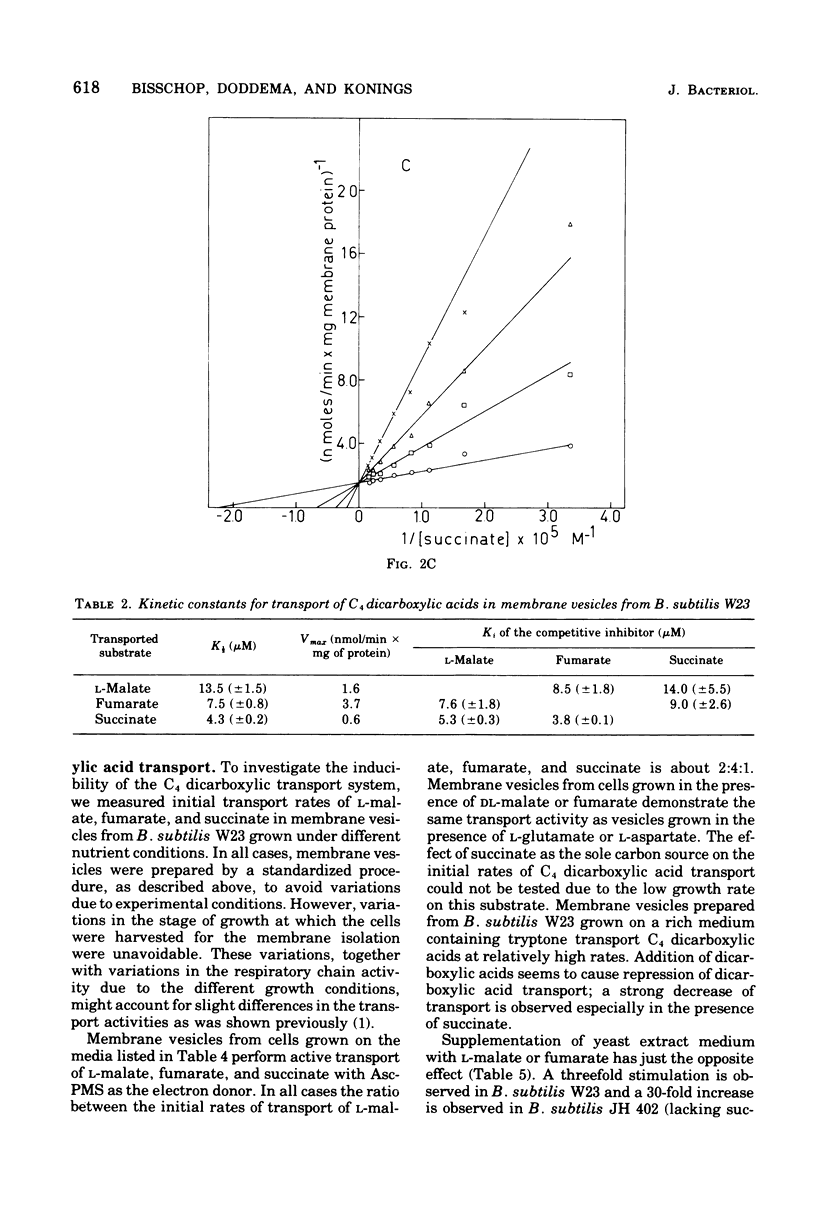
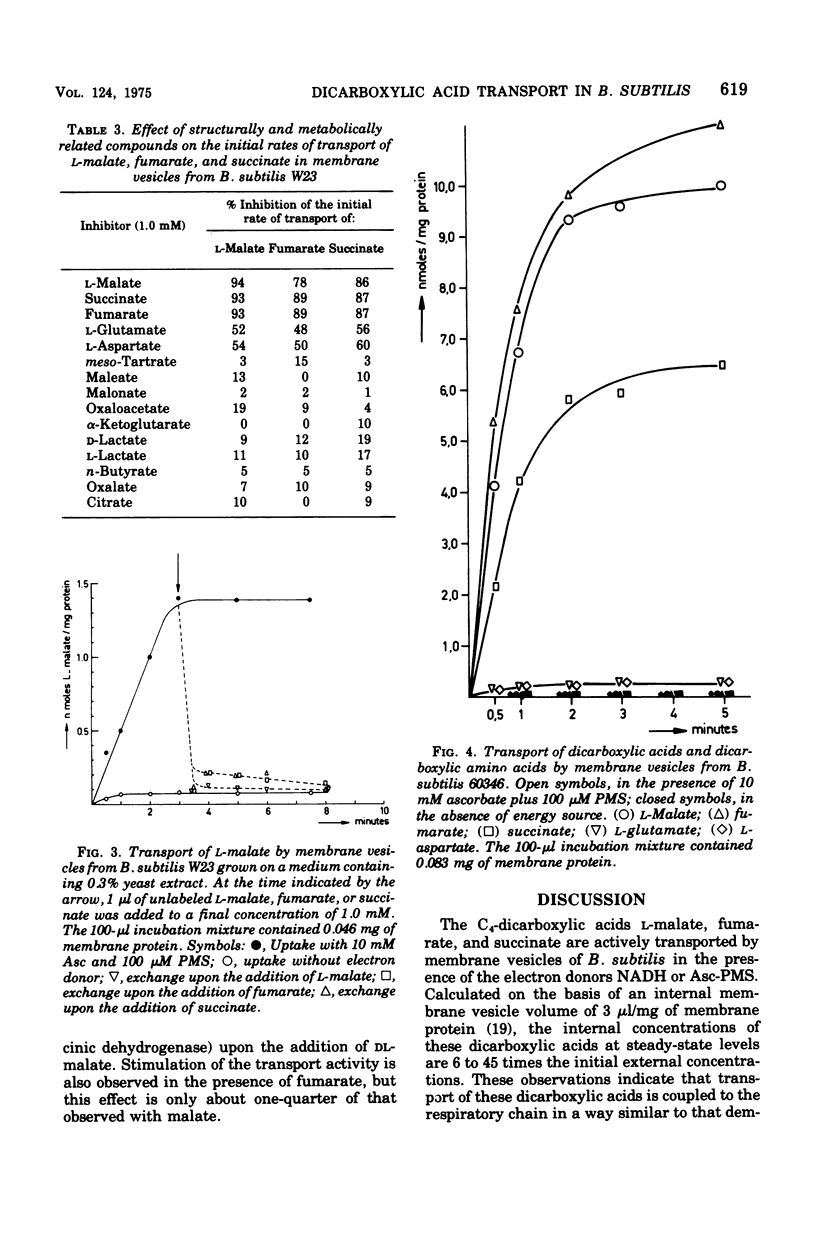
