Abstract
Pediococcus pentosaceus is commonly found among the adventitious microflora of Cheddar cheese. When this organism was incubated with L-(+)-lactate under anaerobic conditions, L-(+)-lactate was rapidly converted to D-(-)-lactate until racemic (DL) lactate was present. Under aerobic conditions this initial reaction was followed by a slower reaction resulting in the use of both lactate isomers and in the production of acetate and CO2. With intact cells the lactate oxidation system had an optimum pH of 5 to 6, depending on the initial lactate concentration. Cells grown anaerobically possessed lactate-oxidizing activity which increased two- to fourfold as sugar was exhausted from the medium. Aerobic growth further increased specific activities. Cheddar cheese was made with the deliberate addition of P. pentosaceus. When the resulting cheese was grated to expose a large surface area to O2, lactate was converted to acetate at a rate which depended on the density of pediococci in the cheese. The lactate oxidation system remained active in cheese which had been ripened for 6 months.
Full text
PDF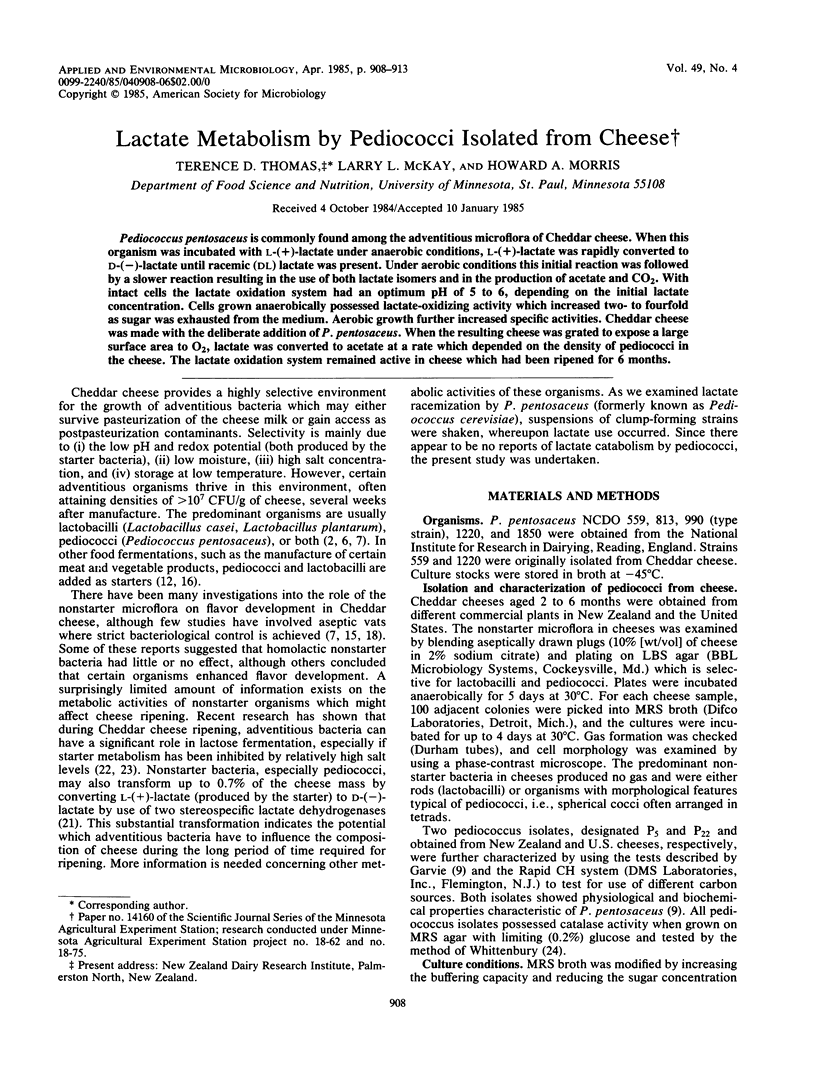
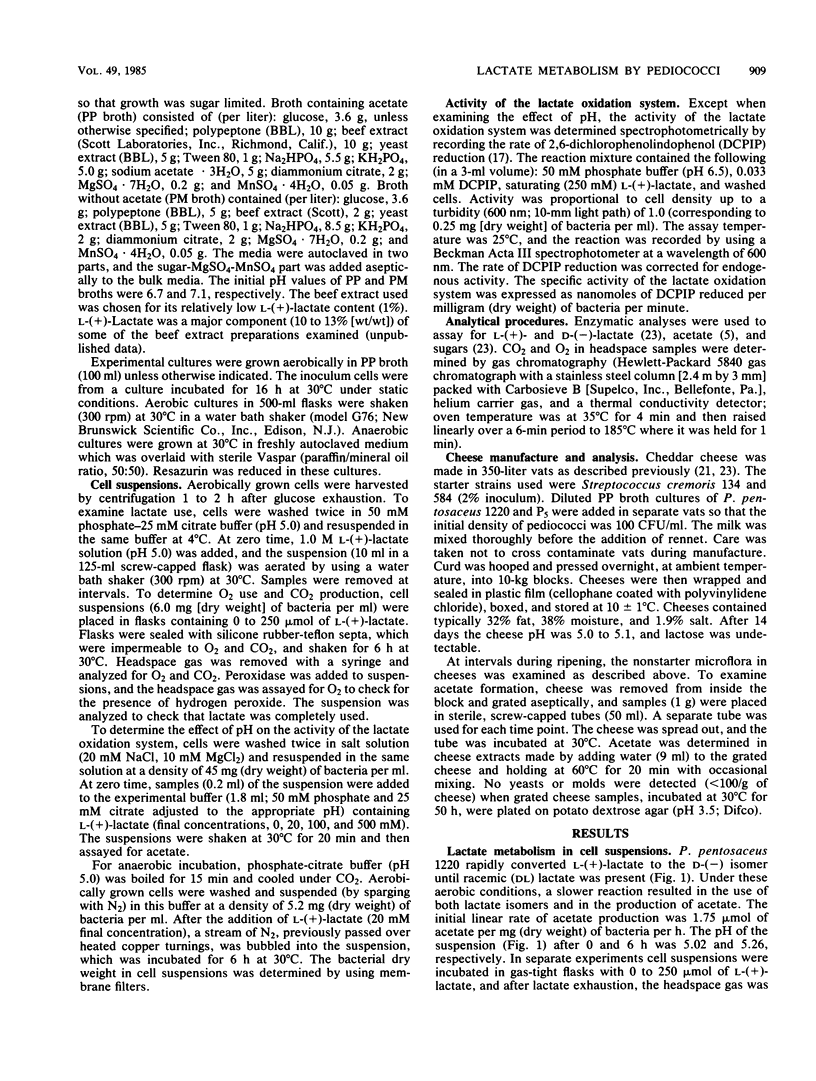
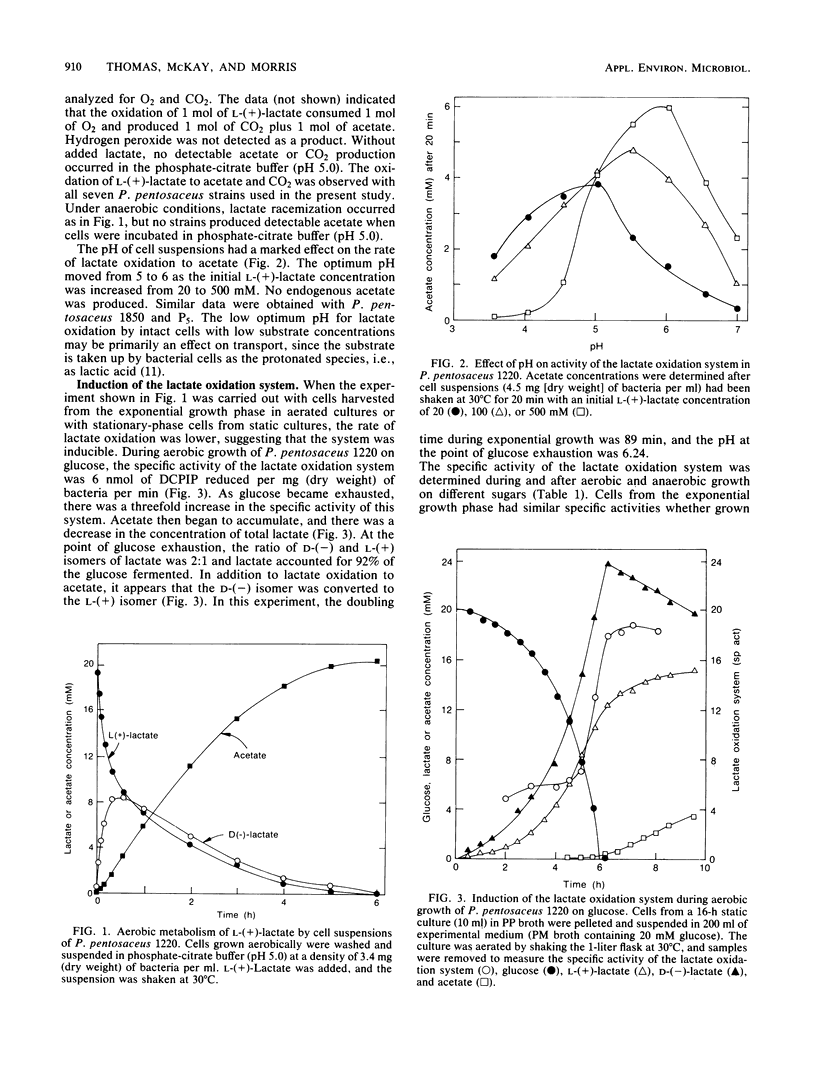
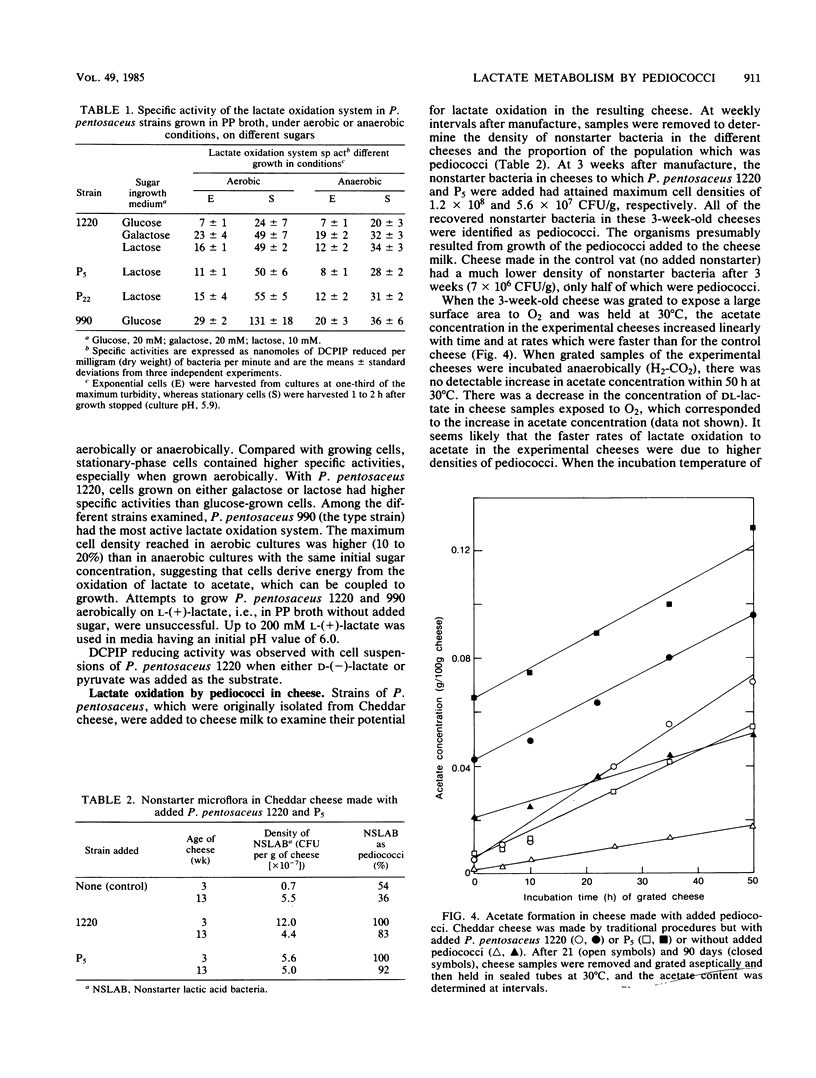
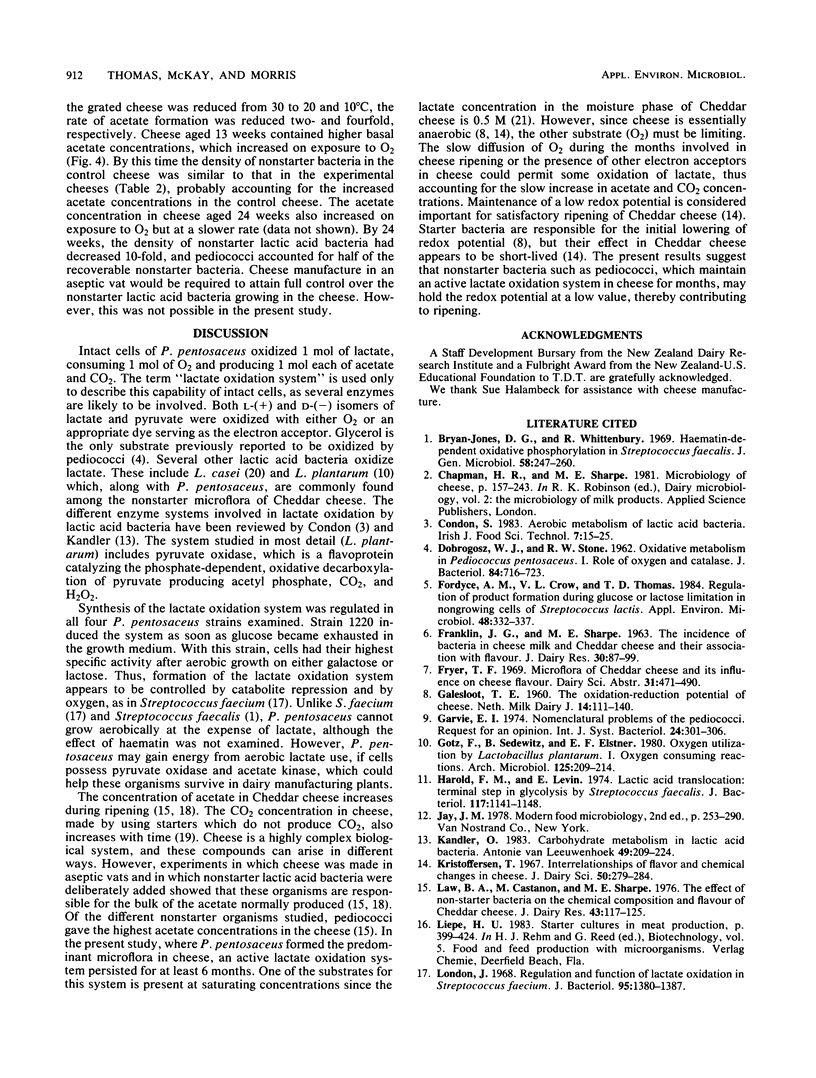
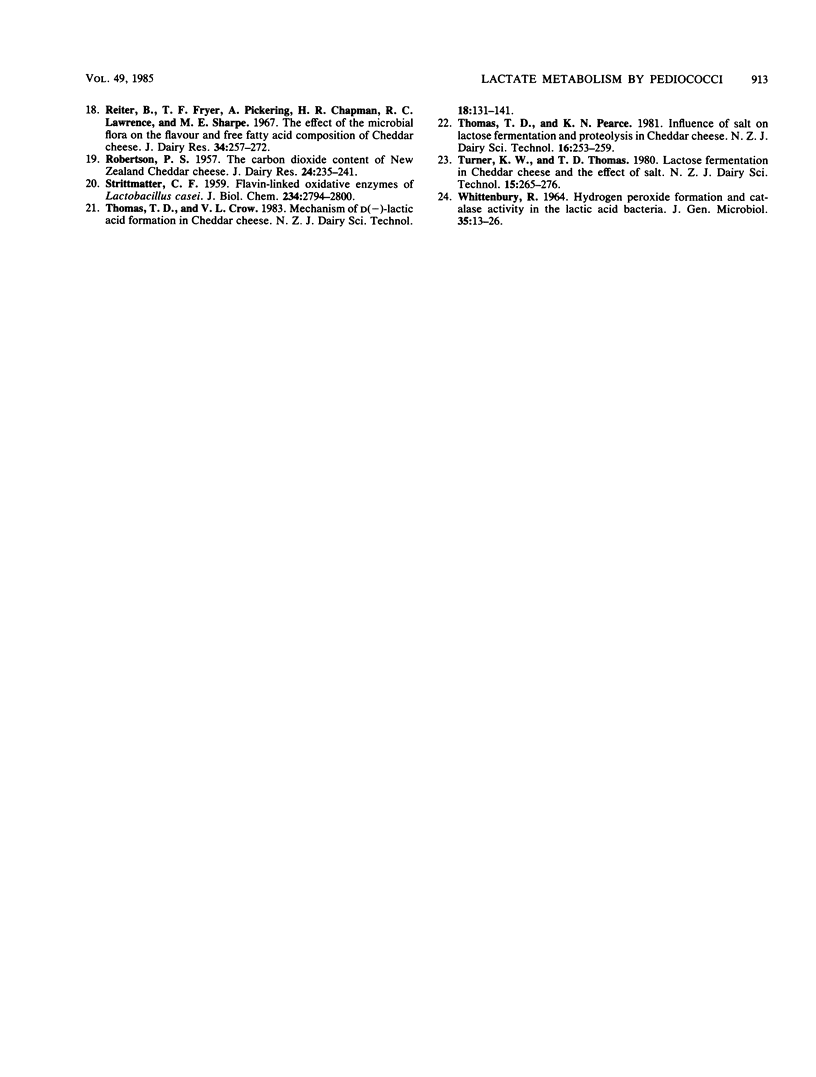
