Abstract
The distribution of cellular fatty acids in defined lipid classes was analyzed in Micrococcus cerificans after growth on specified hydrocarbons. Neutral lipid, phospholipid, and cell residue fatty acids were qualitatively and quantitatively determined for M. cerificans grown on nutrient broth, tetradecane (C14), pentadecane (C15), hexadecane (C16), and heptadecane (C17), respectively. Percentage of total cellular fatty acid localized in defined lipid classes from cells grown on the above growth substrates was (i) neutral lipid—11.8, 1.81, 7.74, 23.1, and 2%; (ii) phospholipid—74.5, 65, 66.43, 62.1, and 86%; (iii) cell residue lipid—13.5, 33.29, 25.82, 14.78, and 11.9%. Phospholipid fatty acid chain length directly reflected the carbon number of the alkane substrate, with 40, 84, 98, and 77% of the fatty acids being 14, 15, 16, and 17 carbons when cells were grown on C14, C15, C16, and C17n-alkanes, respectively. The bound lipids of the cell residue after chloroform-methanol extraction were characterized by 2-hydroxydodecanoic and 2-hydroxytetradecanoic acids plus a broad spectrum of fatty acids ranging from C10 to C17 chain length. An increase in total unsaturated fatty acid localized in the phospholipids was noted from cells grown on alkanes greater than 15 carbons long. An extracellular accumulation of free fatty acid (FFA) was demonstrated in hexadecane-grown cultures that was not