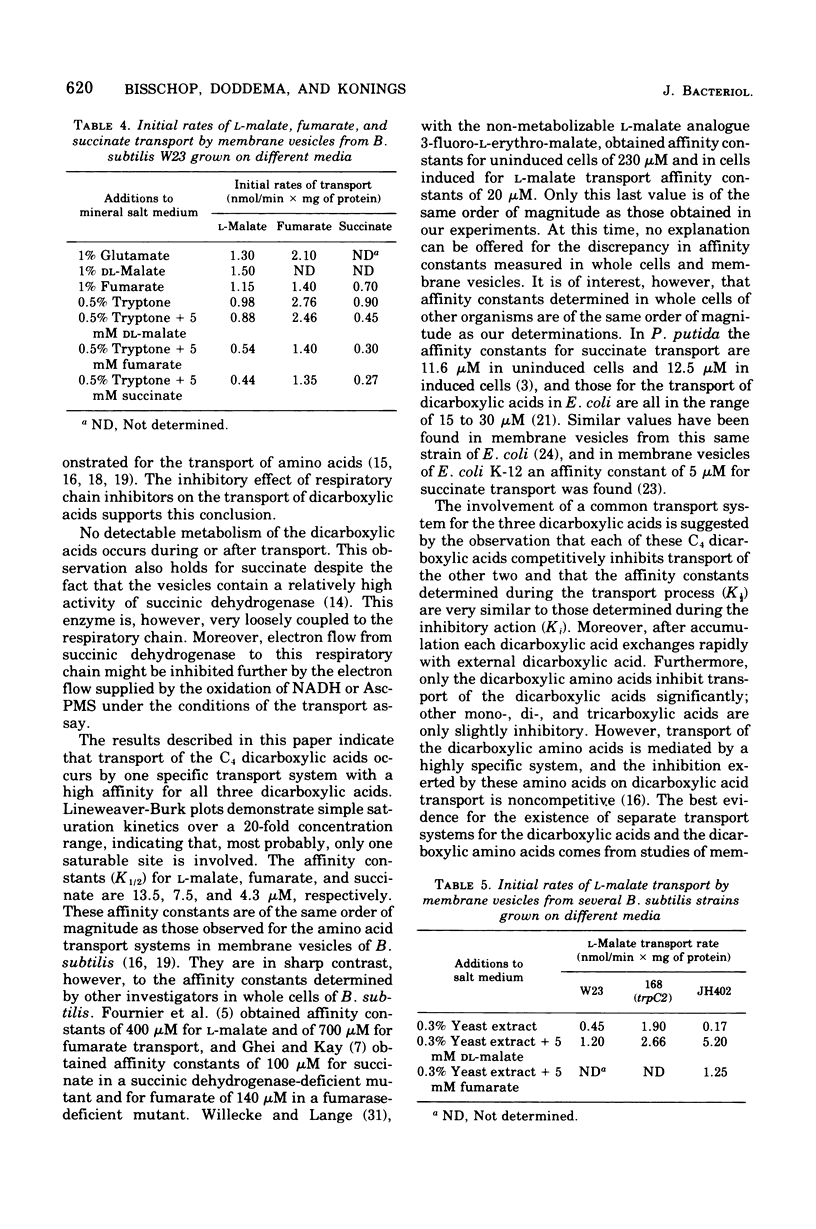
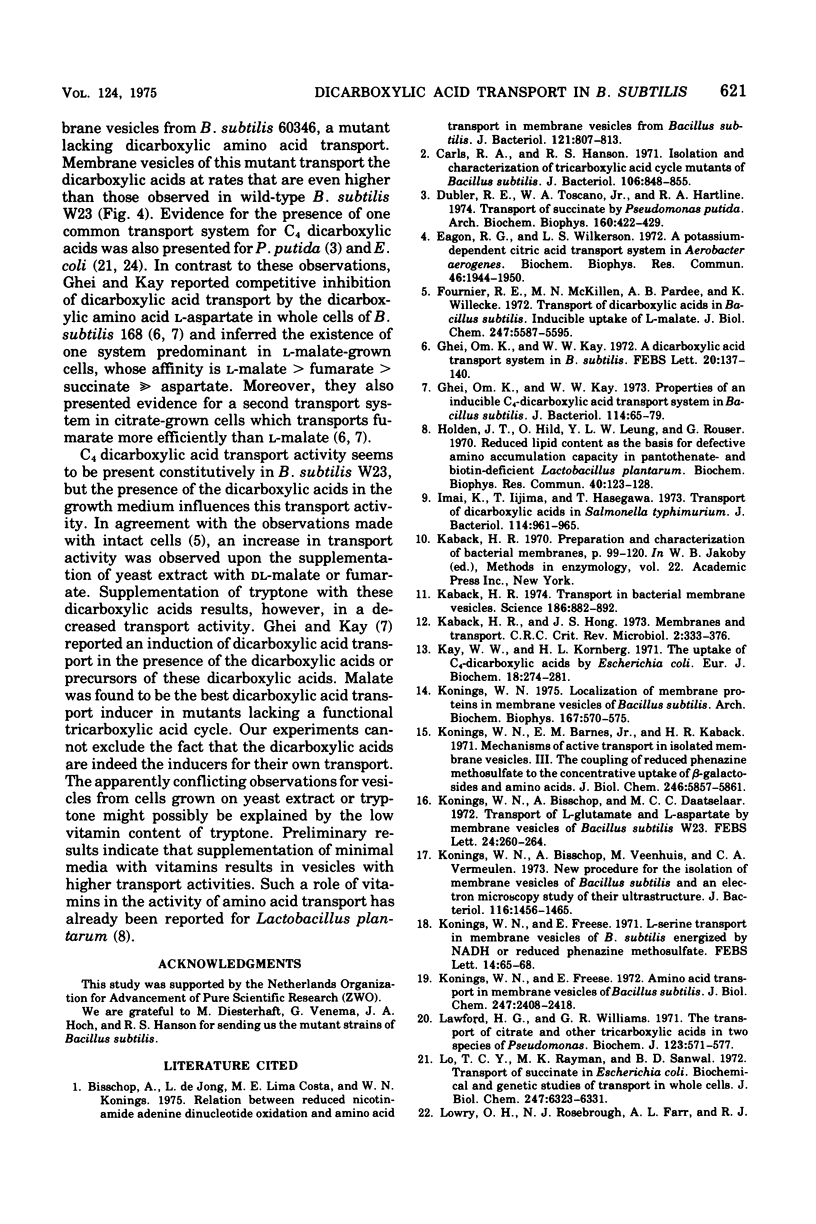
