Abstract
Dissolved substances released during decomposition of the white water lily (Nymphaea odorata) can alter the growth rate of Okefenokee Swamp bacterioplankton. In microcosm experiments dissolved compounds released from senescent Nymphaea leaves caused a transient reduction in the abundance and activity of water column bacterioplankton, followed by a period of intense bacterial growth. Rates of [3H]thymidine incorporation and turnover of dissolved d-glucose were depressed by over 85%, 3 h after the addition of Nymphaea leachates to microcosms containing Okefenokee Swamp water. Bacterial activity subsequently recovered; after 20 h [3H]thymidine incorporation in leachate-treated microcosms was 10-fold greater than that in control microcosms. The recovery of activity was due to a shift in the composition of the bacterial population toward resistance to the inhibitory compounds present in Nymphaea leachates. Inhibitory compounds released during the decomposition of aquatic macrophytes thus act as selective agents which alter the community structure of the bacterial population with respect to leachate resistance. Soluble compounds derived from macrophyte decomposition influence the rate of bacterial secondary production and the availability of microbial biomass to microconsumers.
Full text
PDF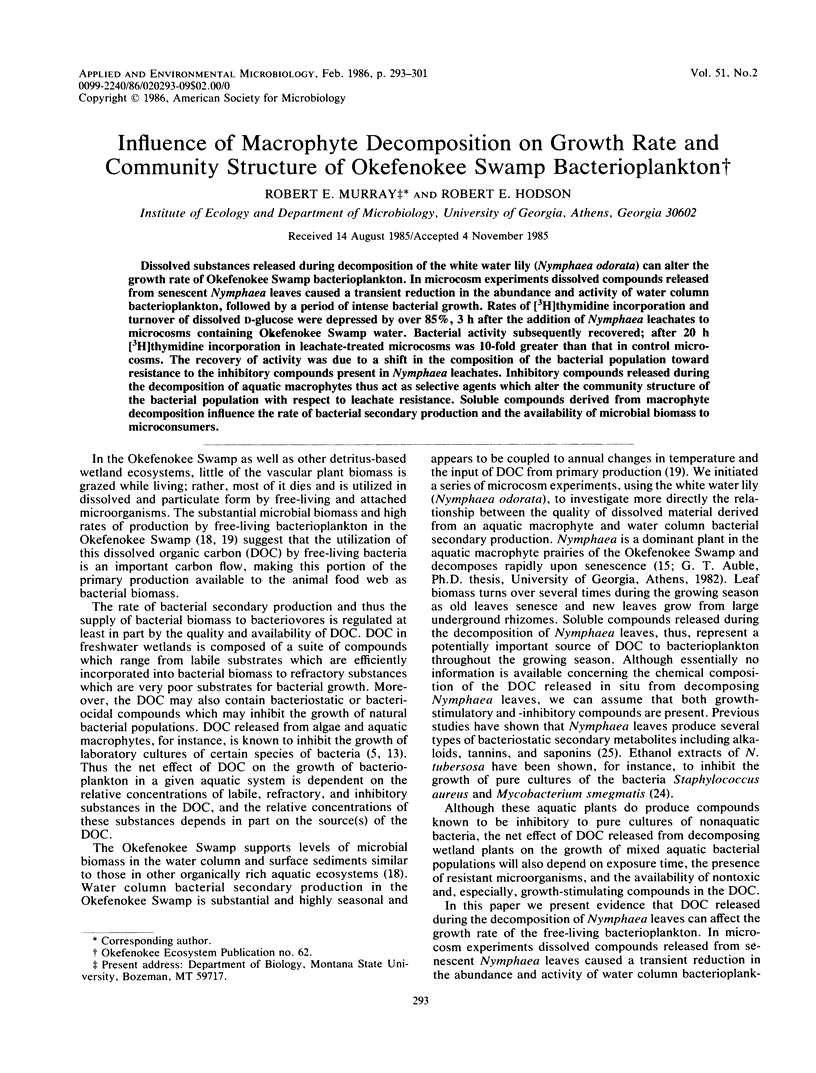
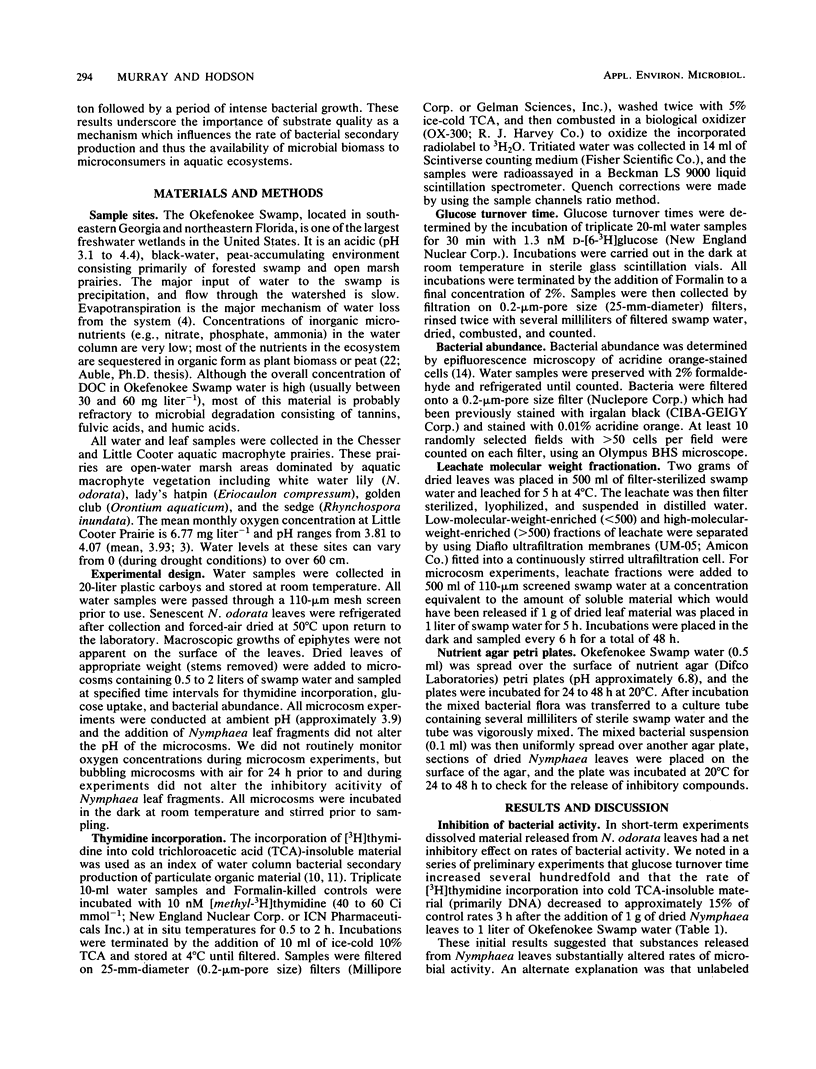
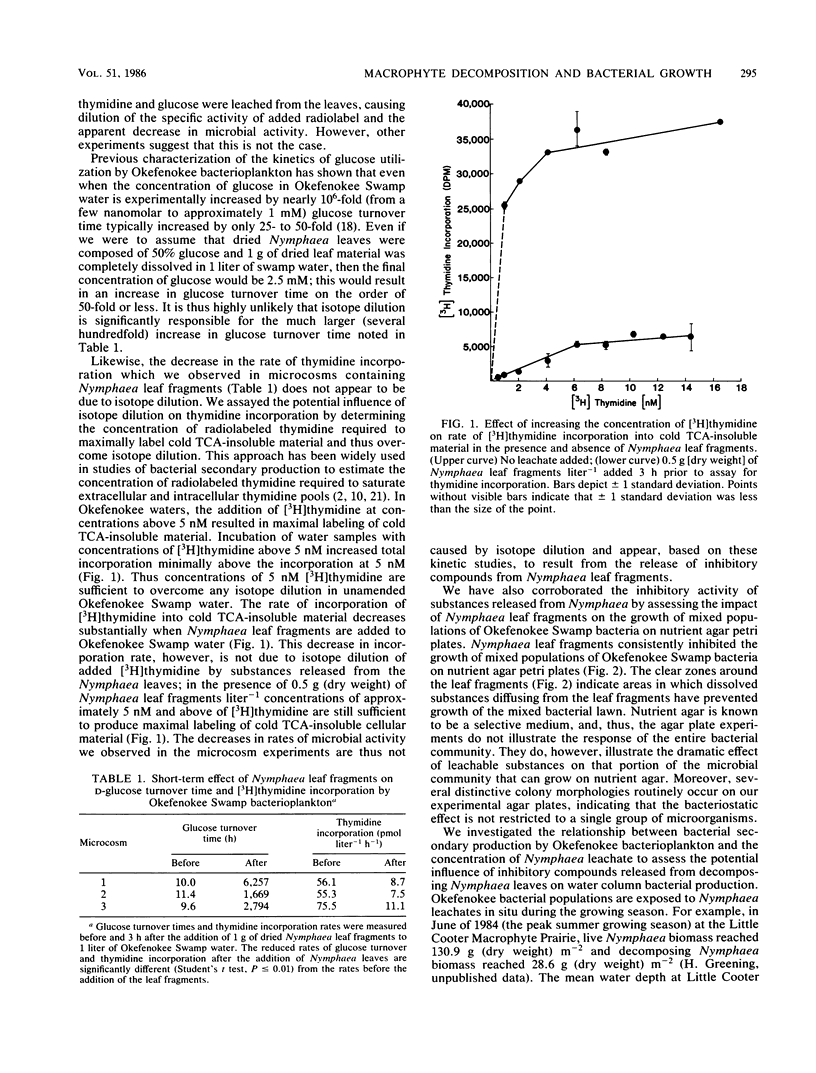
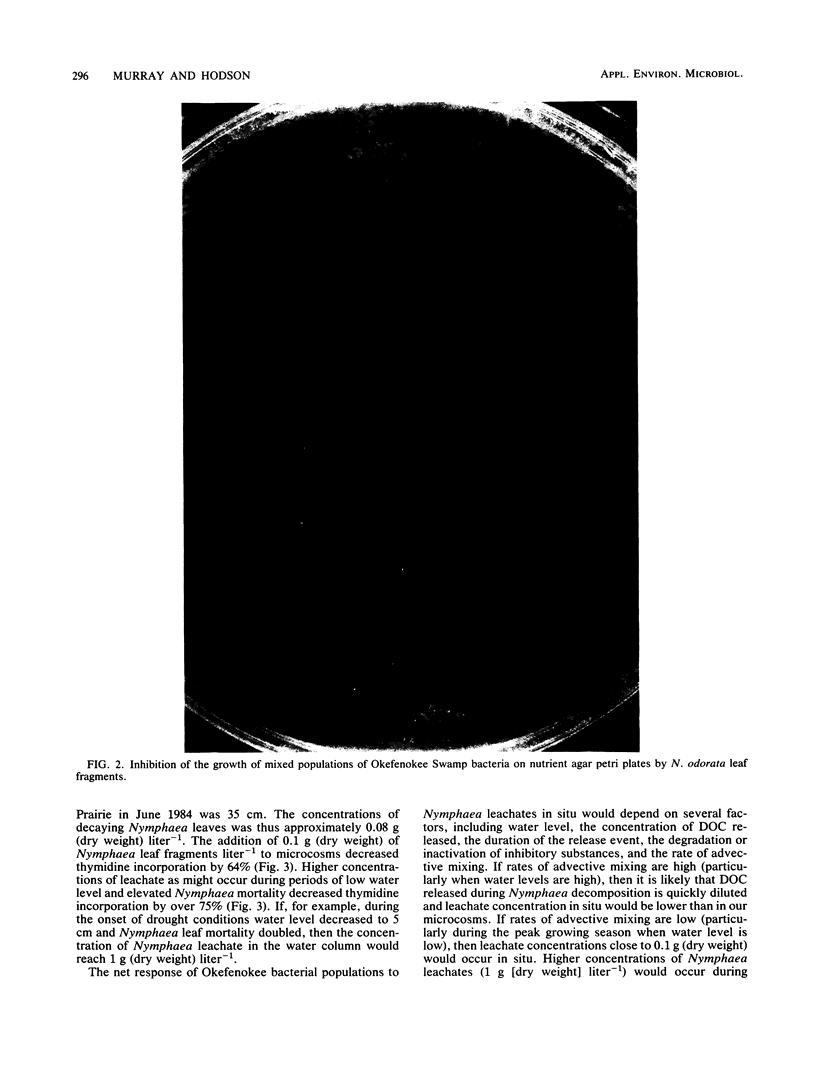
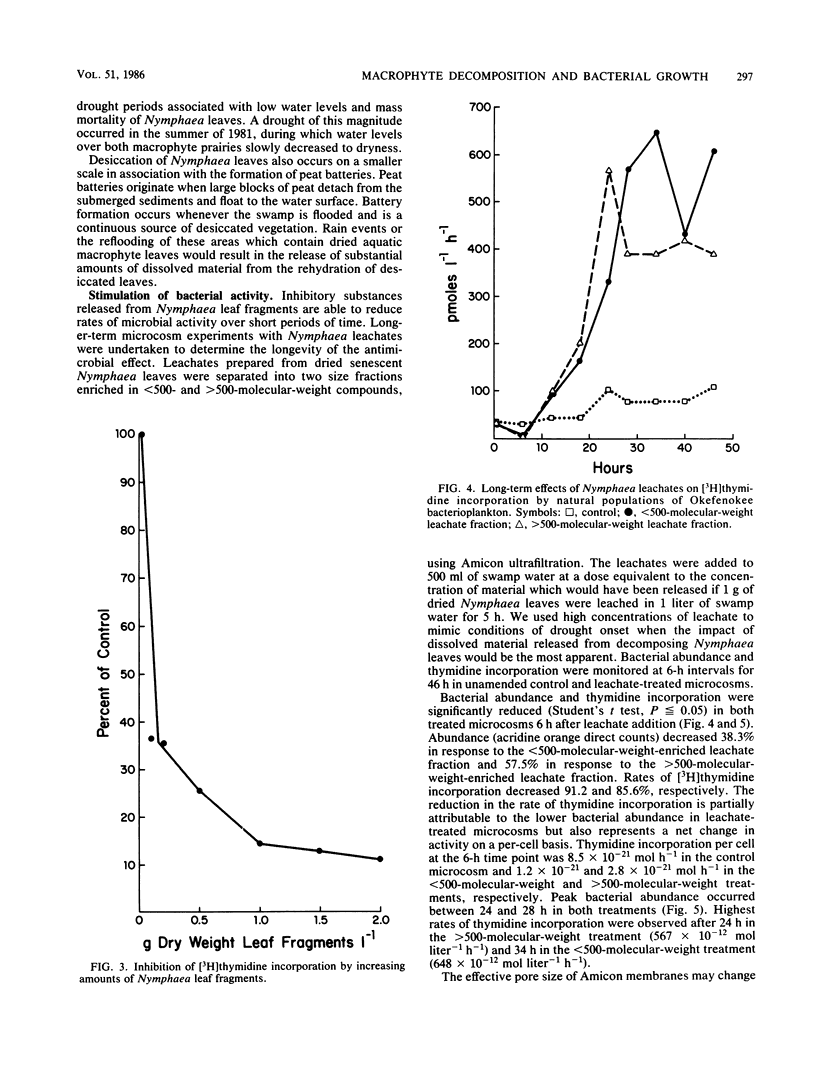
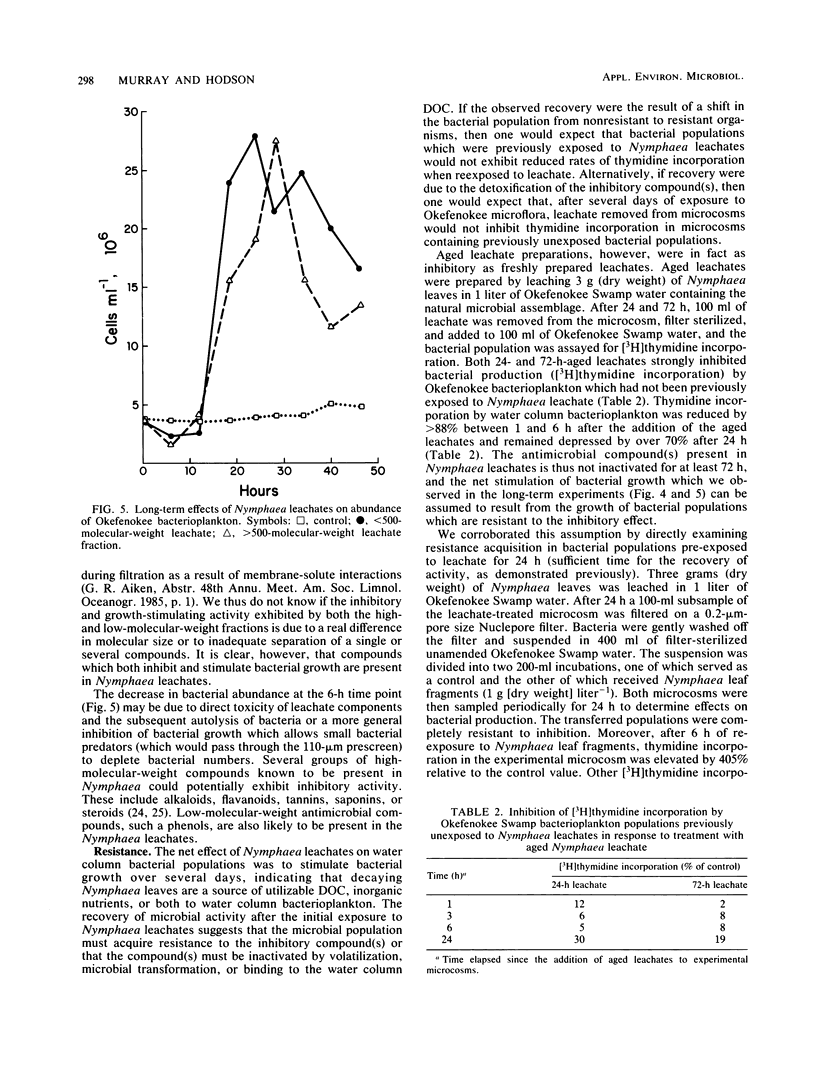
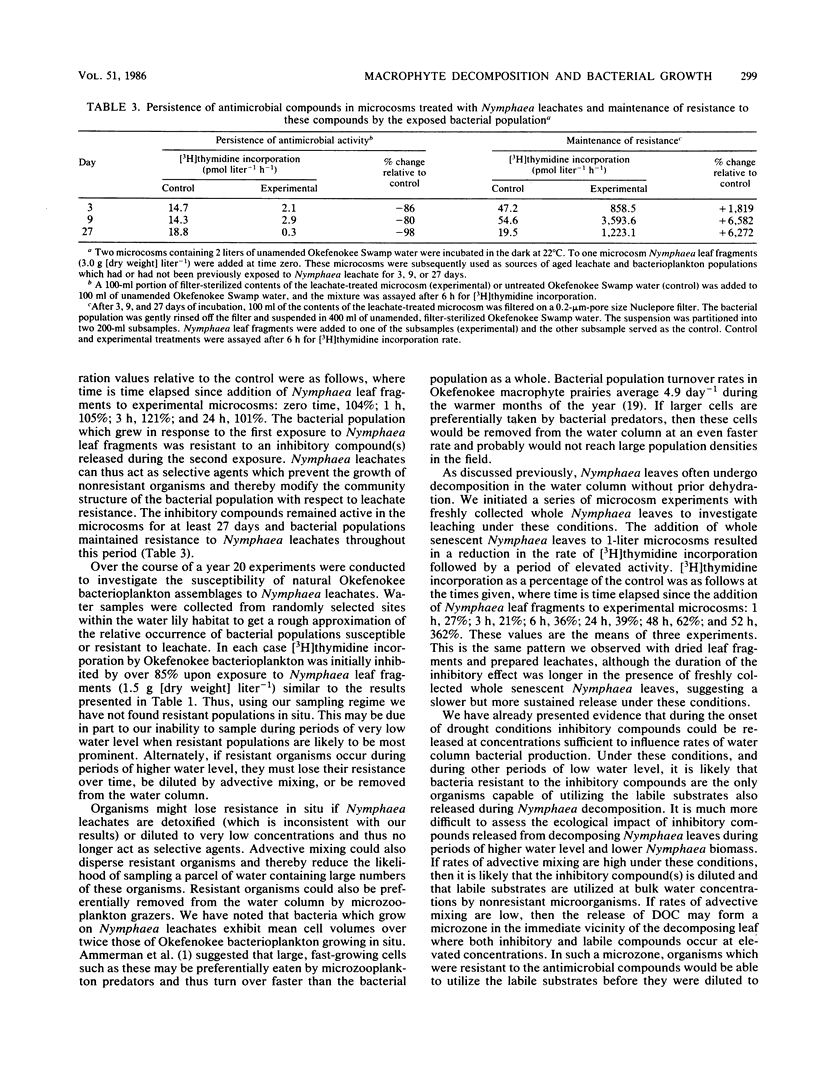
