Abstract
The transport of the tricarboxylic acid cycle C4-dicarboxylic acids was studied in both the wild-type strain and tricarboxylic acid cycle mutants of Bacillus subtilis. Active transport of malate, fumarate, and succinate was found to be inducible by these dicarboxylic acids or by precursors to them, whereas glucose or closely related metabolites catabolite-repressed their uptake. l-Malate was found to be the best dicarboxylic acid transport inducer in succinic dehydrogenase, fumarase, and malic dehydrogenase mutants. Succinate and fumarate are accumulated over 100-fold in succinic dehydrogenase and fumarase mutants, respectively, whereas mutants lacking malate dehydrogenase were unable to accumulate significant quantities of the C4-dicarboxylic acids. The stereospecificity of this transport system was studied from a comparison of the rates of competitive inhibition of both succinate uptake and efflux in a succinate dehydrogenase mutant by utilizing thirty dicarboxylic acid analogues. The system was specific for the C4-dicarboxylic acids of the tricarboxylic acid cycle, neither citrate nor α-ketoglutarate were effective competitive inhibitors. Of a wide variety of metabolic inhibitors tested, inhibiors of oxidative phosphorylation and of the formation of proton gradients were the most potent inhibitors of transport. From the kinetics of dicarboxylic acid transport (Km approximately 10−4 M for succinate or fumarate in succinic acid