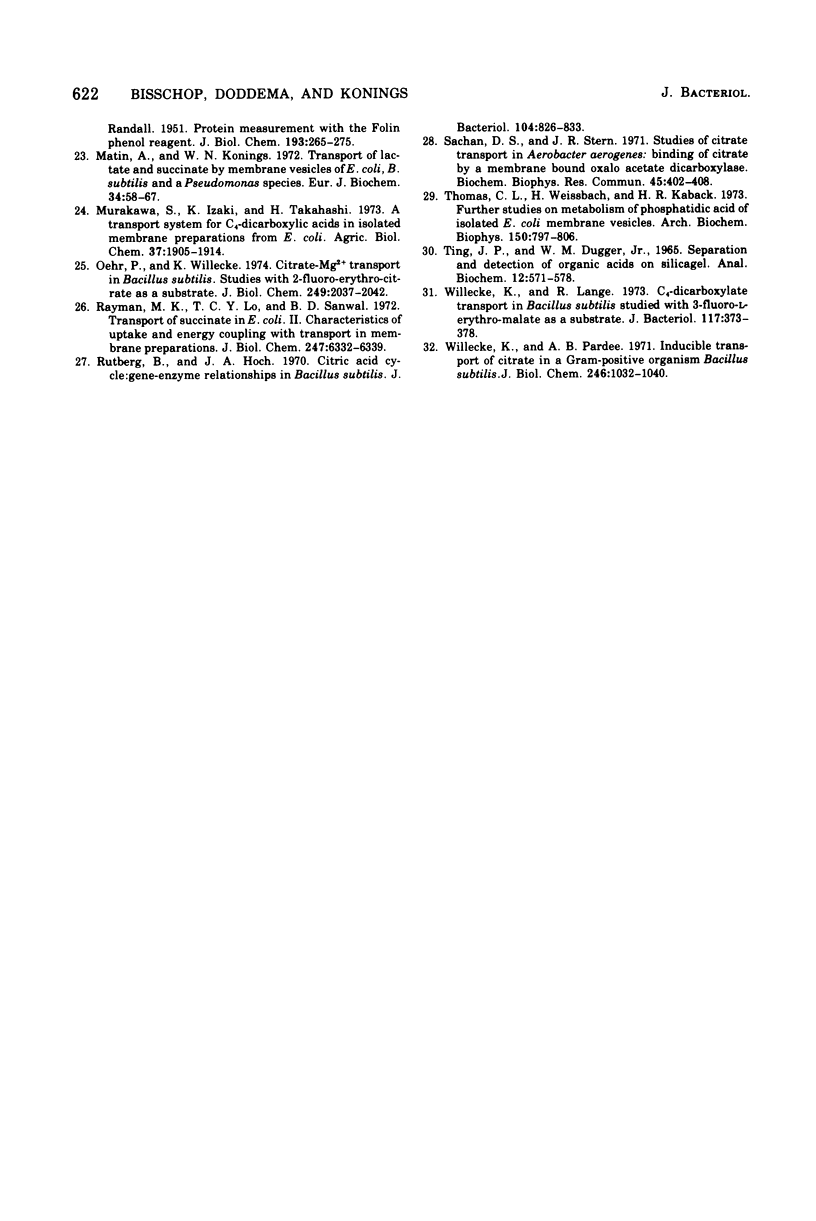
Selected References
These references are in PubMed. This may not be the complete list of references from this article.
