Abstract
The defect in protein synthesis that is observed in Escherichia coli after transfer to low temperature was studied. For the enzyme beta-galactosidase, the elongation reactions of transcription and translation can take place slowly but normally at 5 C. The time necessary to complete the coupled synthesis of the beta-galactosidase messenger ribonucleic acid and polypeptide chain was found to be about 80 min at 5 C. From this result and from the known length of the beta-galactosidase monomer, it is possible to calculate that at 5 C one amino acid is added to the growing polypeptide chain every 4 s. The initiation of transcription of the beta-galactosidase messenger is inhibited after transfer to 5 C. This fact alone, however, cannot account for all of the phenomena observed at 5 C, because a given amount of messenger yields less enzyme at 5 C than it does at 37 C. Furthermore, in cells induced for short periods at 37 C, the capacity to synthesize beta-galactosidase after transfer to 5 C was found to accumulate linearily with the square of the time of induction. Two alternative models could account for these data. If all ribosomes that initiate translation at 37 C yield complete beta-galactosidase polypeptide chains at 5 C, then an inhibition of translation initiation after transfer to 5 C must be invoked to explain the results. If, on the other hand, a substantial portion of the ribosomes that initiate translation at 37 C do not yield complete beta-galactosidase polypeptides at 5 C, then intracistronic polarity could account for the data, and there is no need to invoke an inhibition of translation initiation at 5 C.
Full text
PDF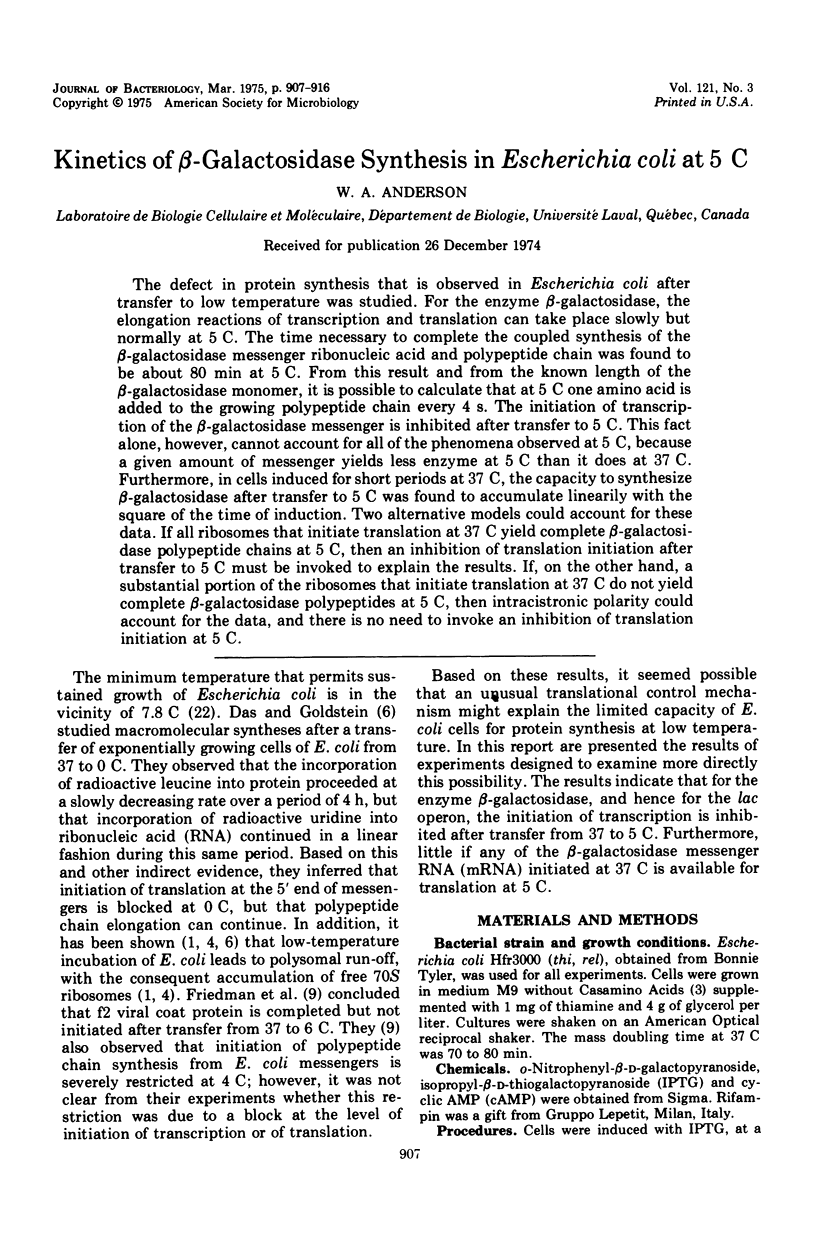
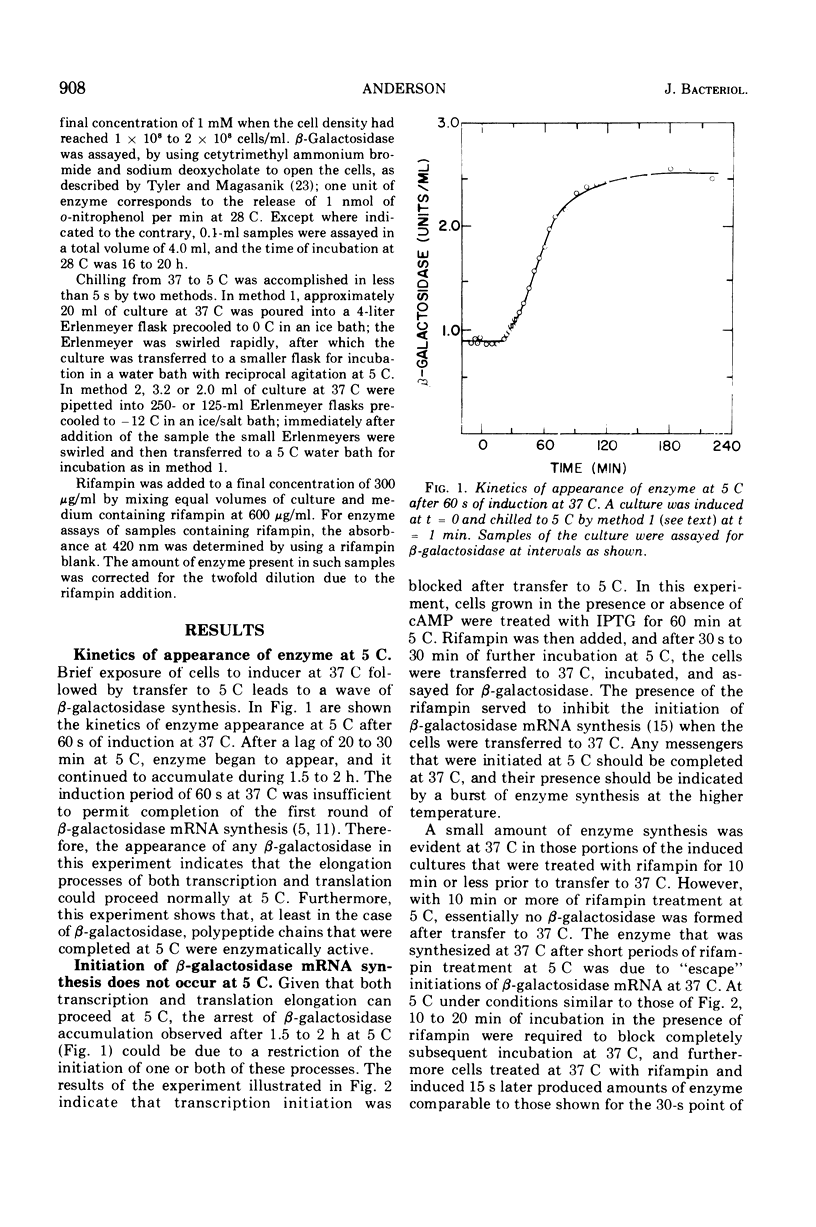
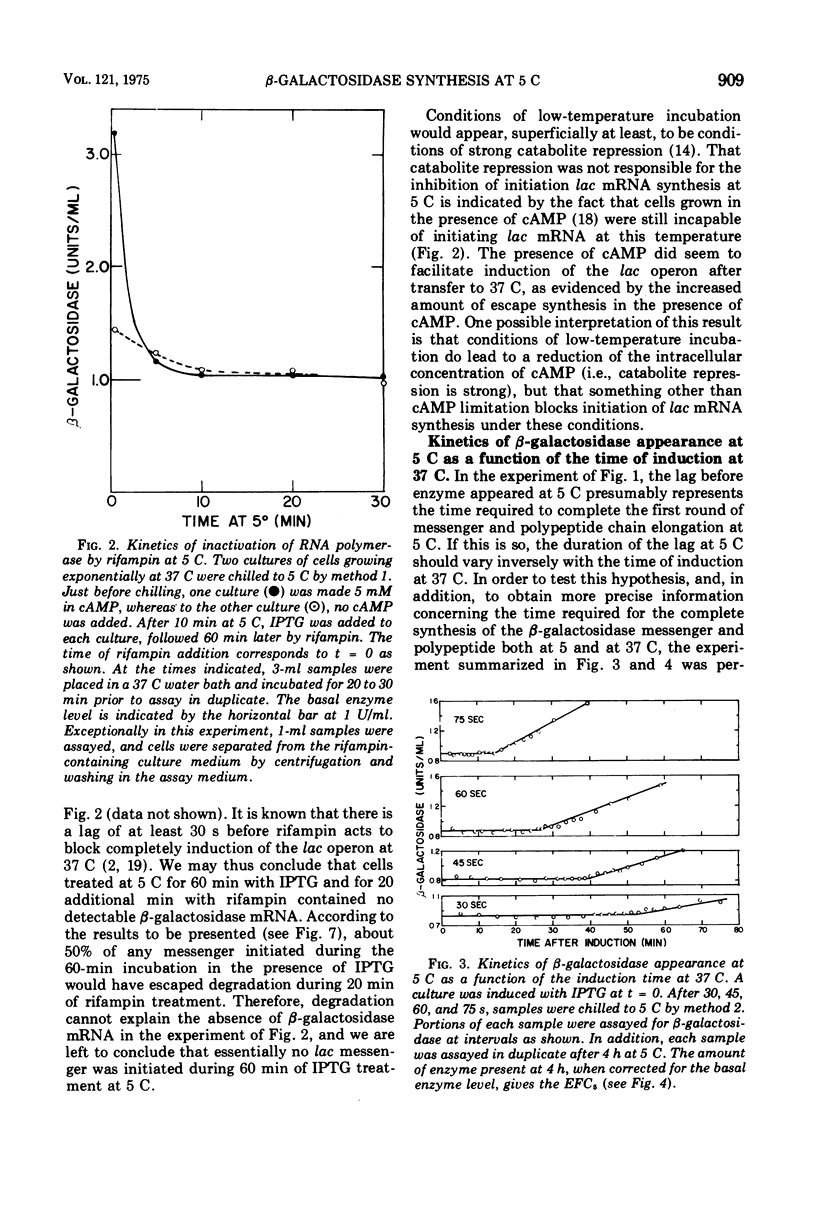
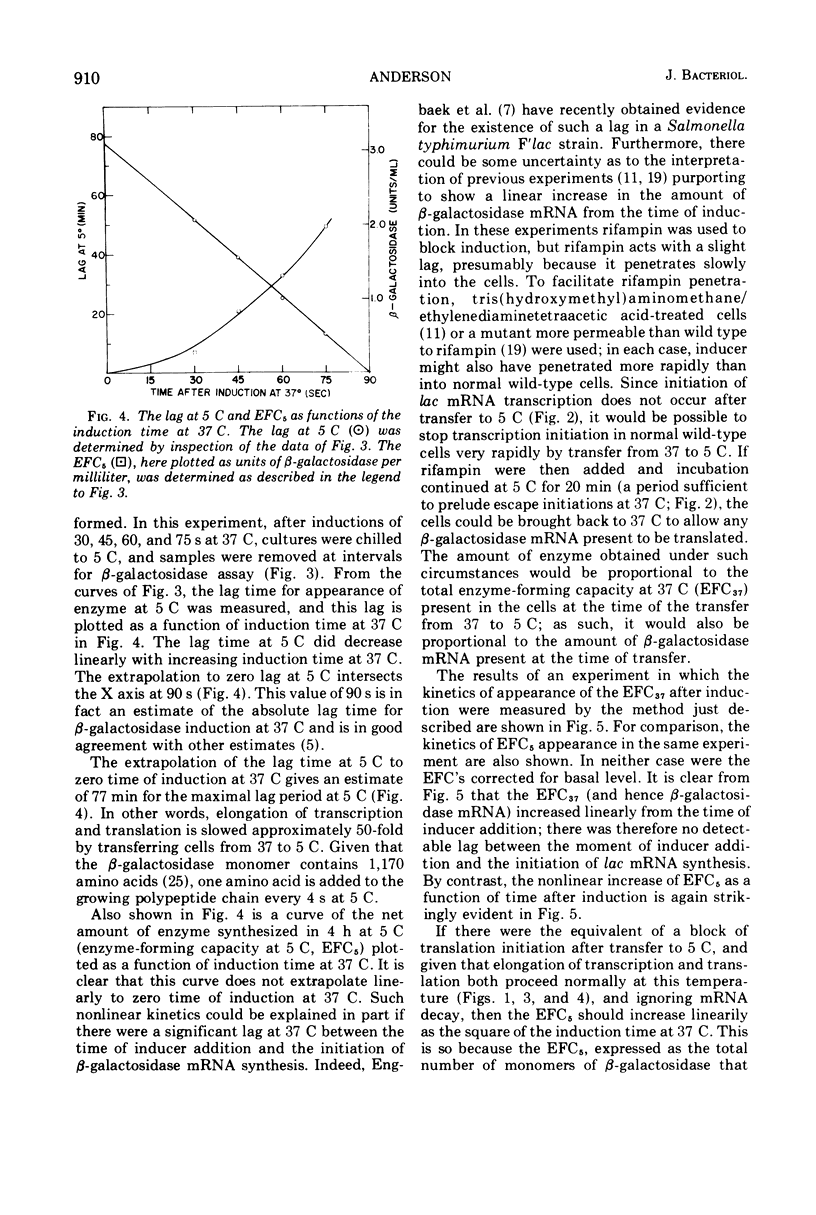
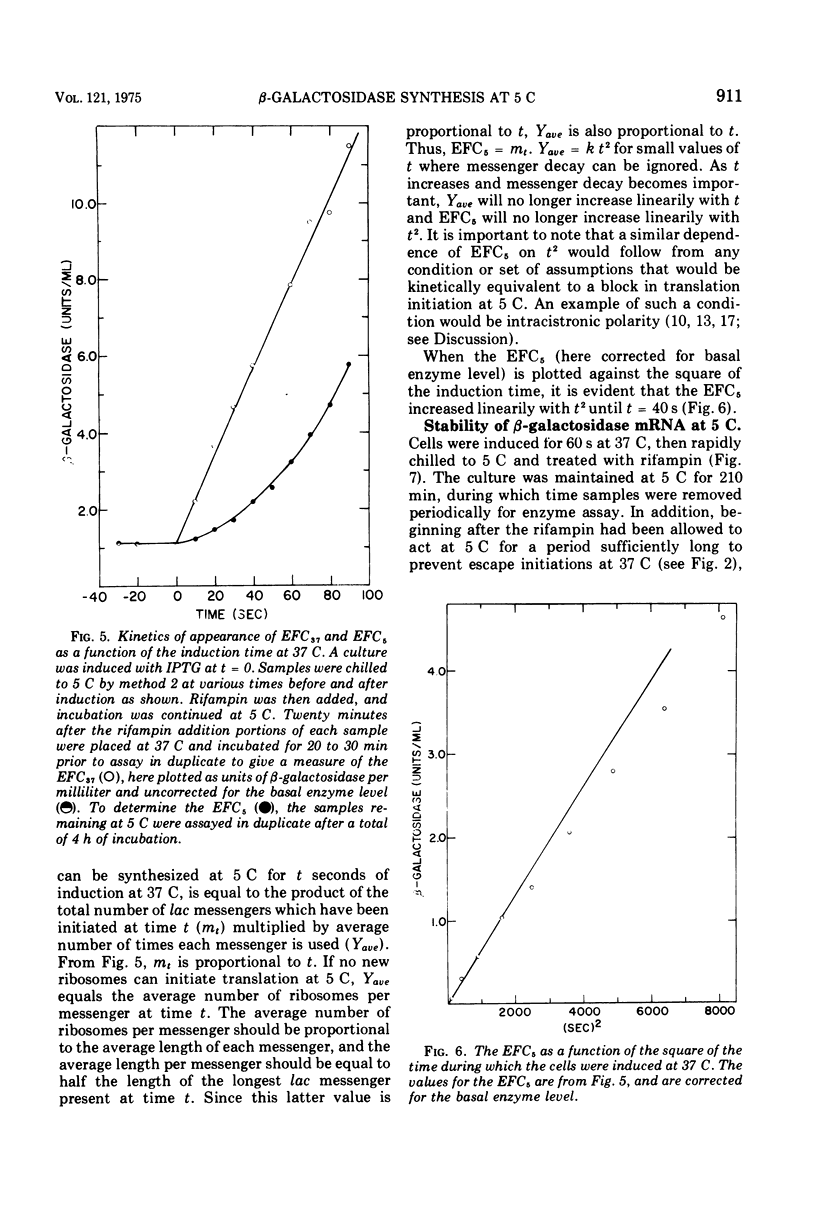
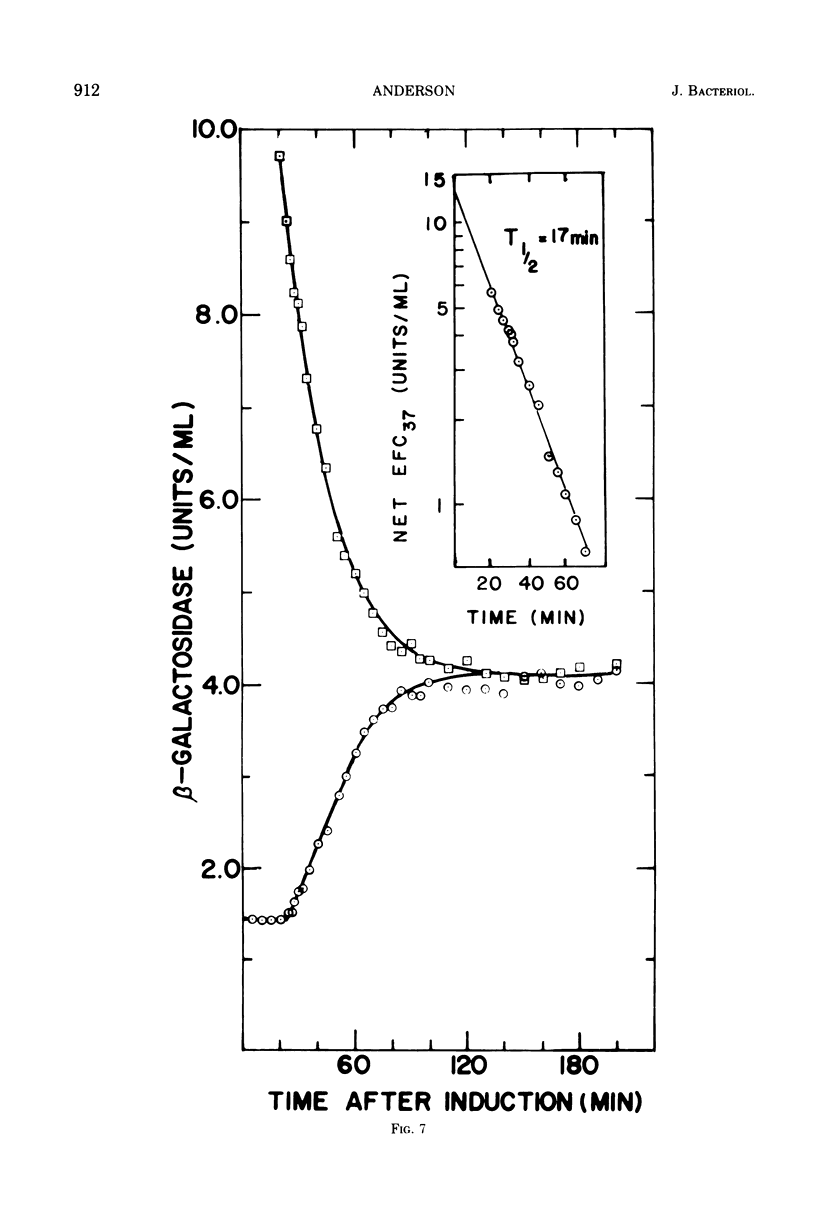
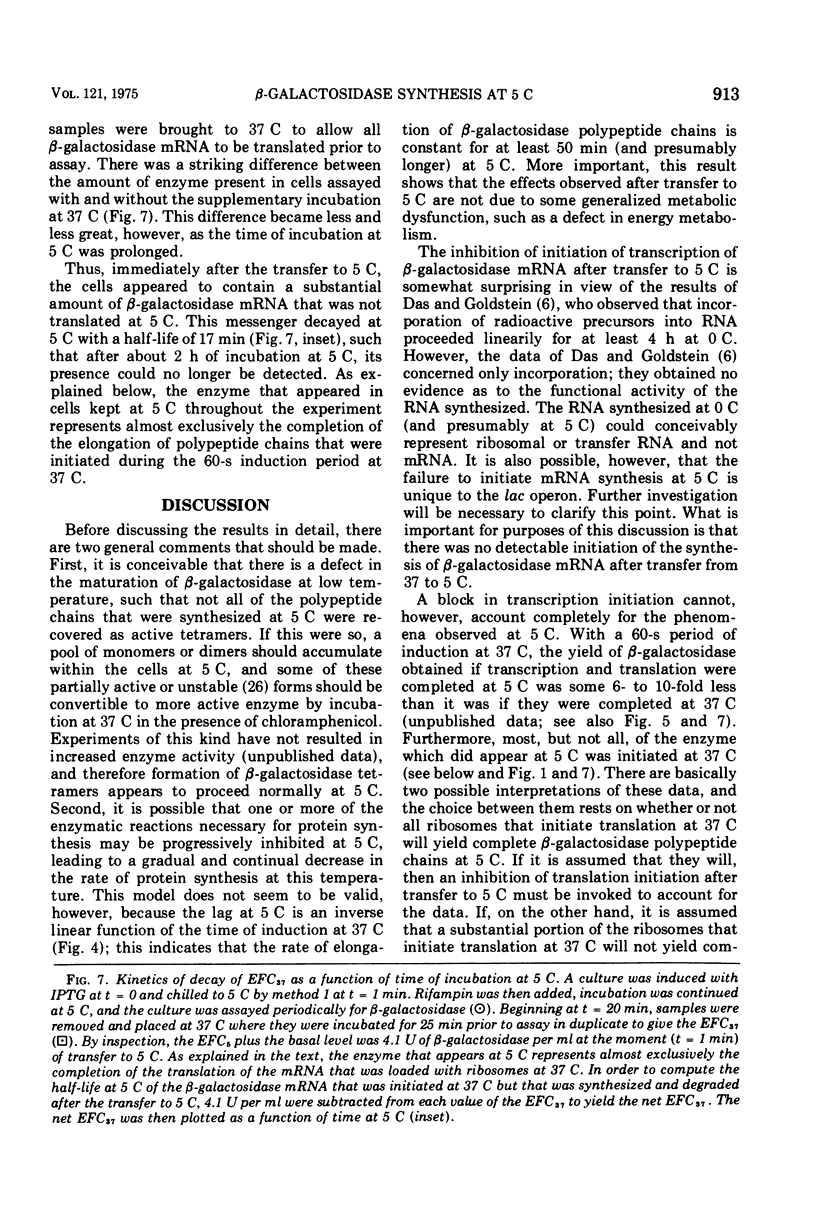
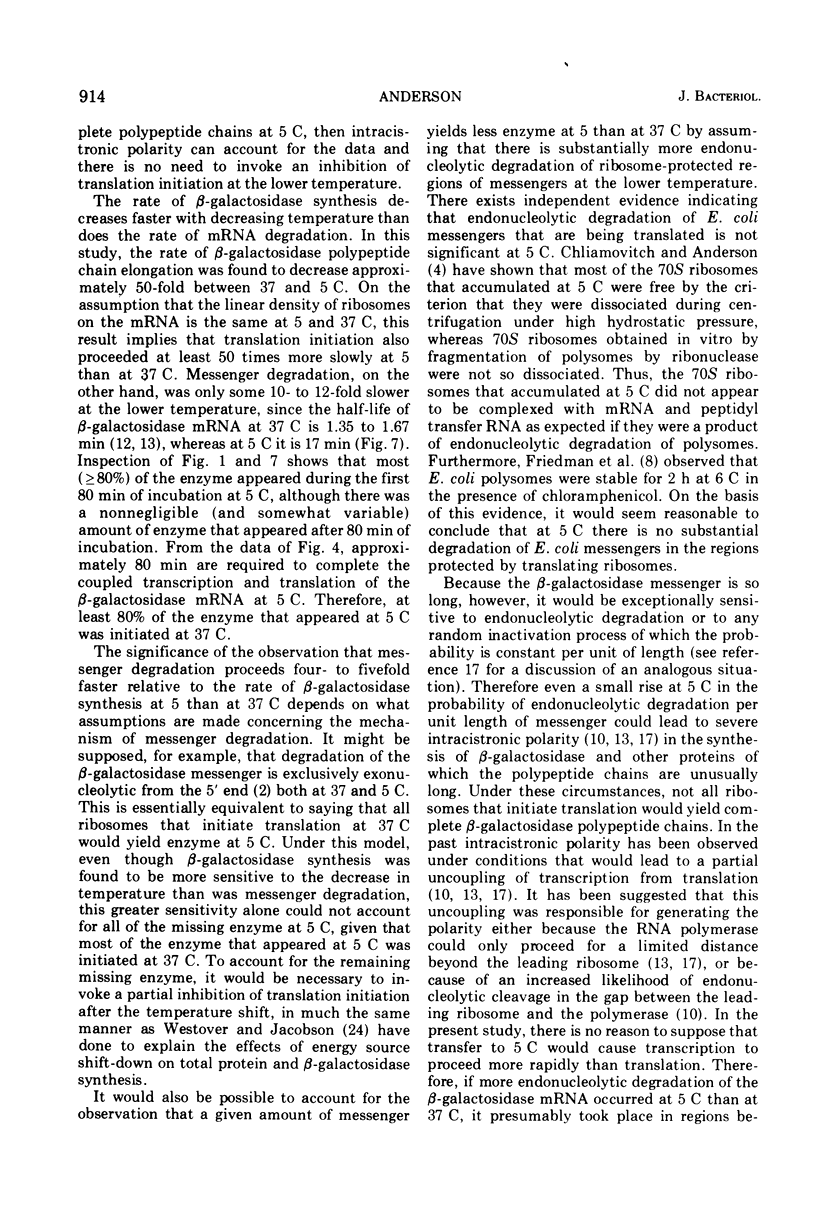
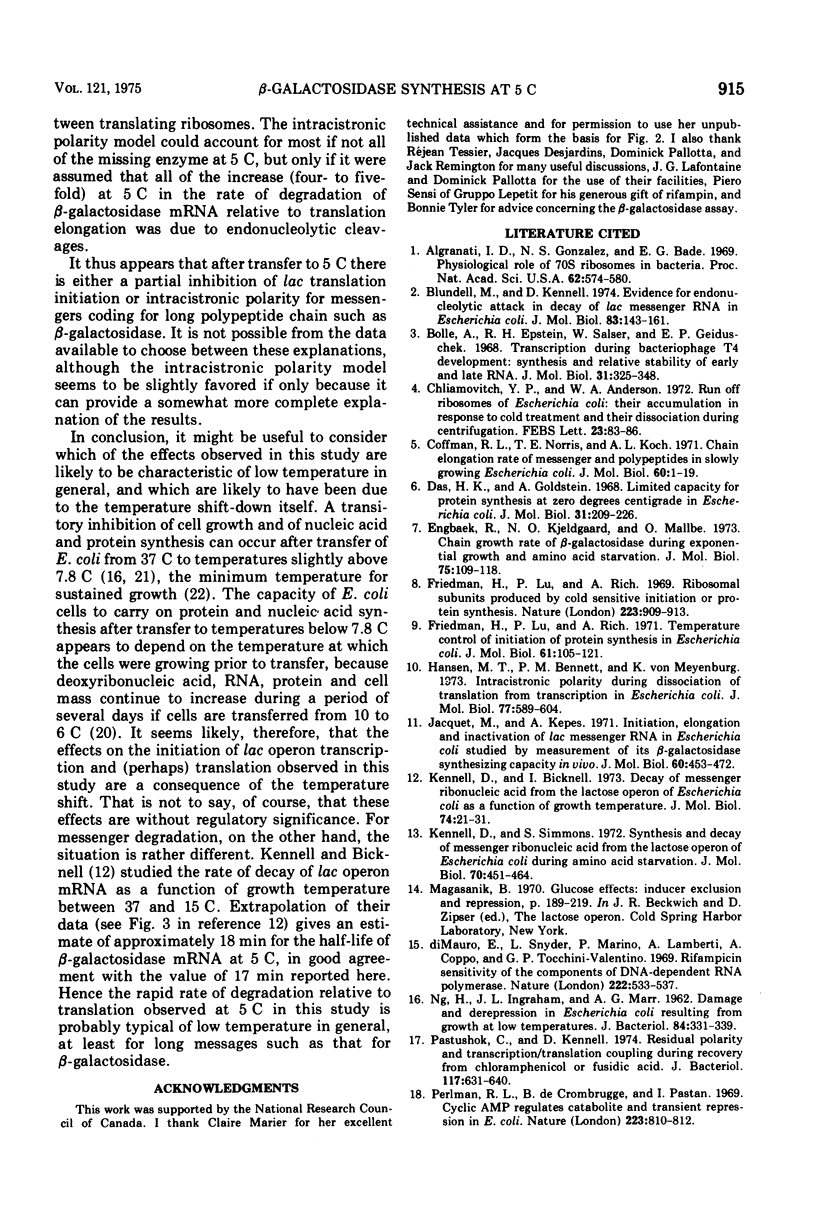