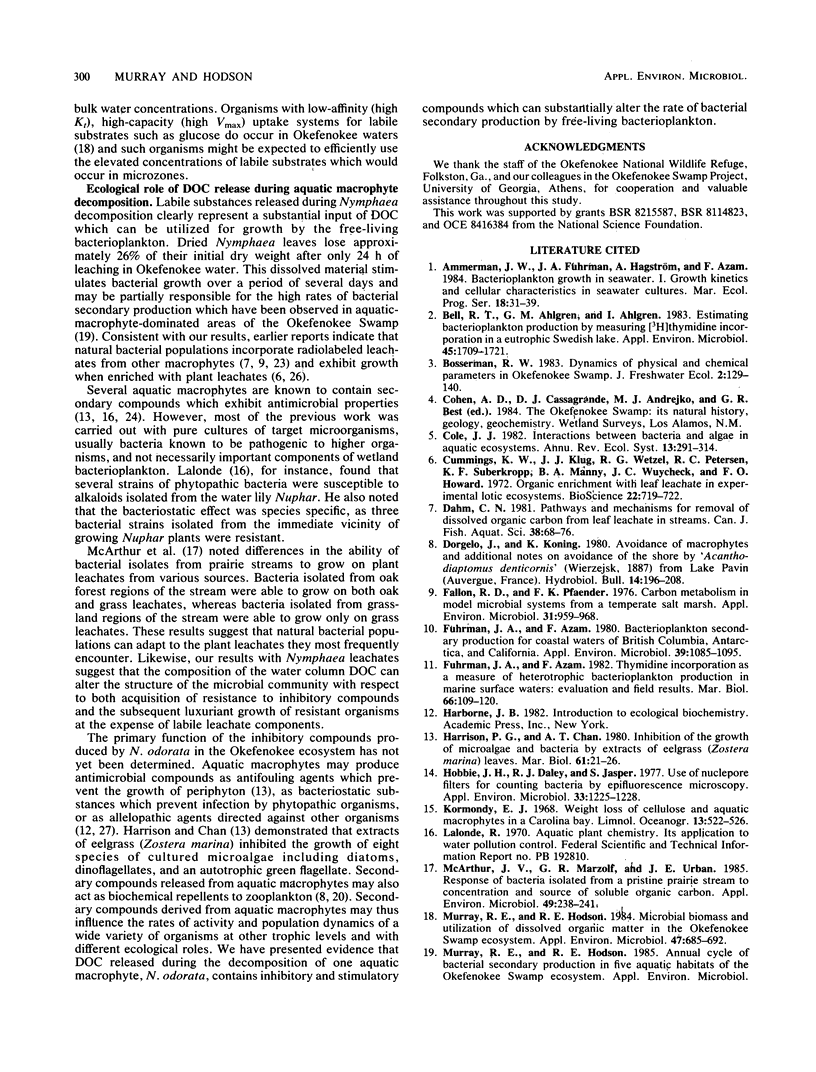
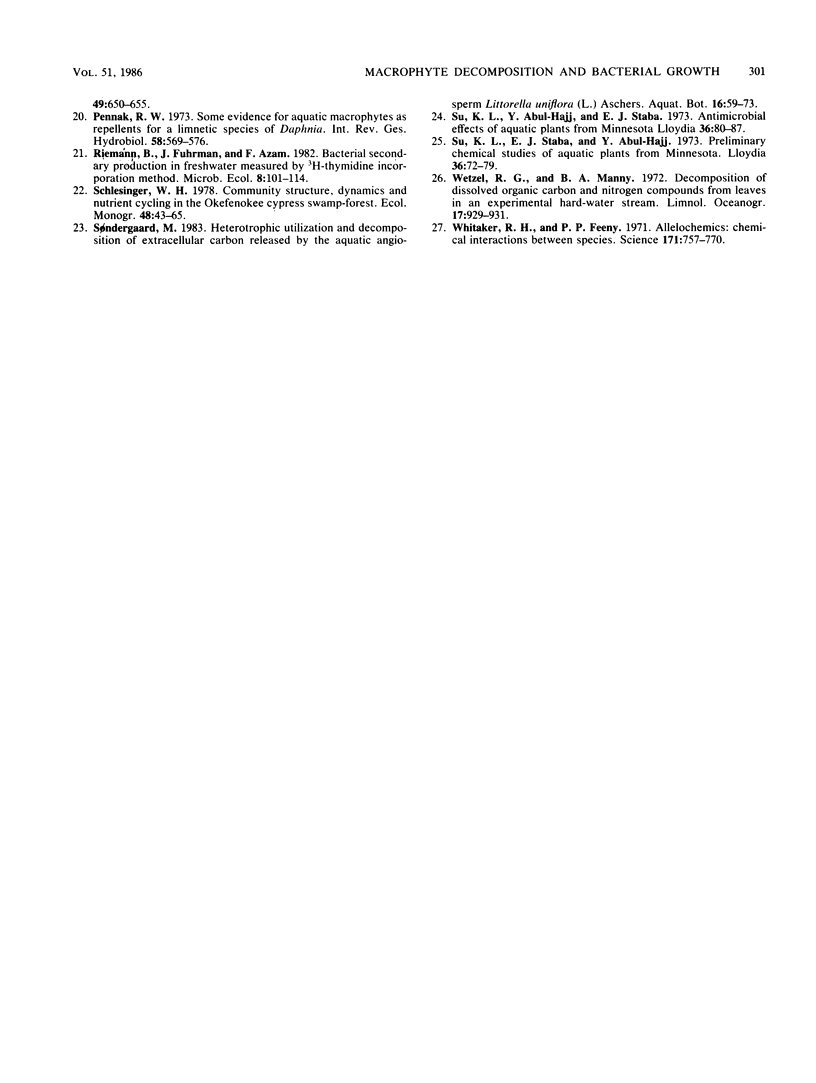
Images in this article
Selected References
These references are in PubMed. This may not be the complete list of references from this article.
- Bell R. T., Ahlgren G. M., Ahlgren I. Estimating Bacterioplankton Production by Measuring [H]thymidine Incorporation in a Eutrophic Swedish Lake. Appl Environ Microbiol. 1983 Jun;45(6):1709–1721. doi: 10.1128/aem.45.6.1709-1721.1983. [DOI] [PMC free article] [PubMed] [Google Scholar]