apparent in non-hydrocarbon-grown cultures. Identification of extracellular FFA demonstrated direct derivation from hexadecane oxidation. Studies supporting inhibition of de novo fatty acid biosynthesis in relationship to extracellular FFA and hexadecane oxidation are described. The ability to alter the fatty acid composition of membrane polar lipids in a predictable manner by the alkane carbon source provides an excellent model system for the investigation of membrane structure-function relationships in M. cerificans.
Full text
PDF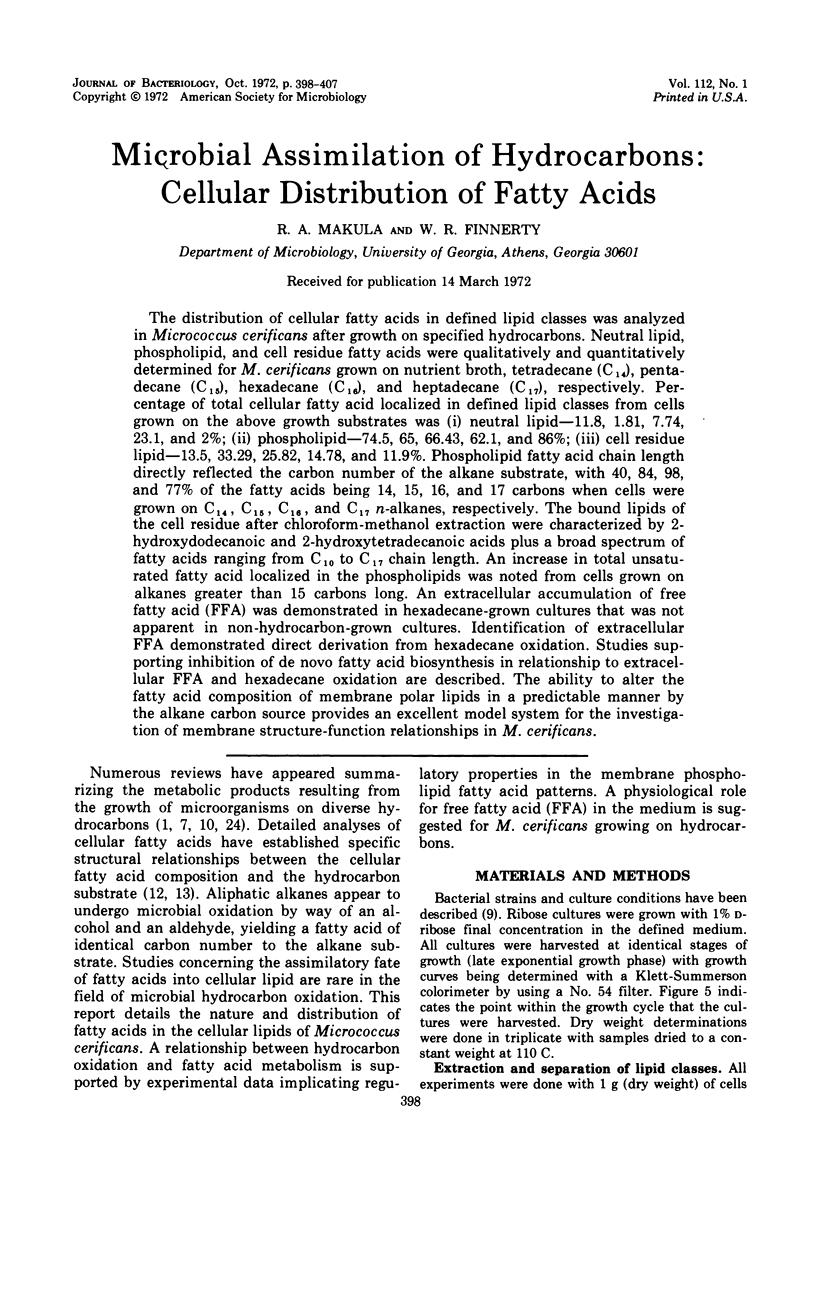
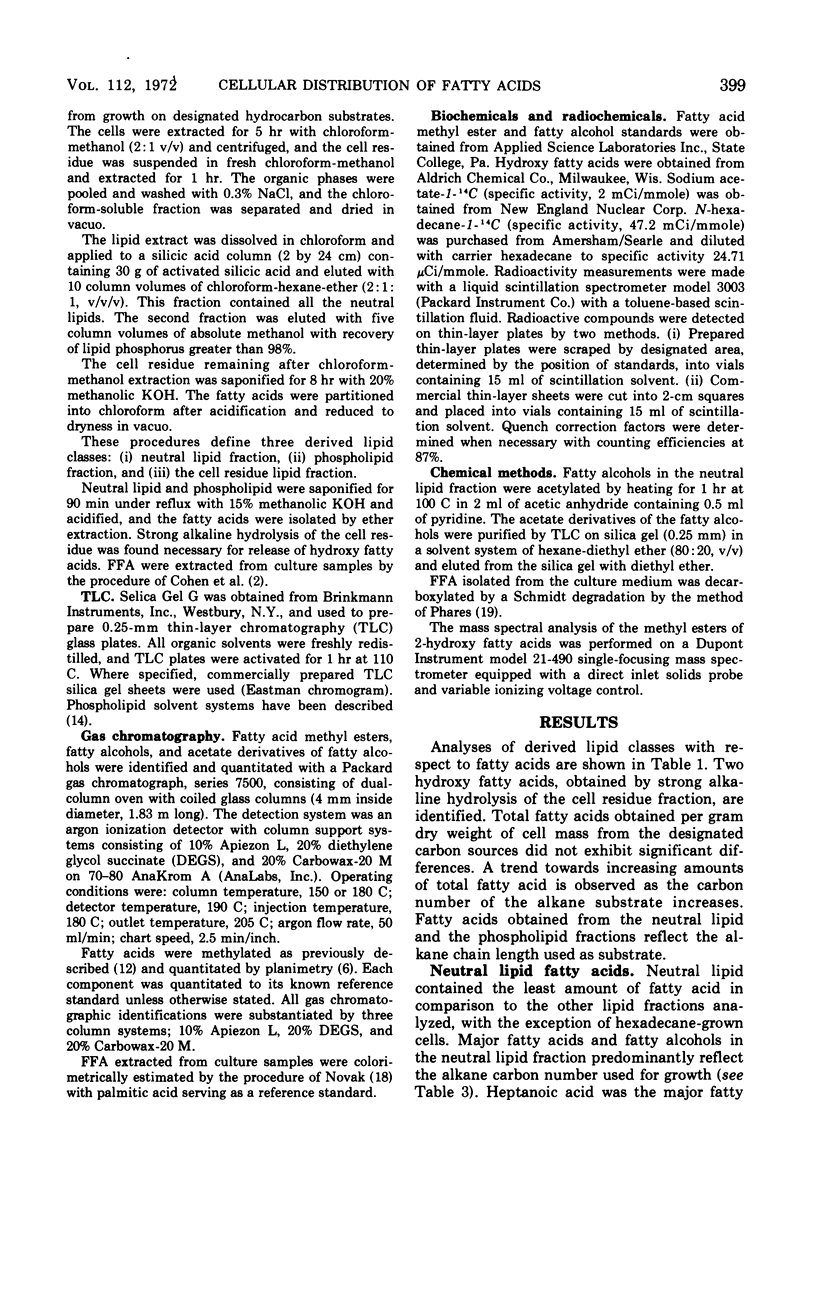
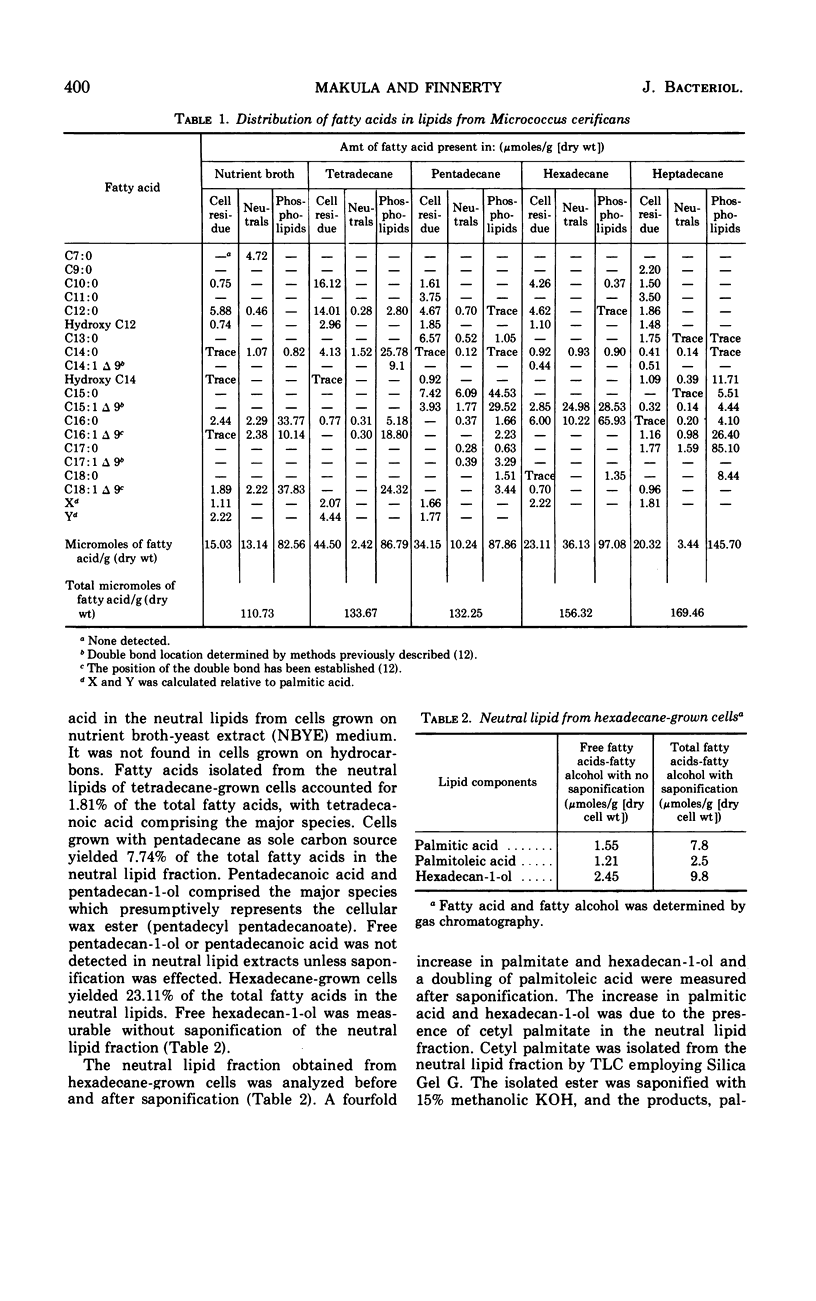
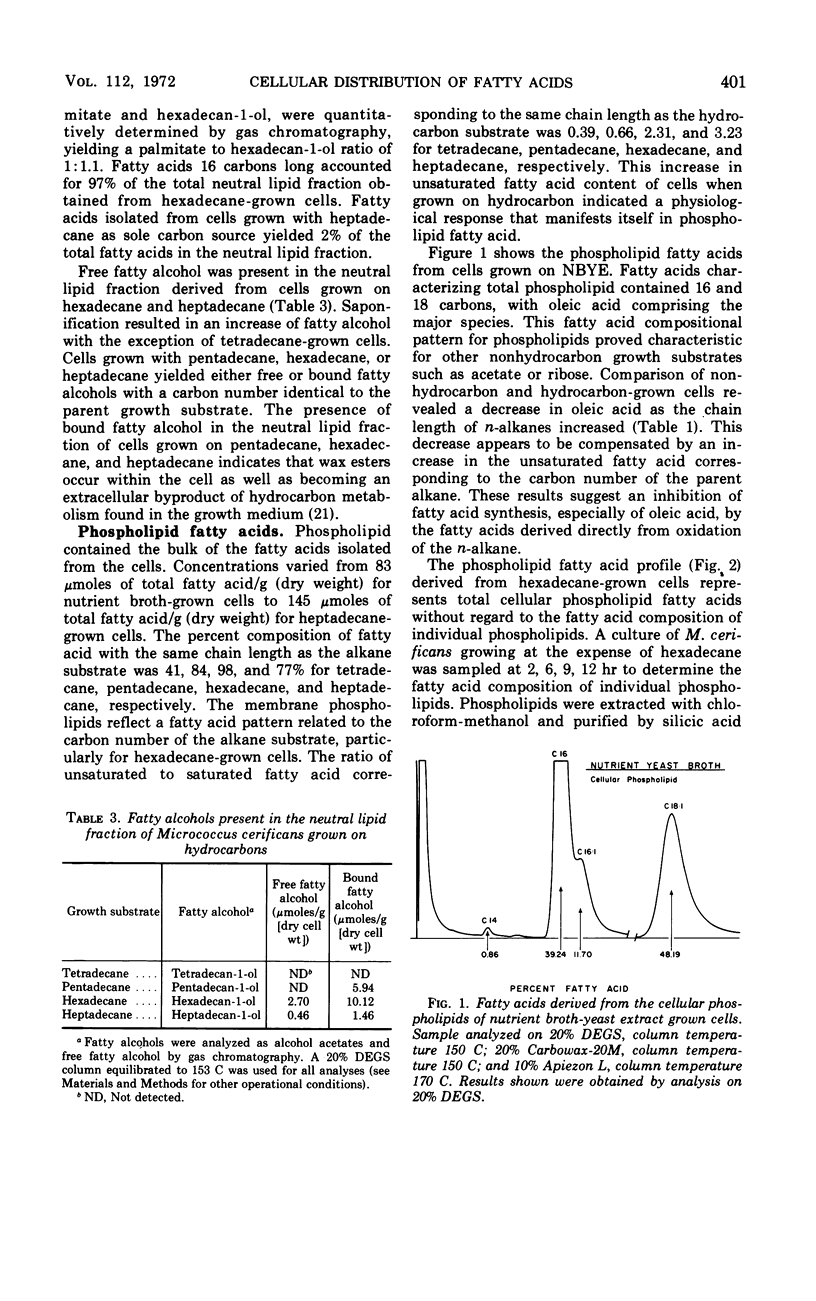
