Abstract
We have further characterized and determined the origins of a family of nuclear phosphoproteins of 84, 50, 43, and 34 kilodaltons (kDa) encoded by a class of early transcripts arising from the adjacent EcoRI fragments R and d (map units 0.682 to 0.713) of the strain AD169 human cytomegalovirus genome. These RNAs have a complex spliced structure with common 5' and internal exons and alternative 3' exons with coterminal 3' ends. At early times, two fully processed species of 2.1 and 2.2 kilobases (kb) predominated. As the infection progressed to late times, there was a decrease in splicing of the RNA, generating larger transcripts 2.5 to 2.65 kb in size, which corresponded to the species which had spliced out only the first intron, as well as the completely unspliced transcript. We previously reported that the 34-kDa protein could be derived from a transcript which had failed to splice out the first intron (D. A. Wright, S. I. Staprans, and D. H. Spector, J. Virol. 62:331-340, 1988), but the origin of the other proteins was unclear. cDNA cloning has shown that the 2.1-, 2.2-, and 2.5-kb RNAs encode the 50-, 43-, and 84-kDa proteins, respectively. The shift in the splicing pattern of these RNAs with time revealed a posttranscriptional control mechanism which results in the differential accumulation of individual proteins within this family of nuclear phosphoproteins. Expression of the 84-, 43-, and 34-kDa proteins correlated well with the steady-state concentrations of their respective mRNAs. The 50-kDa protein, however, was not expressed