dehydrogenase and fumarase mutants) and from the competitive inhibition studies, it was concluded that an inducible dicarboxylic acid transport system mediates the entry of malate, fumarate, or succinate into B. subtilis. Mutants devoid of α-ketoglutarate dehydrogenase were shown to accumulate both α-ketoglutarate and glutamate, and these metabolites subsequently inhibited the transport of all the C4-dicarboxylic acids, suggesting a regulatory role.
Full text
PDF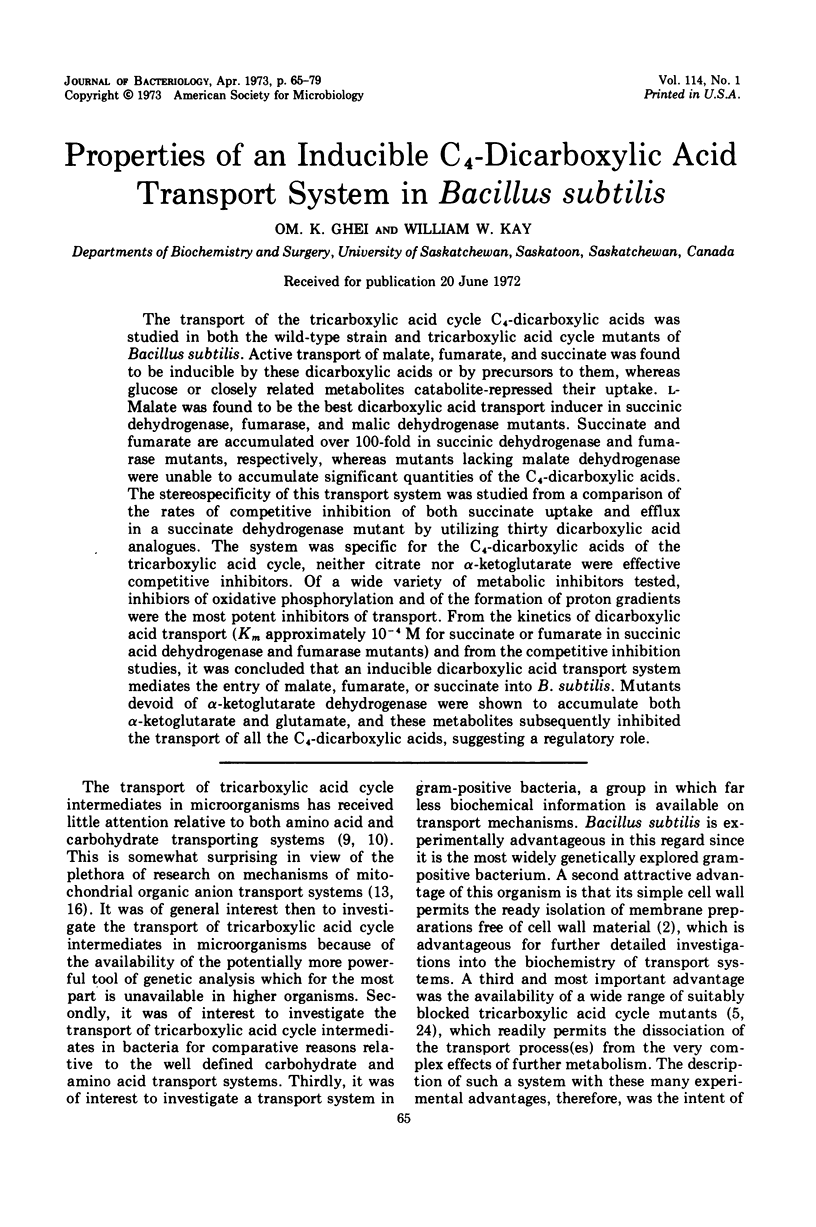
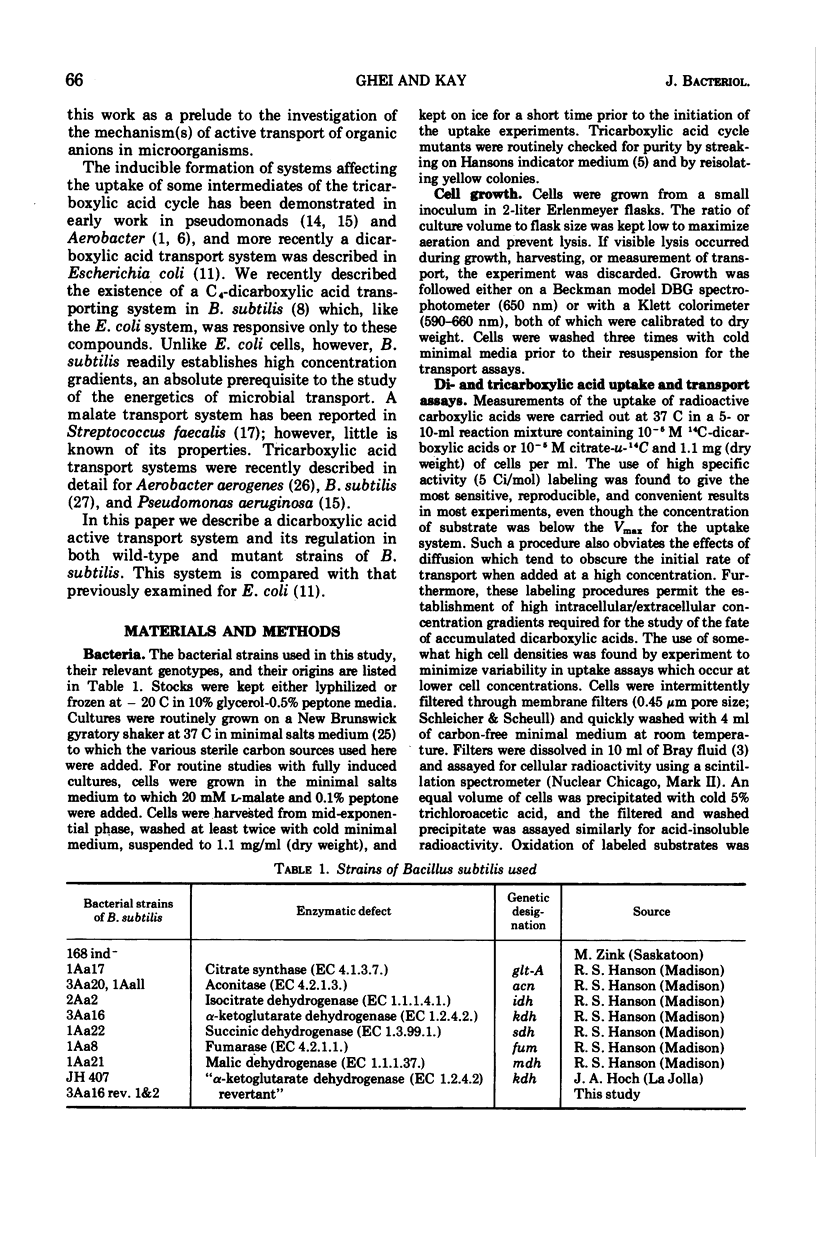
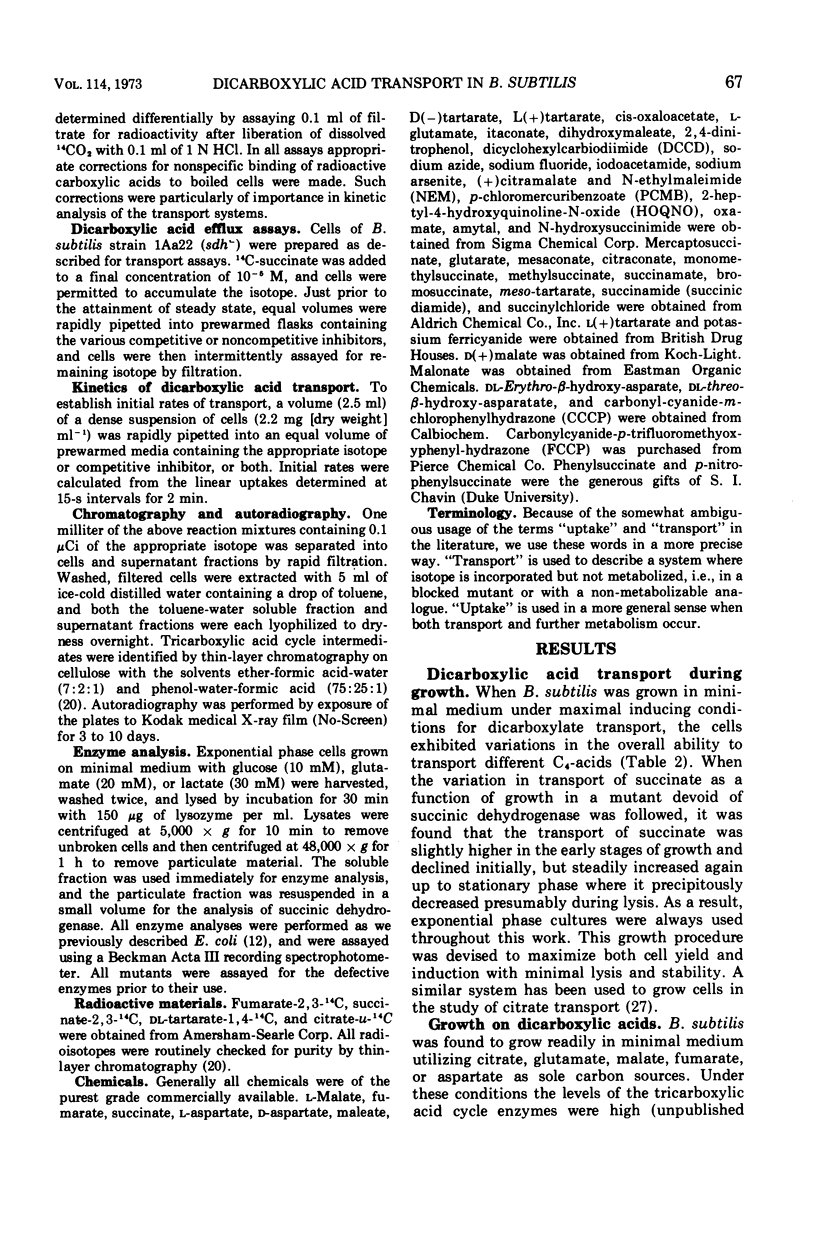