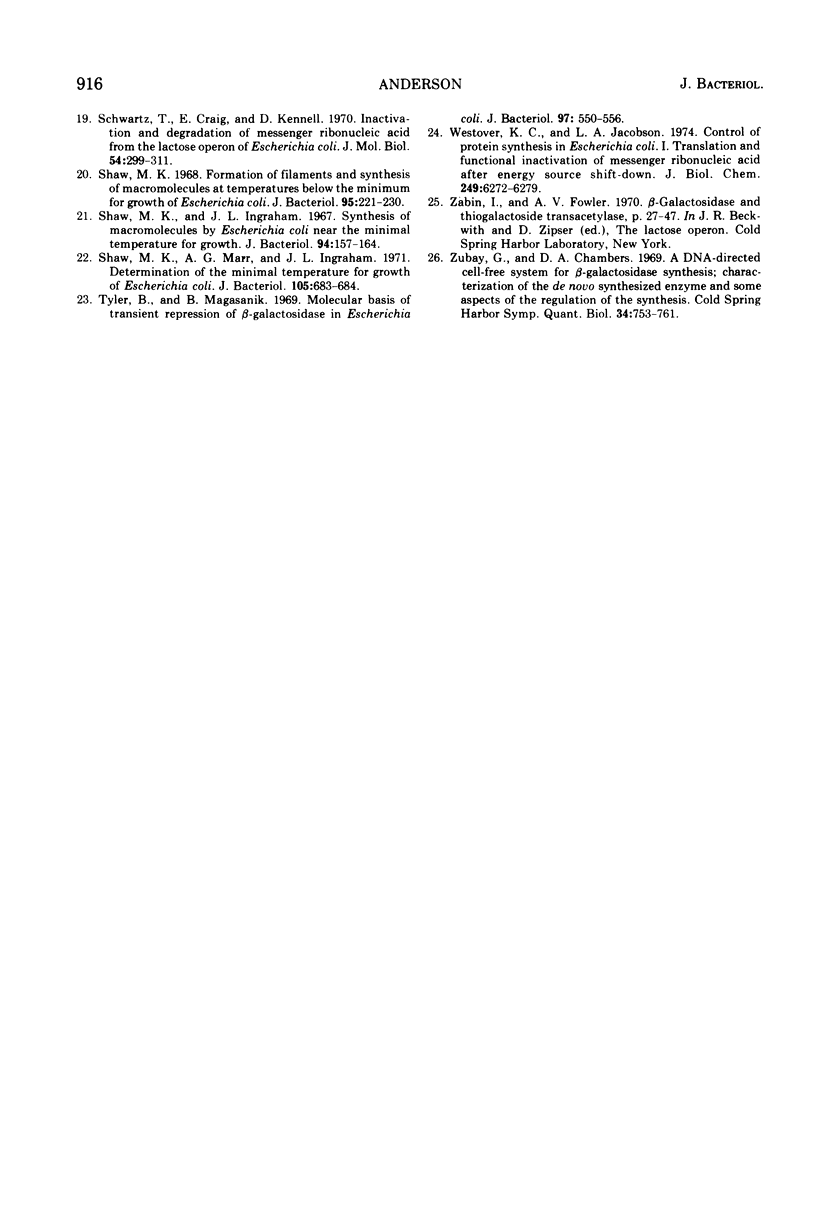
Selected References
These references are in PubMed. This may not be the complete list of references from this article.
- Algranati I. D., Gonzalez N. S., Bade E. G. Physiological role of 70S ribosomes in bacteria. Proc Natl Acad Sci U S A. 1969 Feb;62(2):574–580. doi: 10.1073/pnas.62.2.574. [DOI] [PMC free article] [PubMed] [Google Scholar]
- Blundell M., Kennell D. Evidence for endonucleolytic attack in decay of lac messenger RNA in Escherichia coli. J Mol Biol. 1974 Feb 25;83(2):143–161. doi: 10.1016/0022-2836(74)90385-4. [DOI] [PubMed] [Google Scholar]
- Bolle A., Epstein R. H., Salser W., Geiduschek E. P. Transcription during bacteriophage T4 development: synthesis and relative stability of early and late RNA. J Mol Biol. 1968 Feb 14;31(3):325–348. doi: 10.1016/0022-2836(68)90413-0. [DOI] [PubMed] [Google Scholar]