in abundance until late times, despite the presence of the 2.1-kb mRNA in the cytoplasm at early times, suggesting a secondary level of posttranscriptional regulation for this protein. Full expression of the RNAs and proteins was dependent on continuing viral DNA synthesis. Accumulation of the 50-kDa protein was found to be particularly sensitive to the state of viral DNA replication and could not be detected after inhibition of replication. Further analysis of these proteins revealed that each one had a unique pattern of serine phosphorylation. Although there was one common site of phosphorylation, most likely located within the amino-terminal shared region, even this site showed quantitative differences in the level of phosphorylation for each of the proteins. Analysis of the Towne strain and two recent independent clinical isolates of human cytomegalovirus has shown that this family of proteins is highly conserved among human cytomegaloviruses.
Full text
PDF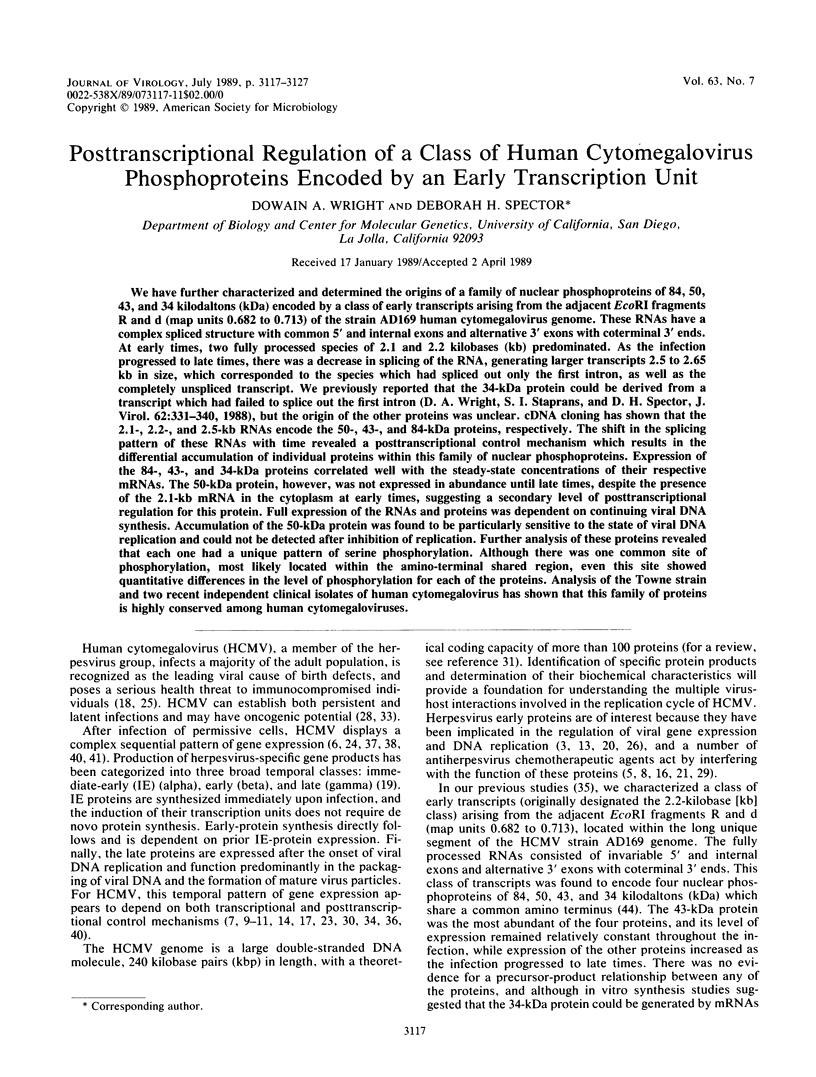
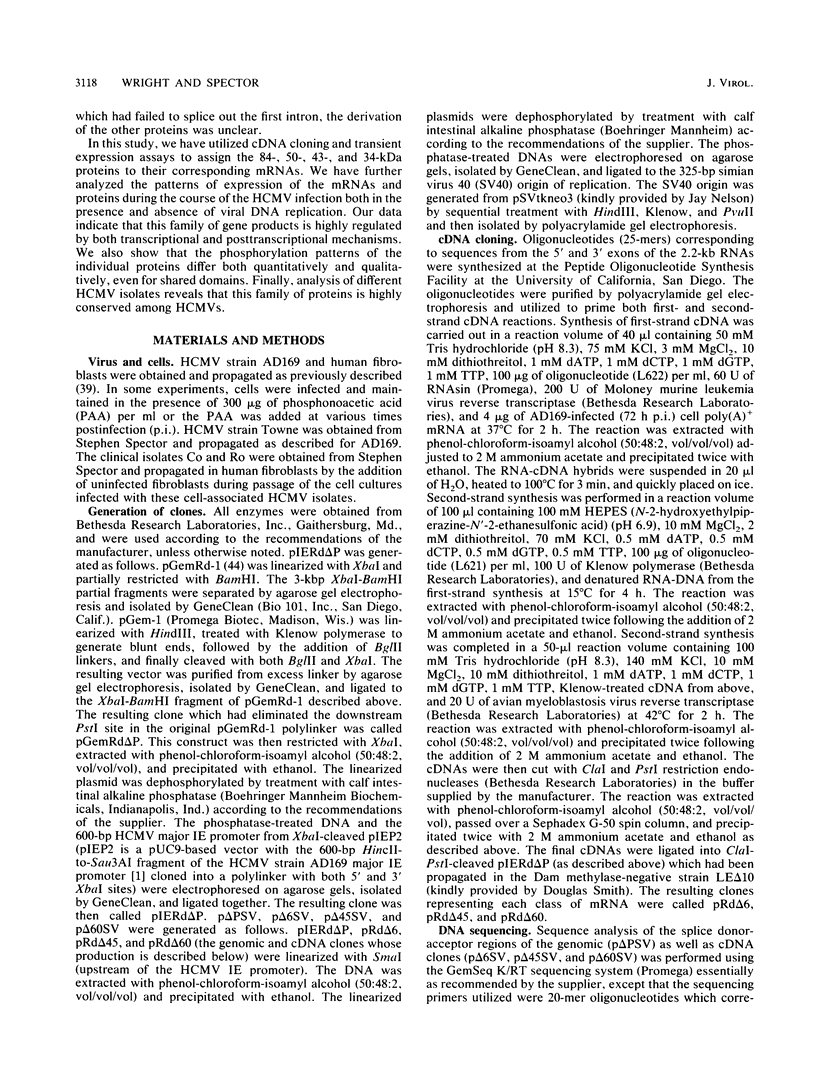