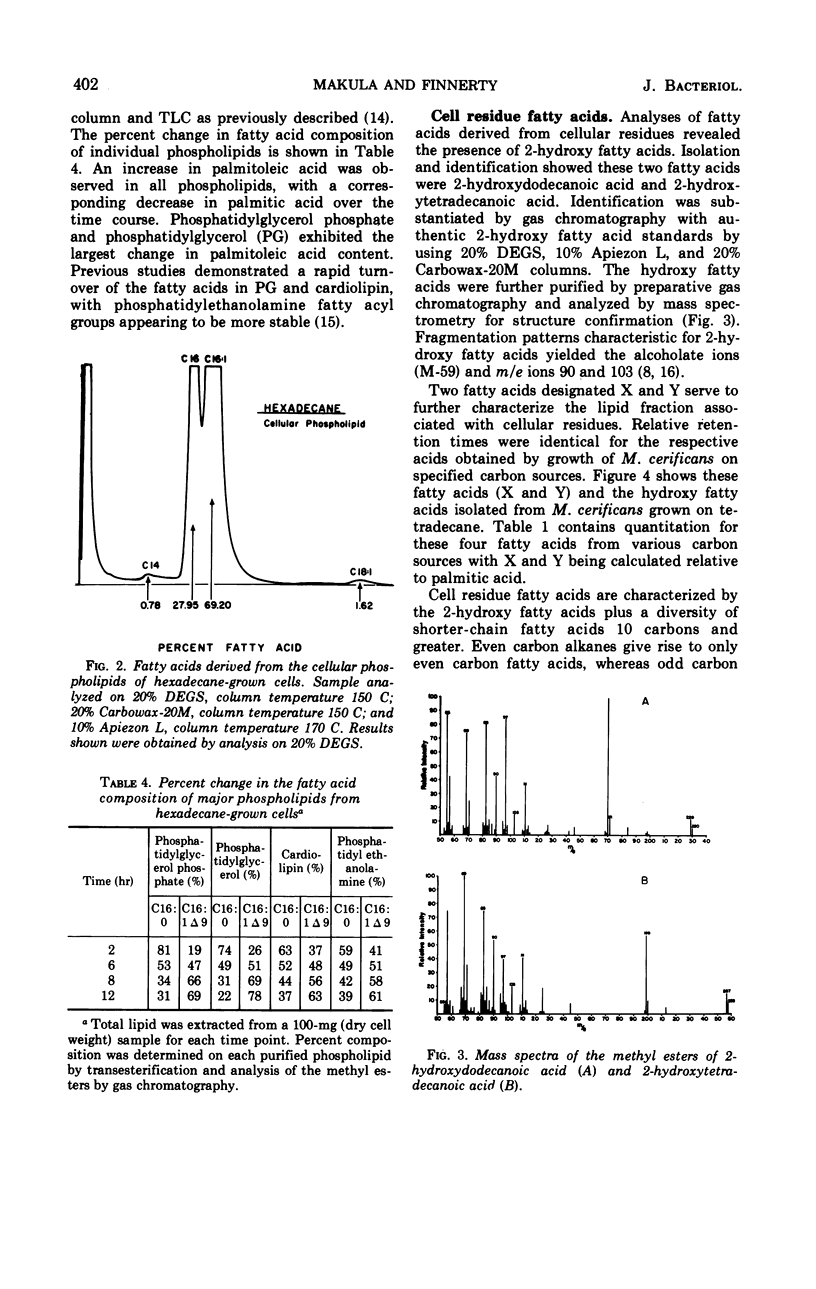
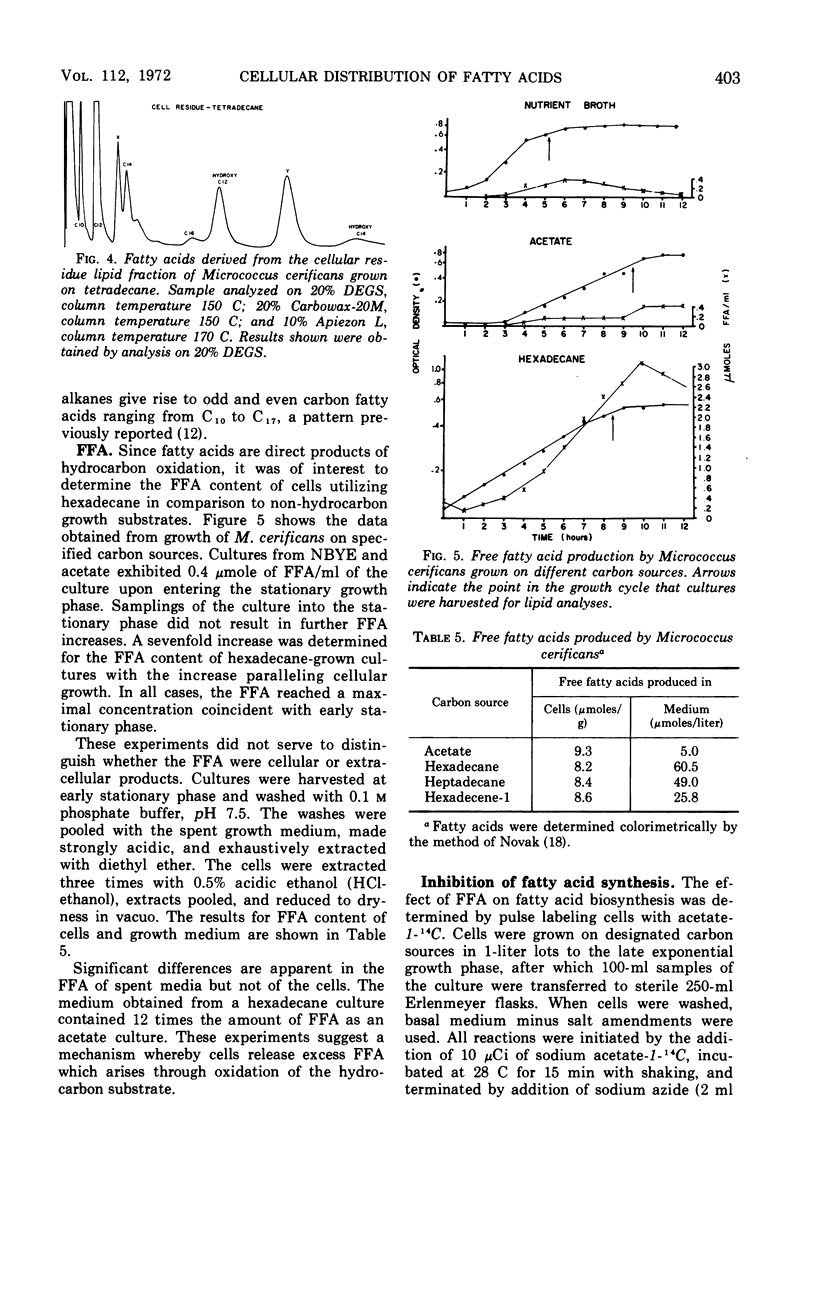
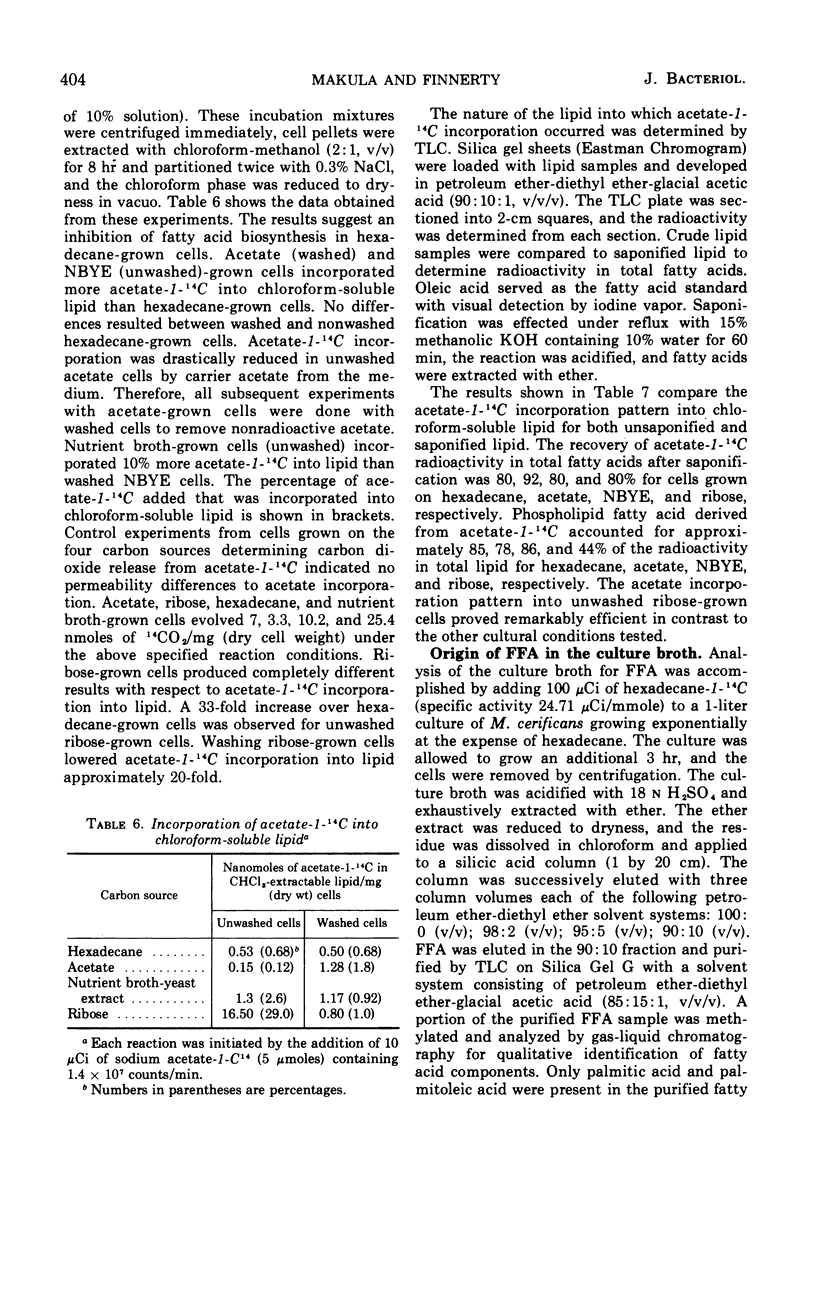
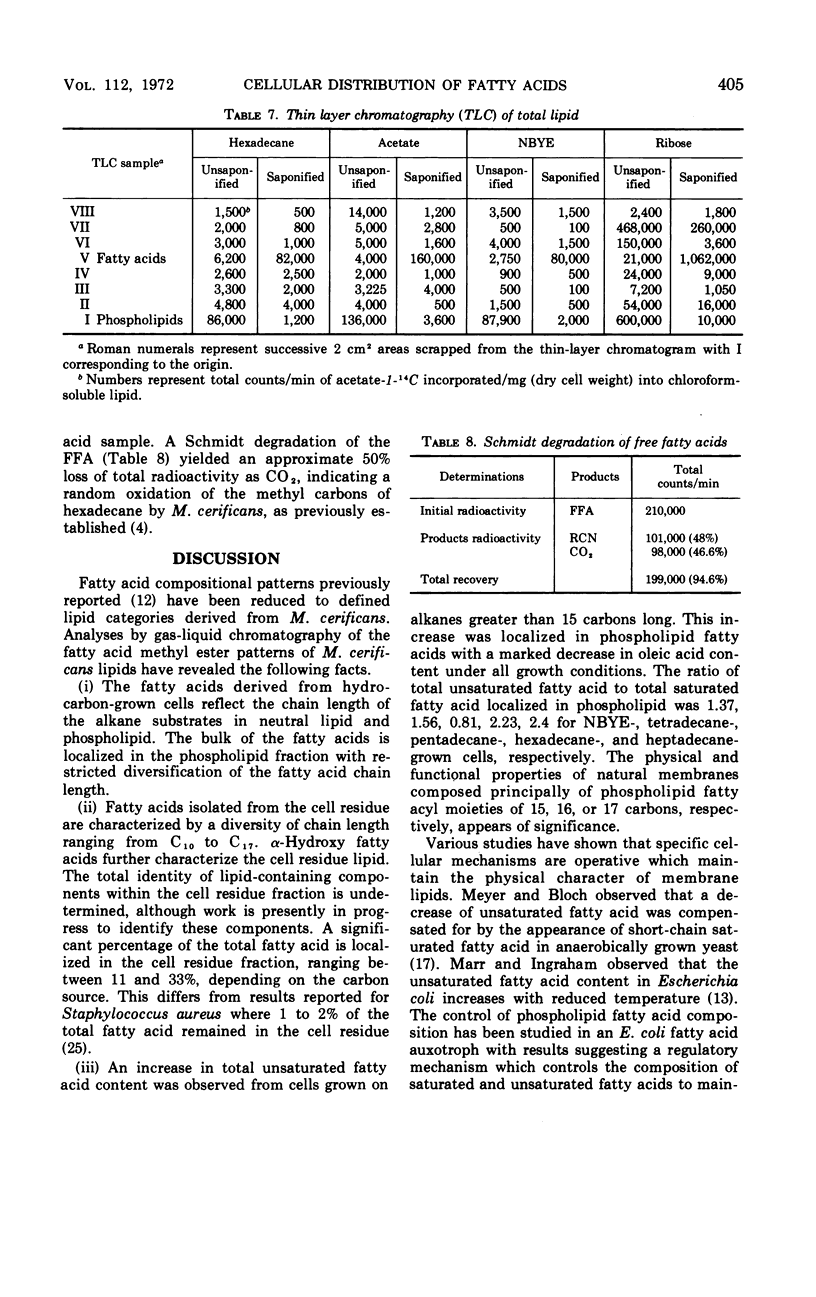
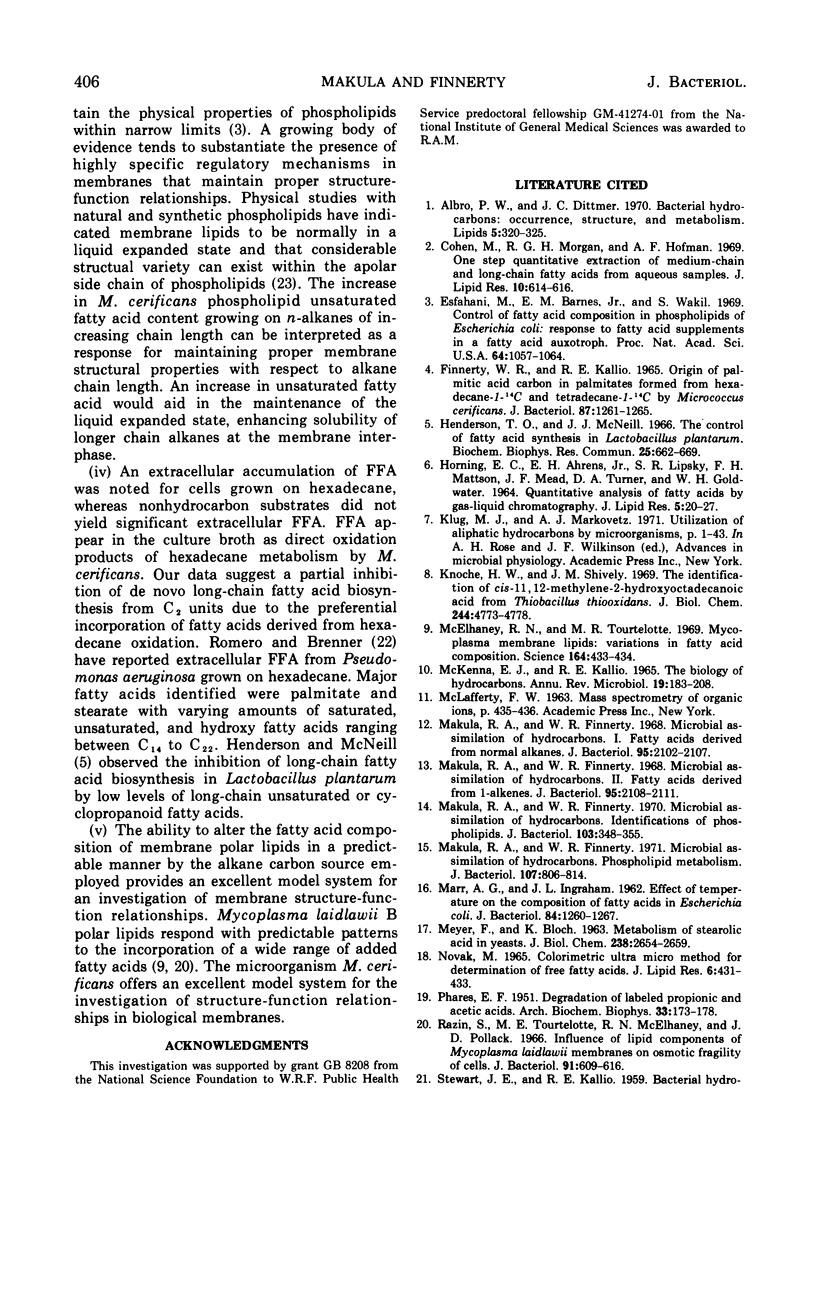
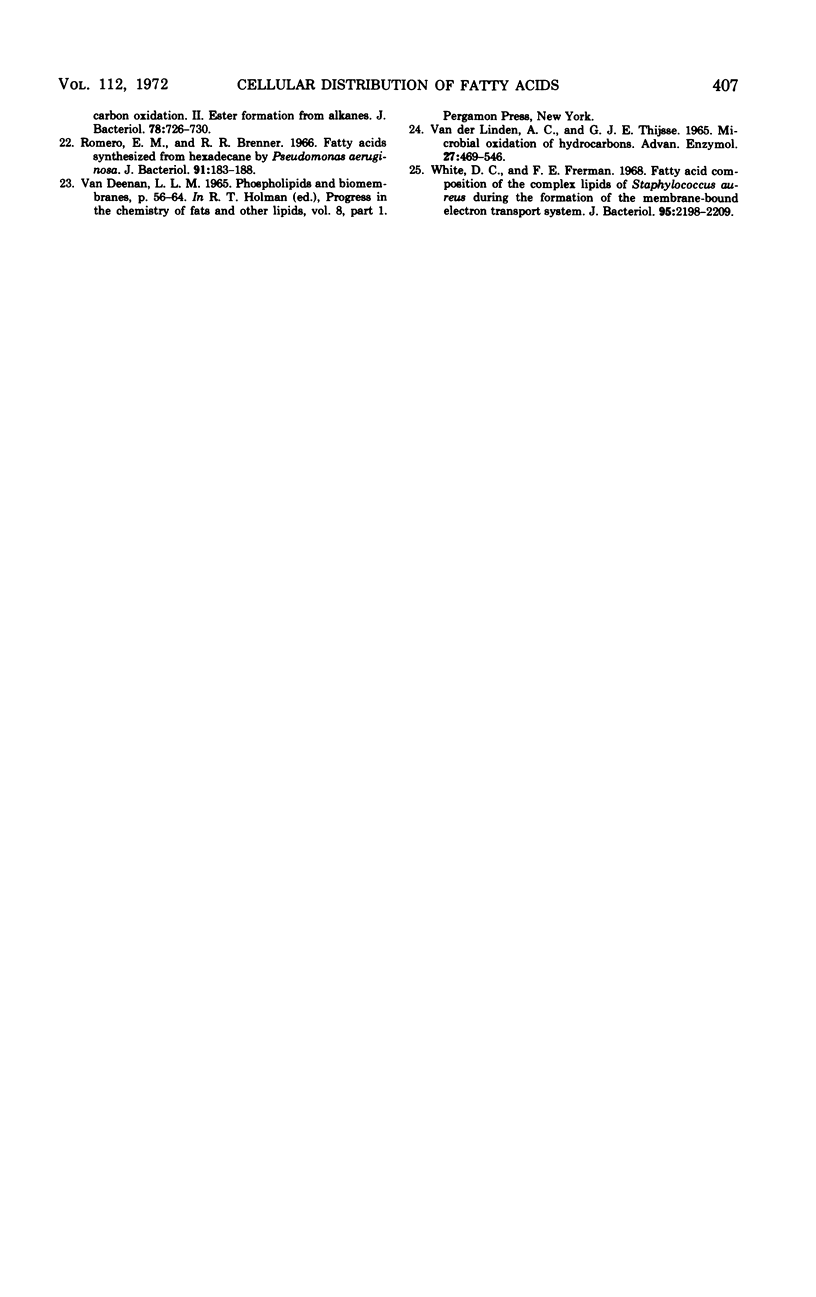
Selected References
These references are in PubMed. This may not be the complete list of references from this article.