- Chliamovitch Y. P., Anderson W. A. Run off ribosomes of Escherichia coli: their accumulation in response to cold treatment and their dissociation during centrifugation. FEBS Lett. 1972 Jun 1;23(1):83–86. doi: 10.1016/0014-5793(72)80290-4. [DOI] [PubMed] [Google Scholar]
- Coffman R. L., Norris T. E., Koch A. L. Chain elongation rate of messenger and polypeptides in slowly growing Escherichia coli. J Mol Biol. 1971 Aug 28;60(1):1–19. doi: 10.1016/0022-2836(71)90442-6. [DOI] [PubMed] [Google Scholar]
- Das H. K., Goldstein A. Limited capacity for protein synthesis at zero degrees centigrade in Escherichia coli. J Mol Biol. 1968 Jan 28;31(2):209–226. doi: 10.1016/0022-2836(68)90440-3. [DOI] [PubMed] [Google Scholar]
- Engbaek F., Kjeldgaard N. O., Maaloe O. Chain growth rate of -galactosidase during exponential growth and amino acid starvation. J Mol Biol. 1973 Mar 25;75(1):109–118. doi: 10.1016/0022-2836(73)90532-9. [DOI] [PubMed] [Google Scholar]
- Friedman H., Lu P., Rich A. Ribosomal subunits produced by cold sensitive initiation of protein synthesis. Nature. 1969 Aug 30;223(5209):909–913. doi: 10.1038/223909a0. [DOI] [PubMed] [Google Scholar]