- Fallon R. D., Pfaender F. K. Carbon metabolism in model microbial systems from a temperate salt marsh. Appl Environ Microbiol. 1976 Jun;31(6):959–968. doi: 10.1128/aem.31.6.959-968.1976. [DOI] [PMC free article] [PubMed] [Google Scholar]
- Fuhrman J. A., Azam F. Bacterioplankton secondary production estimates for coastal waters of british columbia, antarctica, and california. Appl Environ Microbiol. 1980 Jun;39(6):1085–1095. doi: 10.1128/aem.39.6.1085-1095.1980. [DOI] [PMC free article] [PubMed] [Google Scholar]
- Hobbie J. E., Daley R. J., Jasper S. Use of nuclepore filters for counting bacteria by fluorescence microscopy. Appl Environ Microbiol. 1977 May;33(5):1225–1228. doi: 10.1128/aem.33.5.1225-1228.1977. [DOI] [PMC free article] [PubMed] [Google Scholar]
- McArthur J. V., Marzolf G. R., Urban J. E. Response of bacteria isolated from a pristine prairie stream to concentration and source of soluble organic carbon. Appl Environ Microbiol. 1985 Jan;49(1):238–241. doi: 10.1128/aem.49.1.238-241.1985. [DOI] [PMC free article] [PubMed] [Google Scholar]
- Murray R. E., Hodson R. E. Microbial biomass and utilization of dissolved organic matter in the okefenokee swamp ecosystem. Appl Environ Microbiol. 1984 Apr;47(4):685–692. doi: 10.1128/aem.47.4.685-692.1984. [DOI] [PMC free article] [PubMed] [Google Scholar]
- Su K. L., Abul-Hajj Y., Staba E. J. Antimicrobial effects of aquatic plants from Minnesota. Lloydia. 1973 Mar;36(1):80–87. [PubMed] [Google Scholar]
- Whittaker R. H., Feeny P. P. Allelochemics: chemical interactions between species. Science. 1971 Feb 26;171(3973):757–770. doi: 10.1126/science.171.3973.757. [DOI] [PubMed] [Google Scholar]