Selected References
These references are in PubMed. This may not be the complete list of references from this article.
- Bryan-Jones D. G., Whittenbury R. Haematin-dependent oxidative phosphorylation in Streptococcus faecalis. J Gen Microbiol. 1969 Oct;58(2):247–260. doi: 10.1099/00221287-58-2-247. [DOI] [PubMed] [Google Scholar]
- DOBROGOSZ W. J., STONE R. W. Oxidative metabolism in Pediococcus pentosaceus. I. Role of oxygen and catalase. J Bacteriol. 1962 Oct;84:716–723. doi: 10.1128/jb.84.4.716-723.1962. [DOI] [PMC free article] [PubMed] [Google Scholar]
- Fordyce A. M., Crow V. L., Thomas T. D. Regulation of product formation during glucose or lactose limitation in nongrowing cells of Streptococcus lactis. Appl Environ Microbiol. 1984 Aug;48(2):332–337. doi: 10.1128/aem.48.2.332-337.1984. [DOI] [PMC free article] [PubMed] [Google Scholar]
- Götz F., Sedewitz B., Elstner E. F. Oxygen utilization by Lactobacillus plantarum. I. Oxygen consuming reactions. Arch Microbiol. 1980 Apr;125(3):209–214. doi: 10.1007/BF00446878. [DOI] [PubMed] [Google Scholar]
- Harold F. M., Levin E. Lactic acid translocation: terminal step in glycolysis by Streptococcus faecalis. J Bacteriol. 1974 Mar;117(3):1141–1148. doi: 10.1128/jb.117.3.1141-1148.1974. [DOI] [PMC free article] [PubMed] [Google Scholar]
- Kandler O. Carbohydrate metabolism in lactic acid bacteria. Antonie Van Leeuwenhoek. 1983 Sep;49(3):209–224. doi: 10.1007/BF00399499. [DOI] [PubMed] [Google Scholar]
- London J. Regulation and function of lactate oxidation in Streptococcus faecium. J Bacteriol. 1968 Apr;95(4):1380–1387. doi: 10.1128/jb.95.4.1380-1387.1968. [DOI] [PMC free article] [PubMed] [Google Scholar]
- STRITTMATTER C. F. Flavin-linked oxidative enzymes of Lactobacillus casei. J Biol Chem. 1959 Oct;234:2794–2800. [PubMed] [Google Scholar]
- WHITTENBURY R. HYDROGEN PEROXIDE FORMATION AND CATALASE ACTIVITY IN THE LACTIC ACID BACTERIA. J Gen Microbiol. 1964 Apr;35:13–26. doi: 10.1099/00221287-35-1-13. [DOI] [PubMed] [Google Scholar]