- Albro P. W., Dittmer J. C. Bacterial hydrocarbons: occurrence, structure and metabolism. Lipids. 1970 Mar;5(3):320–325. doi: 10.1007/BF02531463. [DOI] [PubMed] [Google Scholar]
- Cohen M., Morgan R. G., Hofmann A. F. One-step quantitative extraction of medium-chain and long-chain fatty acids from aqueous samples. J Lipid Res. 1969 Sep;10(5):614–616. [PubMed] [Google Scholar]
- Esfahani M., Barnes E. M., Jr, Wakil S. J. Control of fatty acid composition in phospholipids of Escherichia coli: response to fatty acid supplements in a fatty acid auxotroph. Proc Natl Acad Sci U S A. 1969 Nov;64(3):1057–1064. doi: 10.1073/pnas.64.3.1057. [DOI] [PMC free article] [PubMed] [Google Scholar]
- FINNERTY W. R., KALLIO R. E. ORIGIN OF PALMITIC ACID CARBON IN PALMITATES FORMED FROM HEXADECANE-1-C-14 AND TETRADECANE-1-C-14 BY MICROCOCCUS CERIFICANS. J Bacteriol. 1964 Jun;87:1261–1265. doi: 10.1128/jb.87.6.1261-1265.1964. [DOI] [PMC free article] [PubMed] [Google Scholar]
- HORNING E. C., AHRENS E. H., Jr, LIPSKY S. R., MATTSON F. H., MEAD J. F., TURNER D. A., GOLDWATER W. H. QUANTITATIVE ANALYSIS OF FATTY ACIDS BY GAS-LIQUID CHROMATOGRAPHY. J Lipid Res. 1964 Jan;5:20–27. [PubMed] [Google Scholar]
- Klug M. J., Markovetz A. J. Utilization of aliphatic hydrocarbons by micro-organisms. Adv Microb Physiol. 1971;5:1–43. doi: 10.1016/s0065-2911(08)60404-x. [DOI] [PubMed] [Google Scholar]
- Knoche H. W., Shively J. M. The identification of cis-11,12-methylene-2-hydroxyoctadecanoic acid from Thiobacillus thiooxidans. J Biol Chem. 1969 Sep 10;244(17):4773–4778. [PubMed] [Google Scholar]
- MEYER F., BLOCH K. METABOLISM OF STEAROLIC ACID IN YEAST. J Biol Chem. 1963 Aug;238:2654–2659. [PubMed] [Google Scholar]
- Makula R. A., Finnerty W. R. Microbial assimilation of hydrocarbons: identification of phospholipids. J Bacteriol. 1970 Aug;103(2):348–355. doi: 10.1128/jb.103.2.348-355.1970. [DOI] [PMC free article] [PubMed] [Google Scholar]
- Makula R. A., Finnerty W. R. Microbial assimilation of hydrocarbons: phospholipid metabolism. J Bacteriol. 1971 Sep;107(3):806–814. doi: 10.1128/jb.107.3.806-814.1971. [DOI] [PMC free article] [PubMed] [Google Scholar]
- Makula R., Finnerty W. R. Microbial assimilation of hydrocarbons. I. Fatty acids derived from normal alkanes. J Bacteriol. 1968 Jun;95(6):2102–2107. doi: 10.1128/jb.95.6.2102-2107.1968. [DOI] [PMC free article] [PubMed] [Google Scholar]
- Makula R., Finnerty W. R. Microbial assimilation of hydrocarbons. II. Fatty acids derived from 1-alkenes. J Bacteriol. 1968 Jun;95(6):2108–2111. doi: 10.1128/jb.95.6.2108-2111.1968. [DOI] [PMC free article] [PubMed] [Google Scholar]
- Marr A. G., Ingraham J. L. EFFECT OF TEMPERATURE ON THE COMPOSITION OF FATTY ACIDS IN ESCHERICHIA COLI. J Bacteriol. 1962 Dec;84(6):1260–1267. doi: 10.1128/jb.84.6.1260-1267.1962. [DOI] [PMC free article] [PubMed] [Google Scholar]
- McElhaney R. N., Tourtellotte M. E. Mycoplasma membrane lipids: variations in fatty acid composition. Science. 1969 Apr 25;164(3878):433–434. doi: 10.1126/science.164.3878.433. [DOI] [PubMed] [Google Scholar]
- McKenna E. J., Kallio R. E. The biology of hydrocarbons. Annu Rev Microbiol. 1965;19:183–208. doi: 10.1146/annurev.mi.19.100165.001151. [DOI] [PubMed] [Google Scholar]
- NOVAK M. COLORIMETRIC ULTRAMICRO METHOD FOR THE DETERMINATION OF FREE FATTY ACIDS. J Lipid Res. 1965 Jul;6:431–433. [PubMed] [Google Scholar]
- PHARES E. F. Degradation of labeled propionic and acetic acids. Arch Biochem Biophys. 1951 Sep;33(2):173–178. doi: 10.1016/0003-9861(51)90094-x. [DOI] [PubMed] [Google Scholar]
- Razin S., Tourtellotte M. E., McElhaney R. N., Pollack J. D. Influence of lipid components of Mycoplasma laidlawii membranes on osmotic fragility of cells. J Bacteriol. 1966 Feb;91(2):609–616. doi: 10.1128/jb.91.2.609-616.1966. [DOI] [PMC free article] [PubMed] [Google Scholar]
- Romero E. M., Brenner R. R. Fatty acids synthesized from hexadecane by Pseudomonas aeruginosa. J Bacteriol. 1966 Jan;91(1):183–188. doi: 10.1128/jb.91.1.183-188.1966. [DOI] [PMC free article] [PubMed] [Google Scholar]
- STEWART J. E., KALLIO R. E. Bacterial hydrocarbon oxidation. II. Ester formation from alkanes. J Bacteriol. 1959 Nov;78:726–730. doi: 10.1128/jb.78.5.726-730.1959. [DOI] [PMC free article] [PubMed] [Google Scholar]
- White D. C., Frerman F. E. Fatty acid composition of the complex lipids of Staphylococcus aureus during the formation of the membrane-bound electron transport system. J Bacteriol. 1968 Jun;95(6):2198–2209. doi: 10.1128/jb.95.6.2198-2209.1968. [DOI] [PMC free article] [PubMed] [Google Scholar]
- van der Linden A. C., Thijsse G. J. The mechanisms of microbial oxidations of petroleum hydrocarbons. Adv Enzymol Relat Areas Mol Biol. 1965;27:469–546. doi: 10.1002/9780470122723.ch10. [DOI] [PubMed] [Google Scholar]