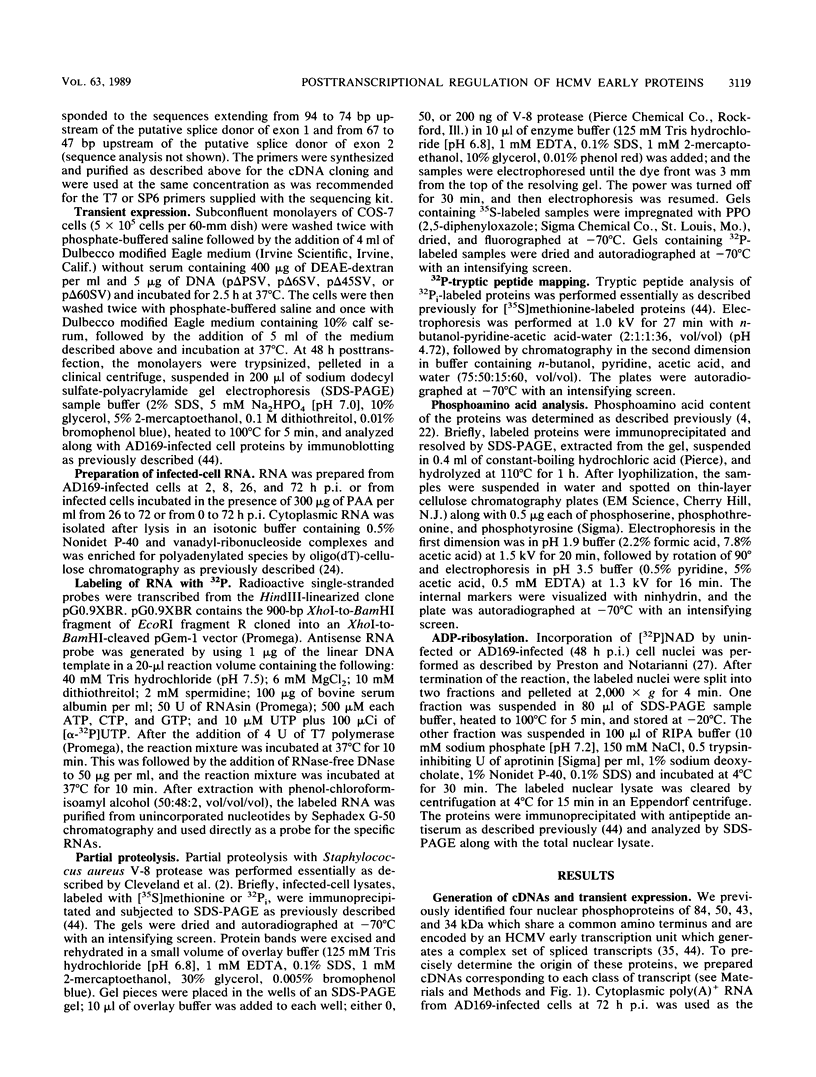
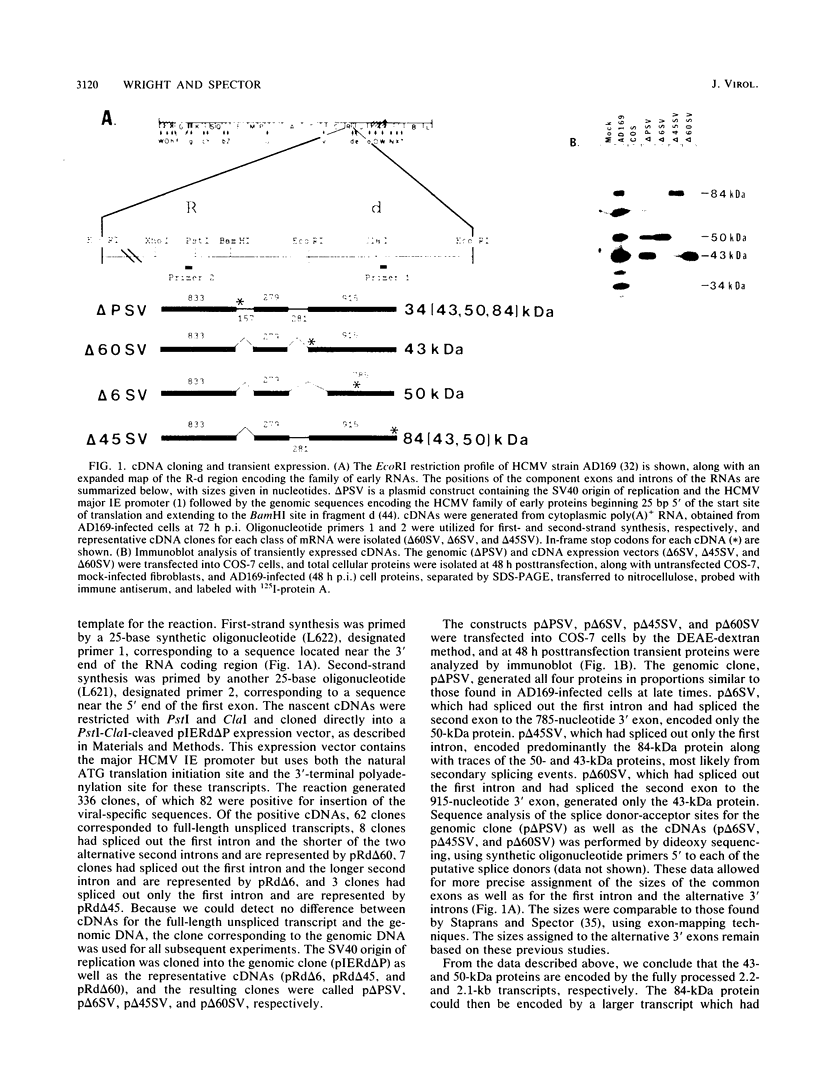
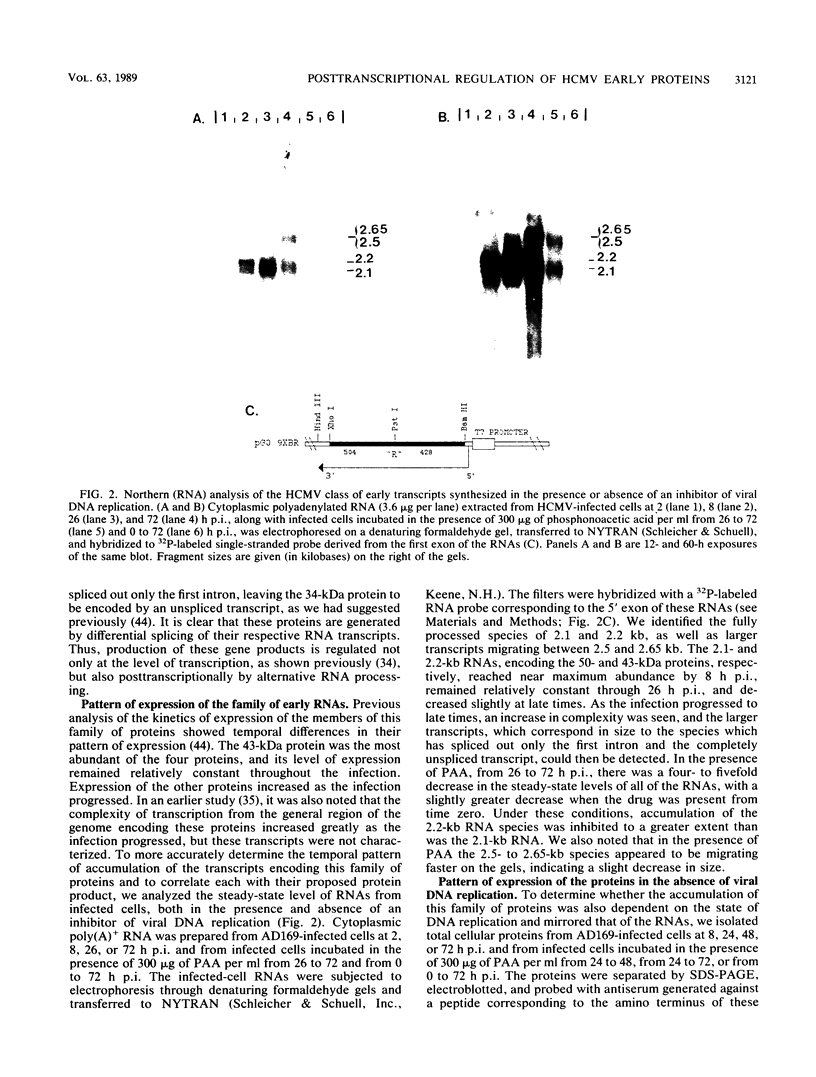
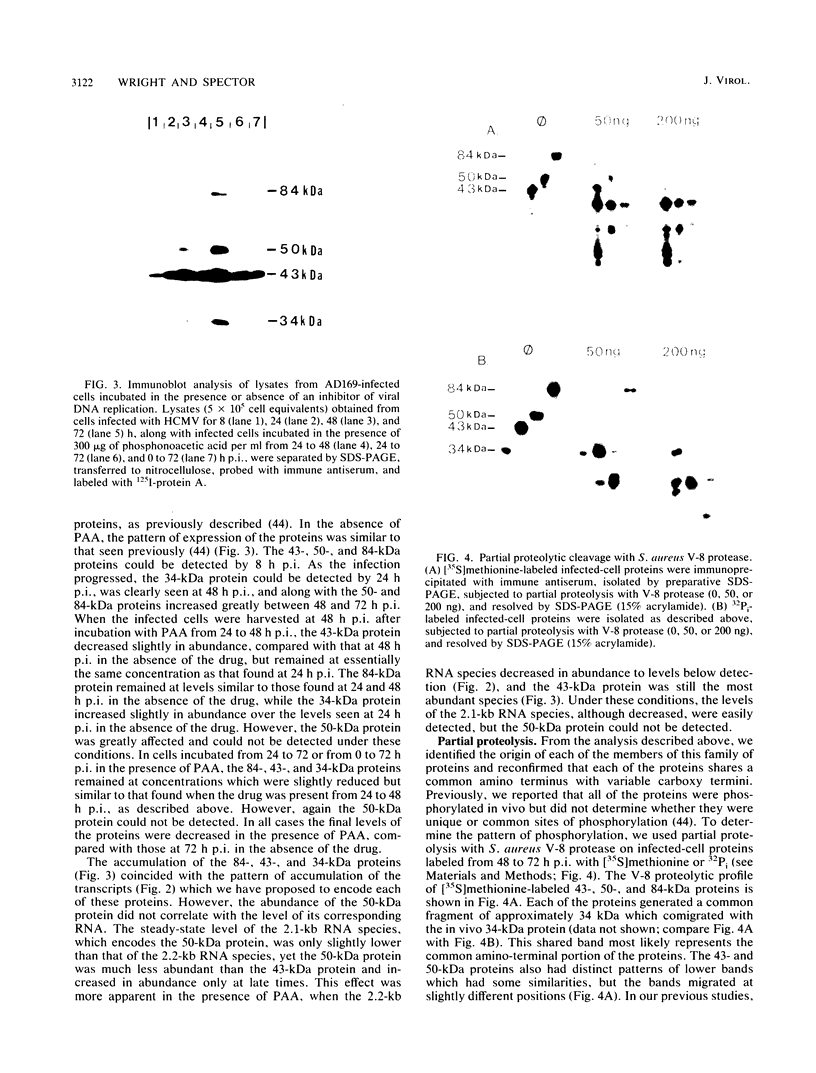
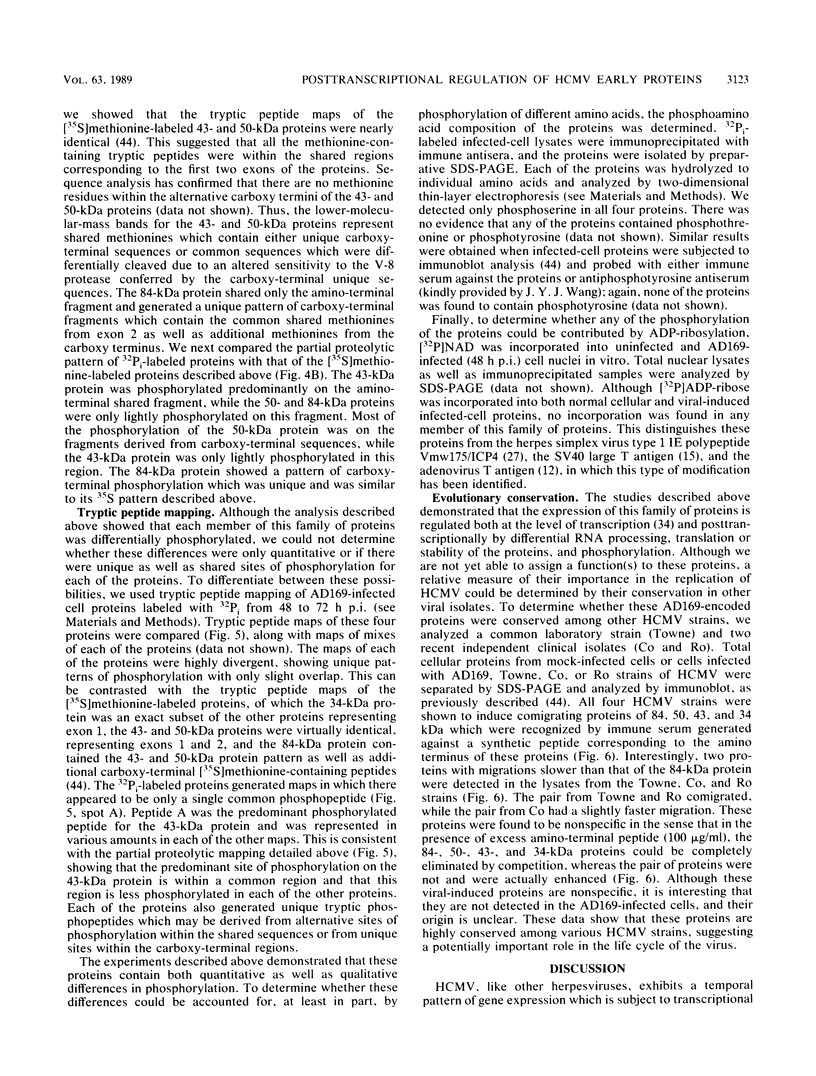
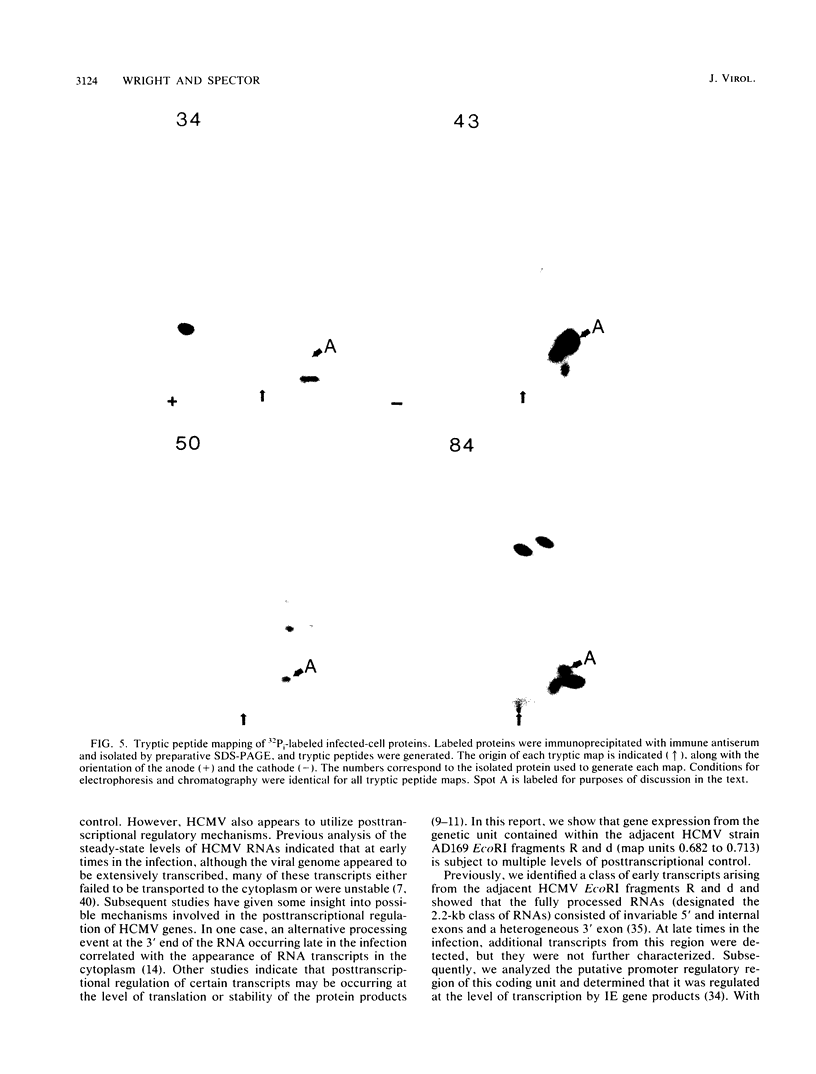
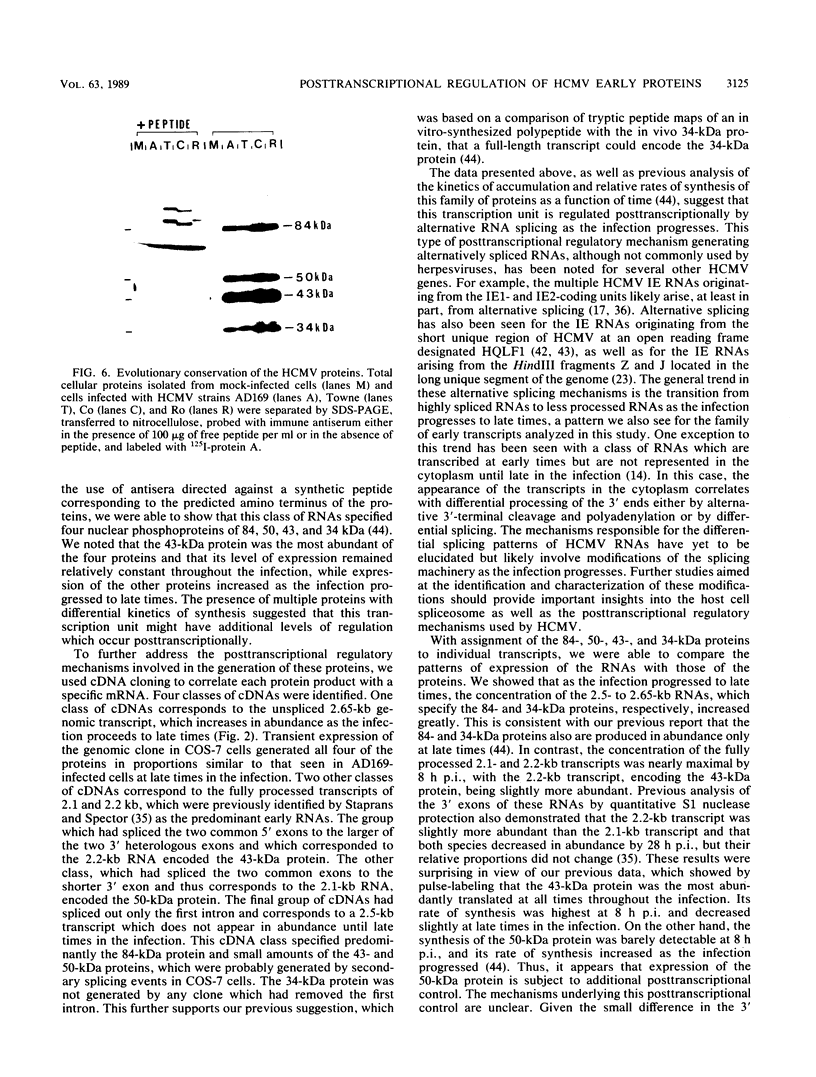
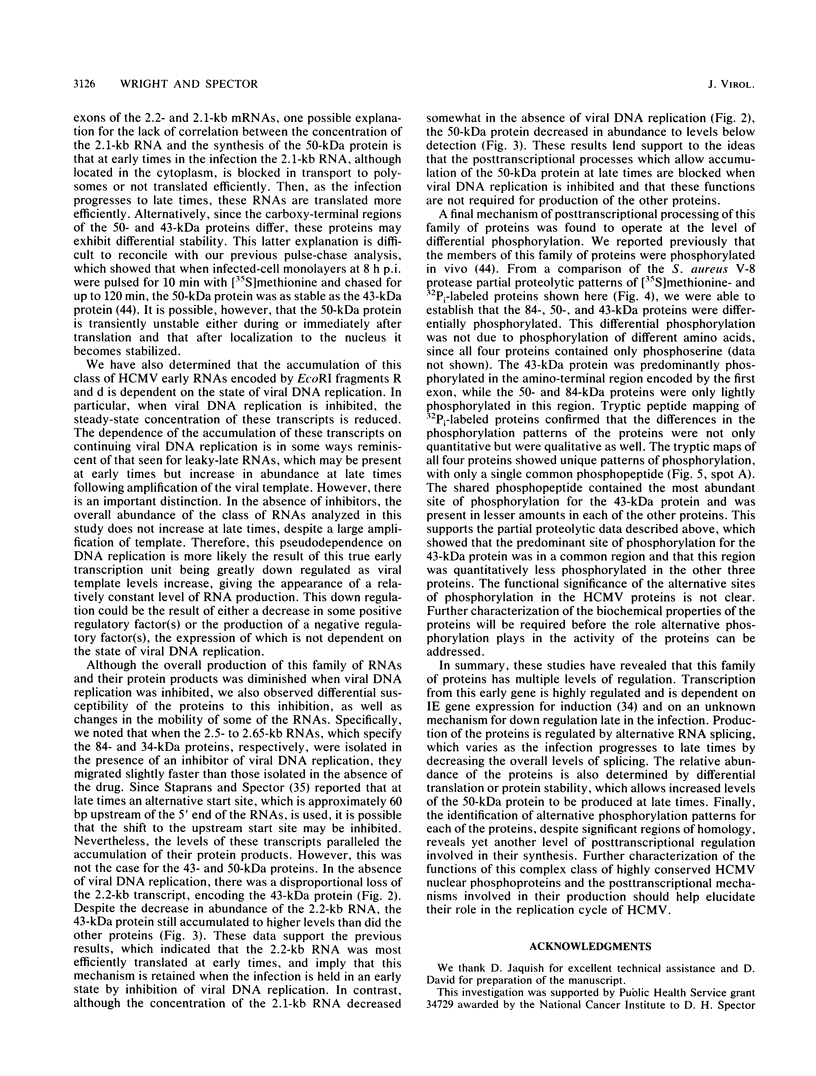
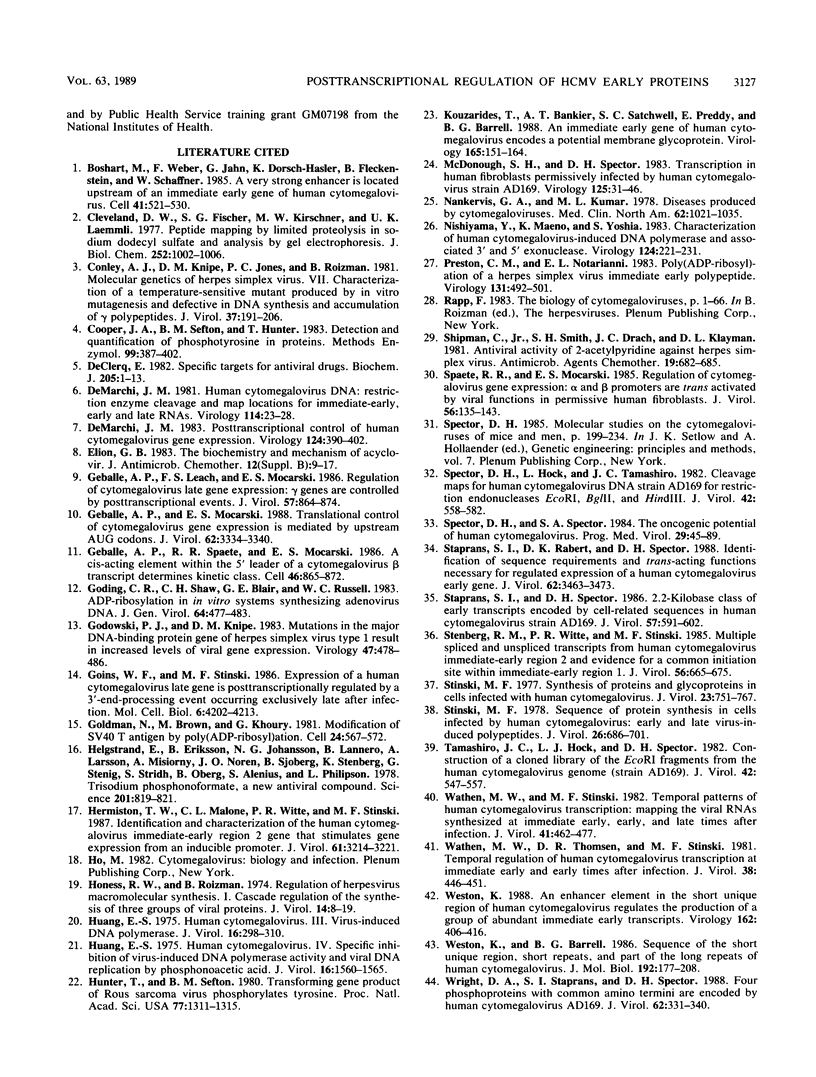
Images in this article
Selected References
These references are in PubMed. This may not be the complete list of references from this article.