- Bisschop A., de Jong L., Lima Costa M. E., Konings W. N. Relation between reduced nicotinamide adenine dinucleotide oxidation and amino acid transport in membrane vesicles from Bacillus subtilis. J Bacteriol. 1975 Mar;121(3):807–813. doi: 10.1128/jb.121.3.807-813.1975. [DOI] [PMC free article] [PubMed] [Google Scholar]
- Carls R. A., Hanson R. S. Isolation and characterization of tricarboxylic acid cycle mutants of Bacillus subtilis. J Bacteriol. 1971 Jun;106(3):848–855. doi: 10.1128/jb.106.3.848-855.1971. [DOI] [PMC free article] [PubMed] [Google Scholar]
- Dubler R. E., Toscano W. A., Jr, Hartline R. A. Transport of succinate by Pseudomonas putida. Arch Biochem Biophys. 1974 Feb;160(2):422–429. doi: 10.1016/0003-9861(74)90416-0. [DOI] [PubMed] [Google Scholar]
- Eagon R. G., Wilkerson L. S. A potassium-dependent citric acid transport system in Aerobacter aerogenes. Biochem Biophys Res Commun. 1972 Mar 10;46(5):1944–1950. doi: 10.1016/0006-291x(72)90074-5. [DOI] [PubMed] [Google Scholar]
- Fournier R. E., McKillen M. N., Pardee A. B., Willecke K. Transport of dicarboxylic acids in Bacillus subtilis. Inducible uptake of L-malate. J Biol Chem. 1972 Sep 10;247(17):5587–5595. [PubMed] [Google Scholar]
- Ghei O. K., Kay W. W. Properties of an inducible C 4 -dicarboxylic acid transport system in Bacillus subtilis. J Bacteriol. 1973 Apr;114(1):65–79. doi: 10.1128/jb.114.1.65-79.1973. [DOI] [PMC free article] [PubMed] [Google Scholar]
- Ghei Om K., Kay William W. A Dicarboxyclic acid transport system in Bacillus subtilis. FEBS Lett. 1972 Feb 1;20(2):137–140. doi: 10.1016/0014-5793(72)80777-4. [DOI] [PubMed] [Google Scholar]
- Holden J. T., Hild O., Wong-Leung Y. L., Rouser G. Reduced lipid content as the basis for defective amino acid accumulation capacity in pantothenate- and biotin-deficient Lactobacillus plantarum. Biochem Biophys Res Commun. 1970 Jul 13;40(1):123–128. doi: 10.1016/0006-291x(70)91055-7. [DOI] [PubMed] [Google Scholar]
- Imai K., Iijima T., Hasegawa T. Transport of tricarboxylic acids in Salmonella typhimurium. J Bacteriol. 1973 Jun;114(3):961–965. doi: 10.1128/jb.114.3.961-965.1973. [DOI] [PMC free article] [PubMed] [Google Scholar]
- Kaback H. R. Transport studies in bacterial membrane vesicles. Science. 1974 Dec 6;186(4167):882–892. doi: 10.1126/science.186.4167.882. [DOI] [PubMed] [Google Scholar]
- Kay W. W., Kornberg H. L. The uptake of C4-dicarboxylic acids by Escherichia coli. Eur J Biochem. 1971 Jan;18(2):274–281. doi: 10.1111/j.1432-1033.1971.tb01240.x. [DOI] [PubMed] [Google Scholar]
- Konings W. N., Barnes E. M., Jr, Kaback H. R. Mechanisms of active transport in isolated membrane vesicles. 2. The coupling of reduced phenazine methosulfate to the concentrative uptake of beta-galactosides and amino acids. J Biol Chem. 1971 Oct 10;246(19):5857–5861. [PubMed] [Google Scholar]
- Konings W. N., Bisschop A., Daatselaar M. C.C. Transport of L-glutamate and L-aspartate by membrane vesicles of Bacillus subtilis W 23. FEBS Lett. 1972 Aug 15;24(3):260–264. doi: 10.1016/0014-5793(72)80368-5. [DOI] [PubMed] [Google Scholar]
- Konings W. N., Bisschop A., Veenhuis M., Vermeulen C. A. New procedure for the isolation of membrane vesicles of Bacillus subtilis and an electron microscopy study of their ultrastructure. J Bacteriol. 1973 Dec;116(3):1456–1465. doi: 10.1128/jb.116.3.1456-1465.1973. [DOI] [PMC free article] [PubMed] [Google Scholar]