- Friedman H., Lu P., Rich A. Temperature control of initiation of protein synthesis in Escherichia coli. J Mol Biol. 1971 Oct 14;61(1):105–121. doi: 10.1016/0022-2836(71)90209-9. [DOI] [PubMed] [Google Scholar]
- Hansen M. T., Bennett P. M., von Meyenburg K. Intracistronic polarity during dissociation of translation from transcription in Escherichia coli. J Mol Biol. 1973 Jul 15;77(4):589–604. doi: 10.1016/0022-2836(73)90225-8. [DOI] [PubMed] [Google Scholar]
- Jacquet M., Kepes A. Initiation, elongation and inactivation of lac messenger RNA in Escherichia coli studied studied by measurement of its beta-galactosidase synthesizing capacity in vivo. J Mol Biol. 1971 Sep 28;60(3):453–472. doi: 10.1016/0022-2836(71)90181-1. [DOI] [PubMed] [Google Scholar]
- Kennell D., Bicknell I. Decay of messenger ribonucleic acid from the lactose operon of Escherichia coli as a function of growth temperature. J Mol Biol. 1973 Feb 15;74(1):21–31. doi: 10.1016/0022-2836(73)90351-3. [DOI] [PubMed] [Google Scholar]
- Kennell D., Simmons C. Synthesis and decay of messenger ribonucleic acid from the lactose operon of Escherichia coli during amino-acid starvation. J Mol Biol. 1972 Oct 14;70(3):451–464. doi: 10.1016/0022-2836(72)90552-9. [DOI] [PubMed] [Google Scholar]
- NG H., INGRAHAM J. L., MARR A. G. Damage and derepression in Escherichia coli resulting from growth at low temperatures. J Bacteriol. 1962 Aug;84:331–339. doi: 10.1128/jb.84.2.331-339.1962. [DOI] [PMC free article] [PubMed] [Google Scholar]
- Pastushok C., Kennell D. Residual polarity and transcription-translation coupling during recovery from chloramphenicol or fusidic acid. J Bacteriol. 1974 Feb;117(2):631–640. doi: 10.1128/jb.117.2.631-640.1974. [DOI] [PMC free article] [PubMed] [Google Scholar]
- Perlman R. L., De Crombrugghe B., Pastan I. Cyclic AMP regulates catabolite and transient repression in E. coli. Nature. 1969 Aug 23;223(5208):810–812. doi: 10.1038/223810a0. [DOI] [PubMed] [Google Scholar]