- Boshart M., Weber F., Jahn G., Dorsch-Häsler K., Fleckenstein B., Schaffner W. A very strong enhancer is located upstream of an immediate early gene of human cytomegalovirus. Cell. 1985 Jun;41(2):521–530. doi: 10.1016/s0092-8674(85)80025-8. [DOI] [PubMed] [Google Scholar]
- Conley A. J., Knipe D. M., Jones P. C., Roizman B. Molecular genetics of herpes simplex virus. VII. Characterization of a temperature-sensitive mutant produced by in vitro mutagenesis and defective in DNA synthesis and accumulation of gamma polypeptides. J Virol. 1981 Jan;37(1):191–206. doi: 10.1128/jvi.37.1.191-206.1981. [DOI] [PMC free article] [PubMed] [Google Scholar]
- Cooper J. A., Sefton B. M., Hunter T. Detection and quantification of phosphotyrosine in proteins. Methods Enzymol. 1983;99:387–402. doi: 10.1016/0076-6879(83)99075-4. [DOI] [PubMed] [Google Scholar]
- De Clercq E. Specific targets for antiviral drugs. Biochem J. 1982 Jul 1;205(1):1–13. doi: 10.1042/bj2050001. [DOI] [PMC free article] [PubMed] [Google Scholar]
- DeMarchi J. M. Post-transcriptional control of human cytomegalovirus gene expression. Virology. 1983 Jan 30;124(2):390–402. doi: 10.1016/0042-6822(83)90355-0. [DOI] [PubMed] [Google Scholar]
- Demarchi J. M. Human cytomegalovirus DNA: restriction enzyme cleavage maps and map locations for immediate-early, early, and late RNAs. Virology. 1981 Oct 15;114(1):23–38. doi: 10.1016/0042-6822(81)90249-x. [DOI] [PubMed] [Google Scholar]
- Elion G. B. The biochemistry and mechanism of action of acyclovir. J Antimicrob Chemother. 1983 Sep;12 (Suppl B):9–17. doi: 10.1093/jac/12.suppl_b.9. [DOI] [PubMed] [Google Scholar]
- Geballe A. P., Leach F. S., Mocarski E. S. Regulation of cytomegalovirus late gene expression: gamma genes are controlled by posttranscriptional events. J Virol. 1986 Mar;57(3):864–874. doi: 10.1128/jvi.57.3.864-874.1986. [DOI] [PMC free article] [PubMed] [Google Scholar]
- Geballe A. P., Mocarski E. S. Translational control of cytomegalovirus gene expression is mediated by upstream AUG codons. J Virol. 1988 Sep;62(9):3334–3340. doi: 10.1128/jvi.62.9.3334-3340.1988. [DOI] [PMC free article] [PubMed] [Google Scholar]
- Geballe A. P., Spaete R. R., Mocarski E. S. A cis-acting element within the 5' leader of a cytomegalovirus beta transcript determines kinetic class. Cell. 1986 Sep 12;46(6):865–872. doi: 10.1016/0092-8674(86)90068-1. [DOI] [PubMed] [Google Scholar]
- Goding C. R., Shaw C. H., Blair G. E., Russell W. C. ADP-ribosylation in in vitro systems synthesizing adenovirus DNA. J Gen Virol. 1983 Feb;64(Pt 2):477–483. doi: 10.1099/0022-1317-64-2-477. [DOI] [PubMed] [Google Scholar]
- Godowski P. J., Knipe D. M. Mutations in the major DNA-binding protein gene of herpes simplex virus type 1 result in increased levels of viral gene expression. J Virol. 1983 Sep;47(3):478–486. doi: 10.1128/jvi.47.3.478-486.1983. [DOI] [PMC free article] [PubMed] [Google Scholar]
- Goins W. F., Stinski M. F. Expression of a human cytomegalovirus late gene is posttranscriptionally regulated by a 3'-end-processing event occurring exclusively late after infection. Mol Cell Biol. 1986 Dec;6(12):4202–4213. doi: 10.1128/mcb.6.12.4202. [DOI] [PMC free article] [PubMed] [Google Scholar]
- Goldman N., Brown M., Khoury G. Modification of SV40 T antigen by poly ADP-ribosylation. Cell. 1981 May;24(2):567–572. doi: 10.1016/0092-8674(81)90347-0. [DOI] [PubMed] [Google Scholar]
- Helgstrand E., Eriksson B., Johansson N. G., Lannerö B., Larsson A., Misiorny A., Norén J. O., Sjöberg B., Stenberg K., Stening G. Trisodium phosphonoformate, a new antiviral compound. Science. 1978 Sep 1;201(4358):819–821. doi: 10.1126/science.210500. [DOI] [PubMed] [Google Scholar]
- Hermiston T. W., Malone C. L., Witte P. R., Stinski M. F. Identification and characterization of the human cytomegalovirus immediate-early region 2 gene that stimulates gene expression from an inducible promoter. J Virol. 1987 Oct;61(10):3214–3221. doi: 10.1128/jvi.61.10.3214-3221.1987. [DOI] [PMC free article] [PubMed] [Google Scholar]
- Honess R. W., Roizman B. Regulation of herpesvirus macromolecular synthesis. I. Cascade regulation of the synthesis of three groups of viral proteins. J Virol. 1974 Jul;14(1):8–19. doi: 10.1128/jvi.14.1.8-19.1974. [DOI] [PMC free article] [PubMed] [Google Scholar]
- Huang E. S. Human cytomegalovirus. III. Virus-induced DNA polymerase. J Virol. 1975 Aug;16(2):298–310. doi: 10.1128/jvi.16.2.298-310.1975. [DOI] [PMC free article] [PubMed] [Google Scholar]
- Huang E. S. Human cytomegalovirus. IV. Specific inhibition of virus-induced DNA polymerase activity and viral DNA replication by phosphonoacetic acid. J Virol. 1975 Dec;16(6):1560–1565. doi: 10.1128/jvi.16.6.1560-1565.1975. [DOI] [PMC free article] [PubMed] [Google Scholar]
- Hunter T., Sefton B. M. Transforming gene product of Rous sarcoma virus phosphorylates tyrosine. Proc Natl Acad Sci U S A. 1980 Mar;77(3):1311–1315. doi: 10.1073/pnas.77.3.1311. [DOI] [PMC free article] [PubMed] [Google Scholar]
- Kouzarides T., Bankier A. T., Satchwell S. C., Preddy E., Barrell B. G. An immediate early gene of human cytomegalovirus encodes a potential membrane glycoprotein. Virology. 1988 Jul;165(1):151–164. doi: 10.1016/0042-6822(88)90668-x. [DOI] [PubMed] [Google Scholar]