- Konings W. N., Freese E. Amino acid transport in membrane vesicles of Bacillus subtilis. J Biol Chem. 1972 Apr 25;247(8):2408–2418. [PubMed] [Google Scholar]
- Konings W. N., Freese E. L-serine transport in membrane vesicles of Bacillus subtilis energized by NADH or reduced phenazine methosulfate. FEBS Lett. 1971 Apr 12;14(1):65–68. doi: 10.1016/0014-5793(71)80276-4. [DOI] [PubMed] [Google Scholar]
- Konings W. N. Localization of membrane proteins in membrane vesicles of Bacillus subtilis. Arch Biochem Biophys. 1975 Apr;167(2):570–580. doi: 10.1016/0003-9861(75)90500-7. [DOI] [PubMed] [Google Scholar]
- LOWRY O. H., ROSEBROUGH N. J., FARR A. L., RANDALL R. J. Protein measurement with the Folin phenol reagent. J Biol Chem. 1951 Nov;193(1):265–275. [PubMed] [Google Scholar]
- Lawford H. G., Williams G. R. The transport of citrate and other tricarboxylic acids in two species of Pseudomonas. Biochem J. 1971 Jul;123(4):571–577. doi: 10.1042/bj1230571. [DOI] [PMC free article] [PubMed] [Google Scholar]
- Lo T. C., Rayman M. K., Sanwal B. D. Transport of succinate in Escherichia coli. I. Biochemical and genetic studies of transport in whole cells. J Biol Chem. 1972 Oct 10;247(19):6323–6331. [PubMed] [Google Scholar]
- Matin A., Konings W. N. Transport of lactate and succinate by membrane vesicles of Escherichia coli, Bacillus subtilis and a pseudomonas species. Eur J Biochem. 1973 Apr 2;34(1):58–67. doi: 10.1111/j.1432-1033.1973.tb02728.x. [DOI] [PubMed] [Google Scholar]
- Oehr P., Willecke K. Citrate-Mg2+ transport in Bacillus subtilis. Studies with 2-fluoro-L-erythro-citrate as a substrate. J Biol Chem. 1974 Apr 10;249(7):2037–2042. [PubMed] [Google Scholar]
- Rayman M. K., Lo T. C., Sanwal B. D. Transport of succinate in Escherichia coli. II. Characteristics of uptake and energy coupling with transport in membrane preparations. J Biol Chem. 1972 Oct 10;247(19):6332–6339. [PubMed] [Google Scholar]
- Rutberg B., Hoch J. A. Citric acid cycle: gene-enzyme relationships in Bacillus subtilis. J Bacteriol. 1970 Nov;104(2):826–833. doi: 10.1128/jb.104.2.826-833.1970. [DOI] [PMC free article] [PubMed] [Google Scholar]
- Sachan D. S., Stern J. R. Studies of citrate transport in Aerobacter aerogenes: binding of citrate by a membrane bound oxalacetate decarboxylase. Biochem Biophys Res Commun. 1971 Oct 15;45(2):402–408. doi: 10.1016/0006-291x(71)90833-3. [DOI] [PubMed] [Google Scholar]
- Thomas E. L., Weissbach H., Kaback H. R. Further studies on metabolism of phosphatidic acid of isolated E. coli membrane vesicles. Arch Biochem Biophys. 1972 Jun;150(2):797–806. doi: 10.1016/0003-9861(72)90101-4. [DOI] [PubMed] [Google Scholar]
- Ting I. P., Dugger W. M., Jr Separation and detection of organic acids on silica gel. Anal Biochem. 1965 Sep;12(3):571–578. doi: 10.1016/0003-2697(65)90224-1. [DOI] [PubMed] [Google Scholar]
- Willecke K., Lange R. C4-dicarboxylate transport in Bacillus subtilis studied with 3-fluoro-L-erythro-malate as a substrate. J Bacteriol. 1974 Feb;117(2):373–378. doi: 10.1128/jb.117.2.373-378.1974. [DOI] [PMC free article] [PubMed] [Google Scholar]
- Willecke K., Pardee A. B. Inducible transport of citrate in a Gram-positive bacterium, Bacillus subtilis. J Biol Chem. 1971 Feb 25;246(4):1032–1040. [PubMed] [Google Scholar]