- Schwartz T., Craig E., Kennell D. Inactivation and degradation of messenger ribnucleic acid from the lactose operon of Escherichia coli. J Mol Biol. 1970 Dec 14;54(2):299–311. doi: 10.1016/0022-2836(70)90431-6. [DOI] [PubMed] [Google Scholar]
- Shaw M. K. Formation of filaments and synthesis of macromolecules at temperatures below the minimum for growth of Escherichia coli. J Bacteriol. 1968 Jan;95(1):221–230. doi: 10.1128/jb.95.1.221-230.1968. [DOI] [PMC free article] [PubMed] [Google Scholar]
- Shaw M. K., Ingraham J. L. Synthesis of macromolecules by Escherichia coli near the minimal temperature for growth. J Bacteriol. 1967 Jul;94(1):157–164. doi: 10.1128/jb.94.1.157-164.1967. [DOI] [PMC free article] [PubMed] [Google Scholar]
- Shaw M. K., Marr A. G., Ingraham J. L. Determination of the minimal temperature for growth of Escherichia coli. J Bacteriol. 1971 Feb;105(2):683–684. doi: 10.1128/jb.105.2.683-684.1971. [DOI] [PMC free article] [PubMed] [Google Scholar]
- Tyler B., Magasanik B. Molecular basis of transient repression of beta-galactosidase in Escherichia coli. J Bacteriol. 1969 Feb;97(2):550–556. doi: 10.1128/jb.97.2.550-556.1969. [DOI] [PMC free article] [PubMed] [Google Scholar]
- Westover K. C., Jacobson L. A. Control of protein synthesis of Escherichia coli. I. Translation and functional inactivation of messenger ribonucleic acid after energy source shift-down. J Biol Chem. 1974 Oct 10;249(19):6272–6279. [PubMed] [Google Scholar]
- Zubay G., Chambers D. A. A DNA-directed cell-free system for beta-galactosidase synthesis; characterization of the de novo synthesized enzyme and some aspects of the regulation of synthesis. Cold Spring Harb Symp Quant Biol. 1969;34:753–761. doi: 10.1101/sqb.1969.034.01.085. [DOI] [PubMed] [Google Scholar]
- di Mauro E., Synder L., Marino P., Lamberti A., Coppo A., Tocchini-Valentini G. P. Rifampicin sensitivity of the components of DNA-dependent RNA polymerase. Nature. 1969 May 10;222(5193):533–537. doi: 10.1038/222533a0. [DOI] [PubMed] [Google Scholar]