- Lagerkvist U., Akesson B., Brändén R. Aminoacyl adenylate, a normal intermediate or a dead end in aminoacylation of transfer ribonucleic acid. J Biol Chem. 1977 Feb 10;252(3):1002–1006. [PubMed] [Google Scholar]
- McDonough S. H., Spector D. H. Transcription in human fibroblasts permissively infected by human cytomegalovirus strain AD169. Virology. 1983 Feb;125(1):31–46. doi: 10.1016/0042-6822(83)90061-2. [DOI] [PubMed] [Google Scholar]
- Nankervis G. A., Kumar M. L. Diseases produced by cytomegaloviruses. Med Clin North Am. 1978 Sep;62(5):1021–1035. doi: 10.1016/s0025-7125(16)31752-7. [DOI] [PubMed] [Google Scholar]
- Nishiyama Y., Maeno K., Yoshida S. Characterization of human cytomegalovirus-induced DNA polymerase and the associated 3'-to-5', exonuclease. Virology. 1983 Jan 30;124(2):221–231. doi: 10.1016/0042-6822(83)90339-2. [DOI] [PubMed] [Google Scholar]
- Preston C. M., Notarianni E. L. Poly(ADP-ribosyl)ation of a herpes simplex virus immediate early polypeptide. Virology. 1983 Dec;131(2):492–501. doi: 10.1016/0042-6822(83)90515-9. [DOI] [PubMed] [Google Scholar]
- Shipman C., Jr, Smith S. H., Drach J. C., Klayman D. L. Antiviral activity of 2-acetylpyridine thiosemicarbazones against herpes simplex virus. Antimicrob Agents Chemother. 1981 Apr;19(4):682–685. doi: 10.1128/aac.19.4.682. [DOI] [PMC free article] [PubMed] [Google Scholar]
- Spaete R. R., Mocarski E. S. Regulation of cytomegalovirus gene expression: alpha and beta promoters are trans activated by viral functions in permissive human fibroblasts. J Virol. 1985 Oct;56(1):135–143. doi: 10.1128/jvi.56.1.135-143.1985. [DOI] [PMC free article] [PubMed] [Google Scholar]
- Spector D. H., Hock L., Tamashiro J. C. Cleavage maps for human cytomegalovirus DNA strain AD169 for restriction endonucleases EcoRI, BglII, and HindIII. J Virol. 1982 May;42(2):558–582. doi: 10.1128/jvi.42.2.558-582.1982. [DOI] [PMC free article] [PubMed] [Google Scholar]
- Spector D. H., Spector S. A. The oncogenic potential of human cytomegalovirus. Prog Med Virol. 1984;29:45–89. [PubMed] [Google Scholar]
- Staprans S. I., Rabert D. K., Spector D. H. Identification of sequence requirements and trans-acting functions necessary for regulated expression of a human cytomegalovirus early gene. J Virol. 1988 Sep;62(9):3463–3473. doi: 10.1128/jvi.62.9.3463-3473.1988. [DOI] [PMC free article] [PubMed] [Google Scholar]
- Staprans S. I., Spector D. H. 2.2-kilobase class of early transcripts encoded by cell-related sequences in human cytomegalovirus strain AD169. J Virol. 1986 Feb;57(2):591–602. doi: 10.1128/jvi.57.2.591-602.1986. [DOI] [PMC free article] [PubMed] [Google Scholar]
- Stenberg R. M., Witte P. R., Stinski M. F. Multiple spliced and unspliced transcripts from human cytomegalovirus immediate-early region 2 and evidence for a common initiation site within immediate-early region 1. J Virol. 1985 Dec;56(3):665–675. doi: 10.1128/jvi.56.3.665-675.1985. [DOI] [PMC free article] [PubMed] [Google Scholar]
- Stinski M. F. Sequence of protein synthesis in cells infected by human cytomegalovirus: early and late virus-induced polypeptides. J Virol. 1978 Jun;26(3):686–701. doi: 10.1128/jvi.26.3.686-701.1978. [DOI] [PMC free article] [PubMed] [Google Scholar]
- Stinski M. F. Synthesis of proteins and glycoproteins in cells infected with human cytomegalovirus. J Virol. 1977 Sep;23(3):751–767. doi: 10.1128/jvi.23.3.751-767.1977. [DOI] [PMC free article] [PubMed] [Google Scholar]
- Tamashiro J. C., Hock L. J., Spector D. H. Construction of a cloned library of the EcoRI fragments from the human cytomegalovirus genome (strain AD169). J Virol. 1982 May;42(2):547–557. doi: 10.1128/jvi.42.2.547-557.1982. [DOI] [PMC free article] [PubMed] [Google Scholar]
- Wathen M. W., Stinski M. F. Temporal patterns of human cytomegalovirus transcription: mapping the viral RNAs synthesized at immediate early, early, and late times after infection. J Virol. 1982 Feb;41(2):462–477. doi: 10.1128/jvi.41.2.462-477.1982. [DOI] [PMC free article] [PubMed] [Google Scholar]
- Wathen M. W., Thomsen D. R., Stinski M. F. Temporal regulation of human cytomegalovirus transcription at immediate early and early times after infection. J Virol. 1981 May;38(2):446–459. doi: 10.1128/jvi.38.2.446-459.1981. [DOI] [PMC free article] [PubMed] [Google Scholar]
- Weston K. An enhancer element in the short unique region of human cytomegalovirus regulates the production of a group of abundant immediate early transcripts. Virology. 1988 Feb;162(2):406–416. doi: 10.1016/0042-6822(88)90481-3. [DOI] [PubMed] [Google Scholar]
- Weston K., Barrell B. G. Sequence of the short unique region, short repeats, and part of the long repeats of human cytomegalovirus. J Mol Biol. 1986 Nov 20;192(2):177–208. doi: 10.1016/0022-2836(86)90359-1. [DOI] [PubMed] [Google Scholar]
- Wright D. A., Staprans S. I., Spector D. H. Four phosphoproteins with common amino termini are encoded by human cytomegalovirus AD169. J Virol. 1988 Jan;62(1):331–340. doi: 10.1128/jvi.62.1.331-340.1988. [DOI] [PMC free article] [PubMed] [Google Scholar]