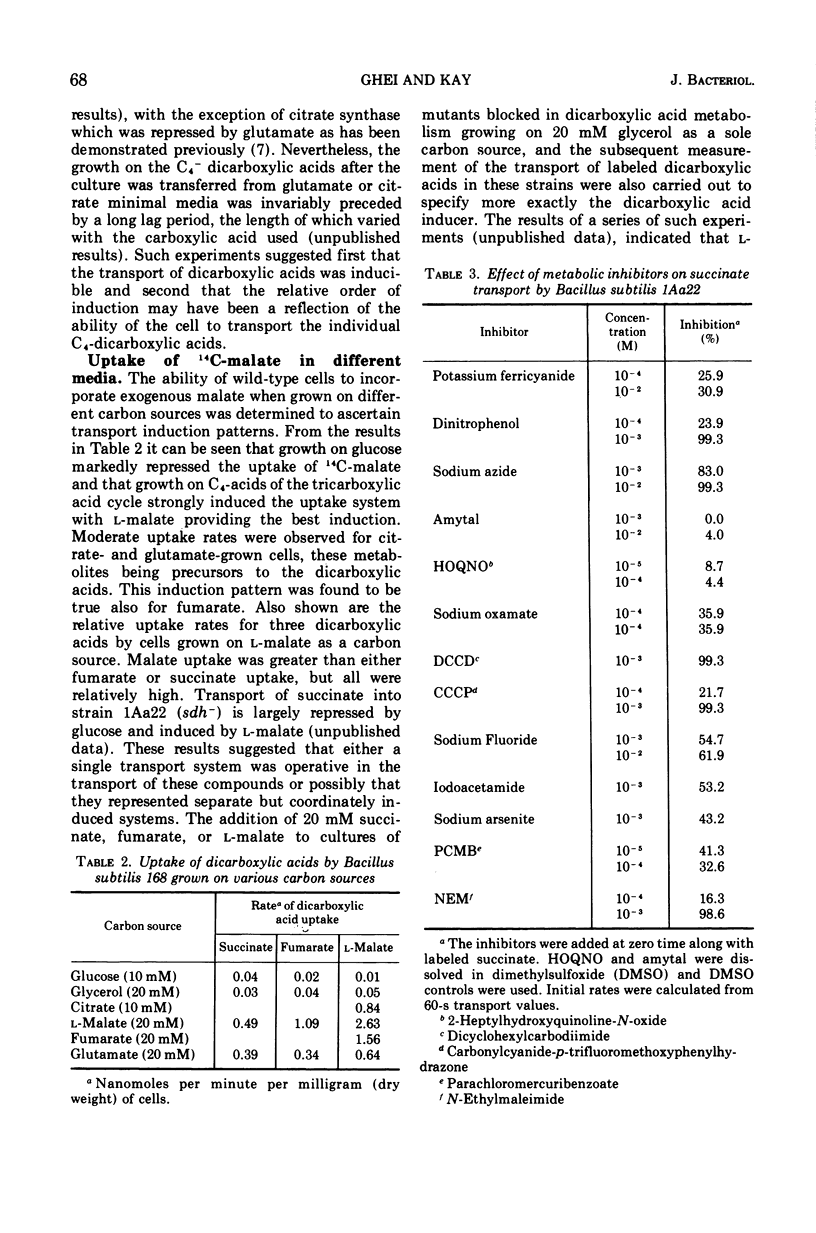
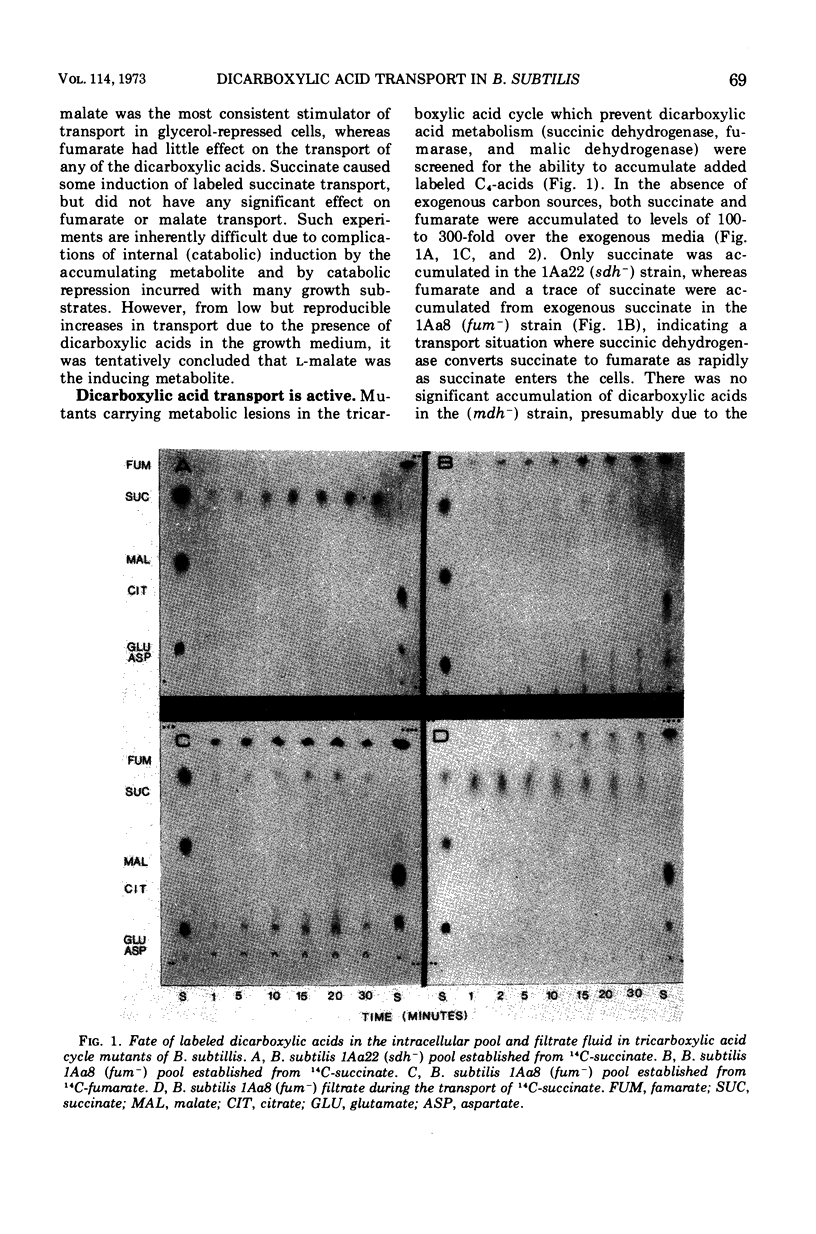
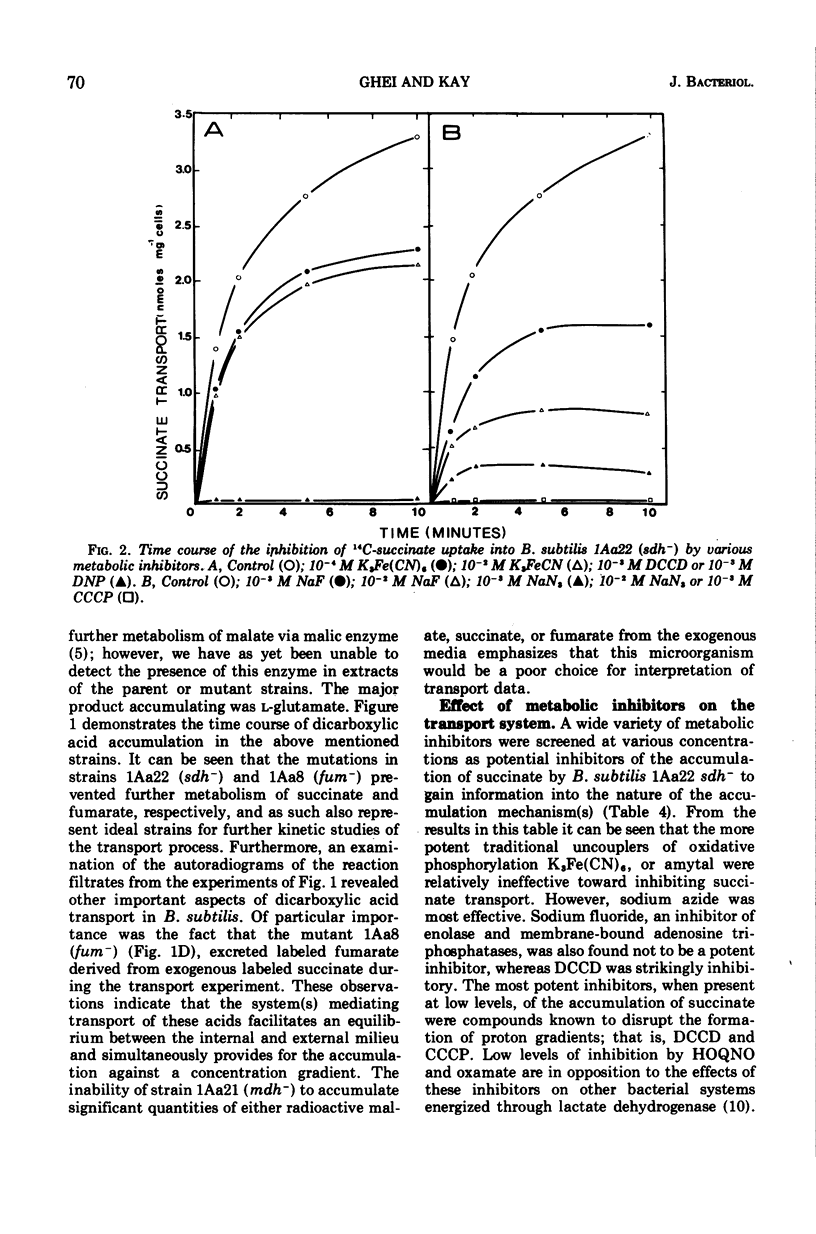
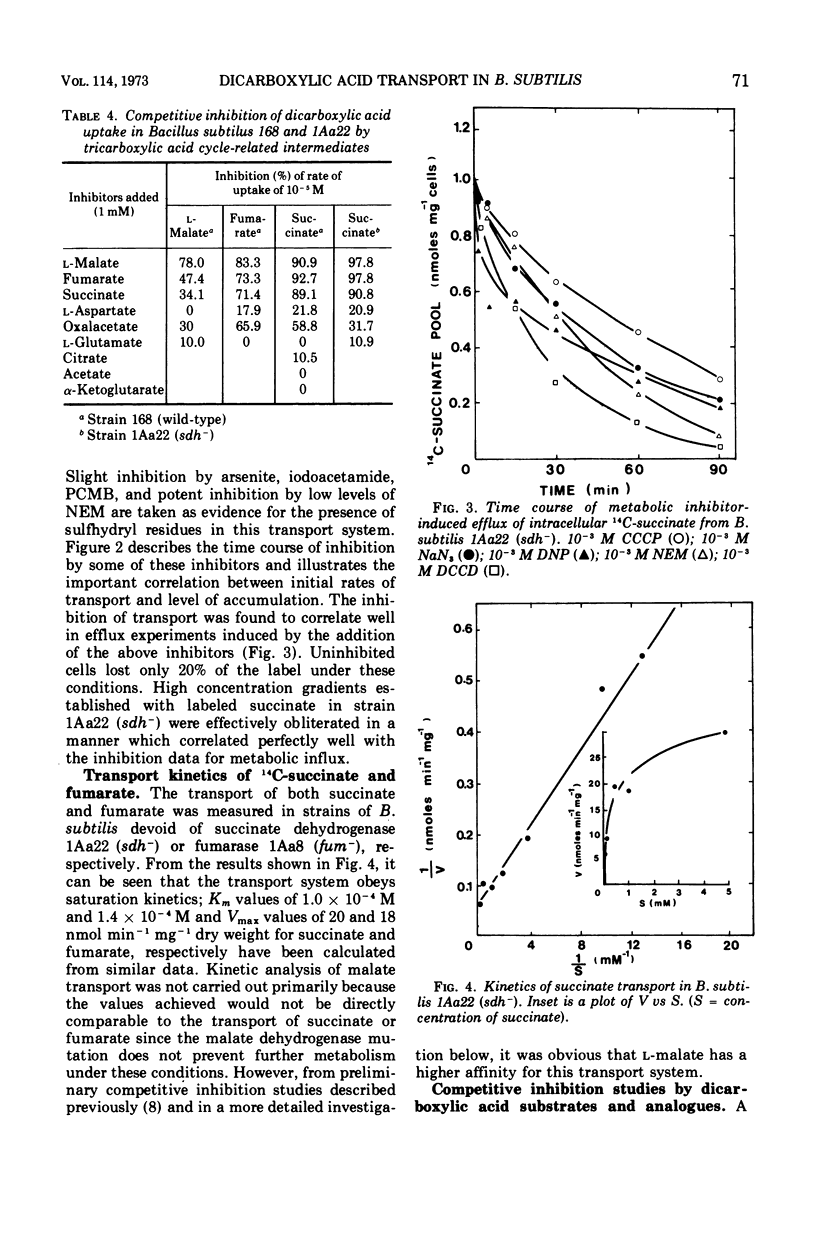
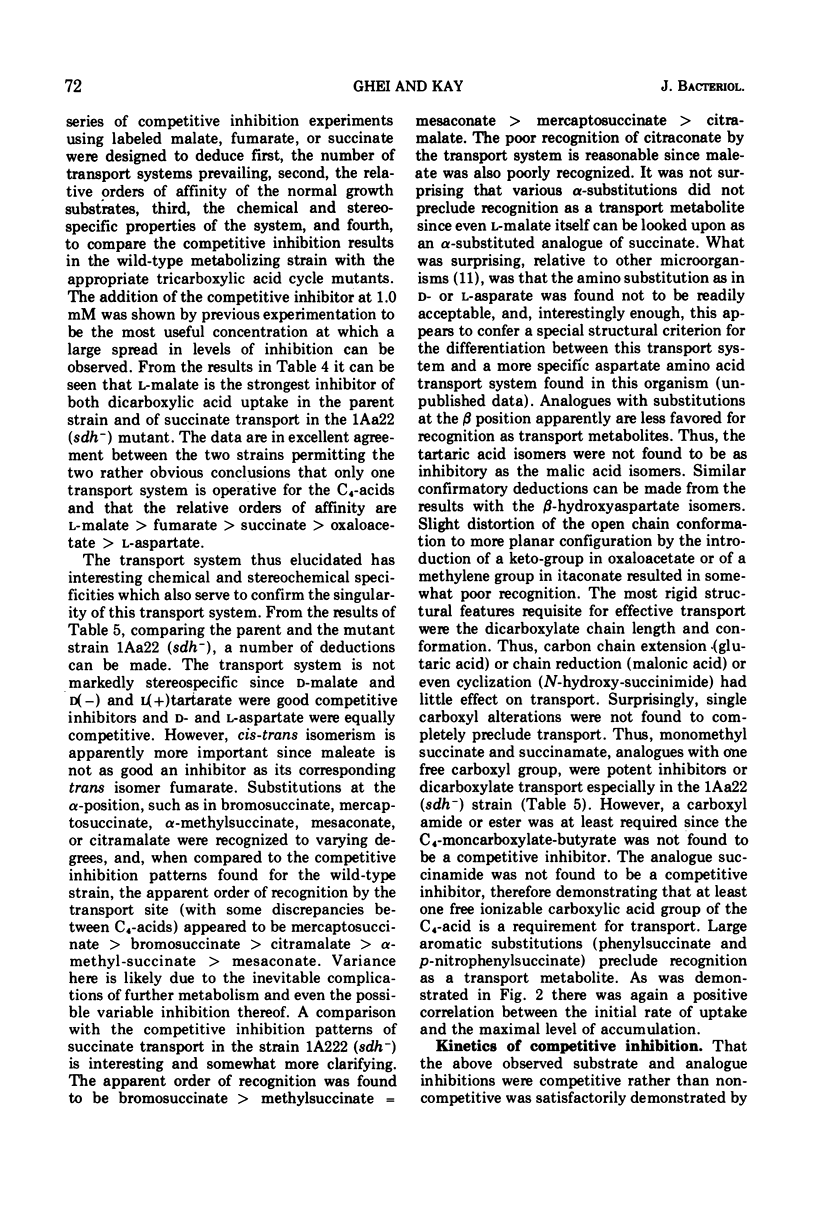
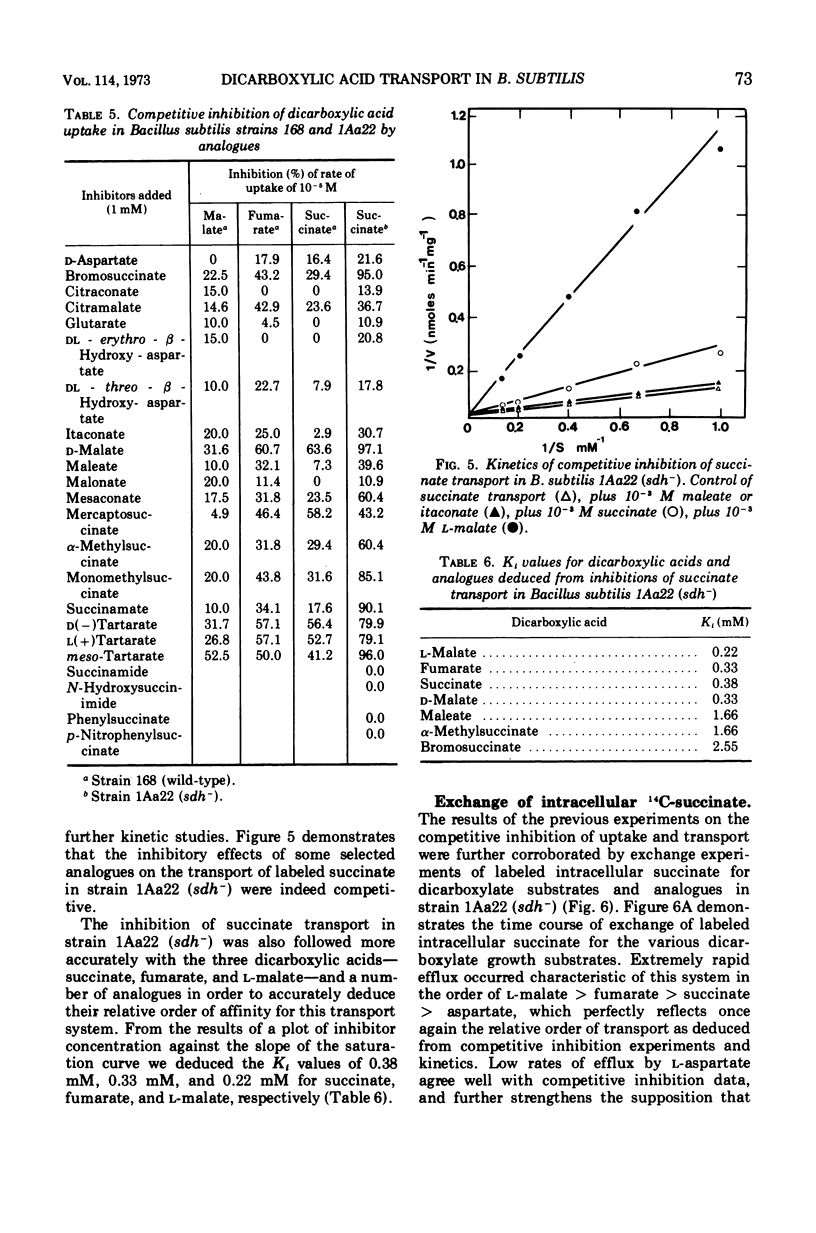
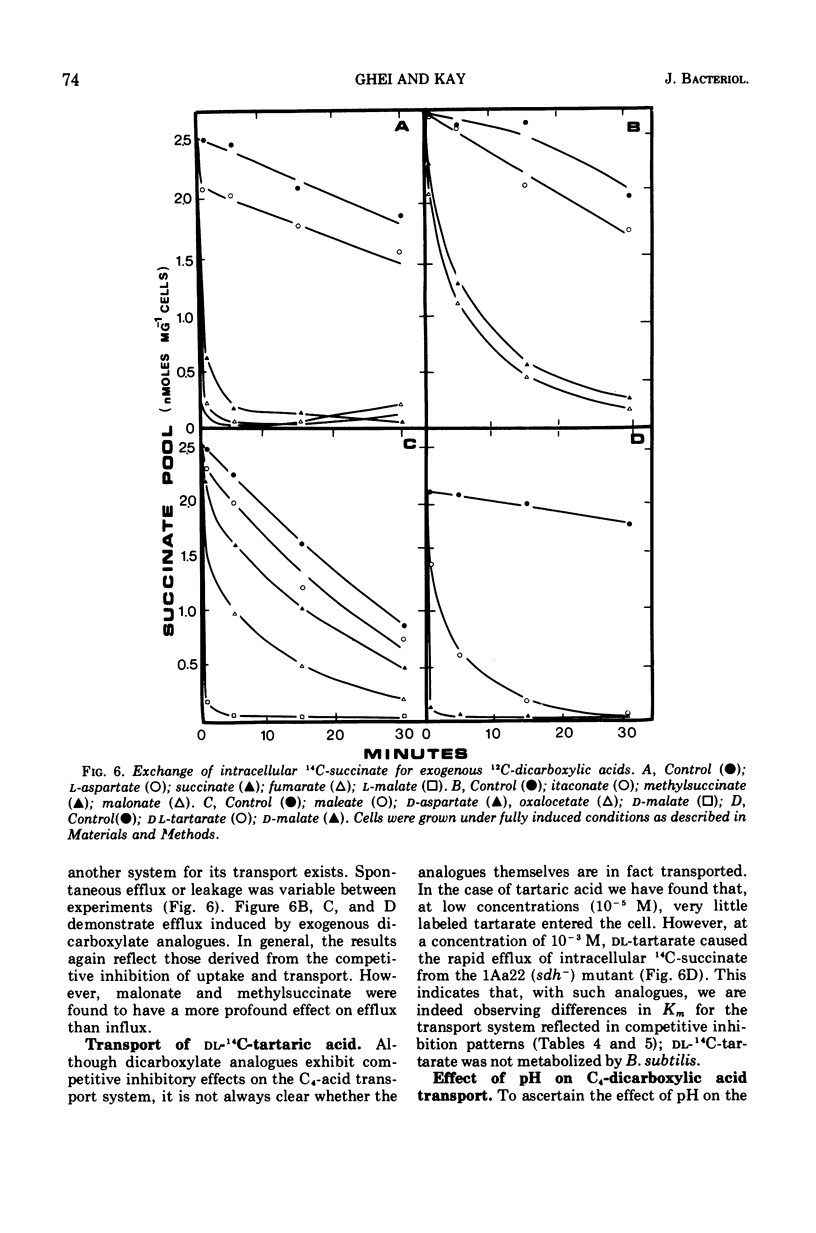
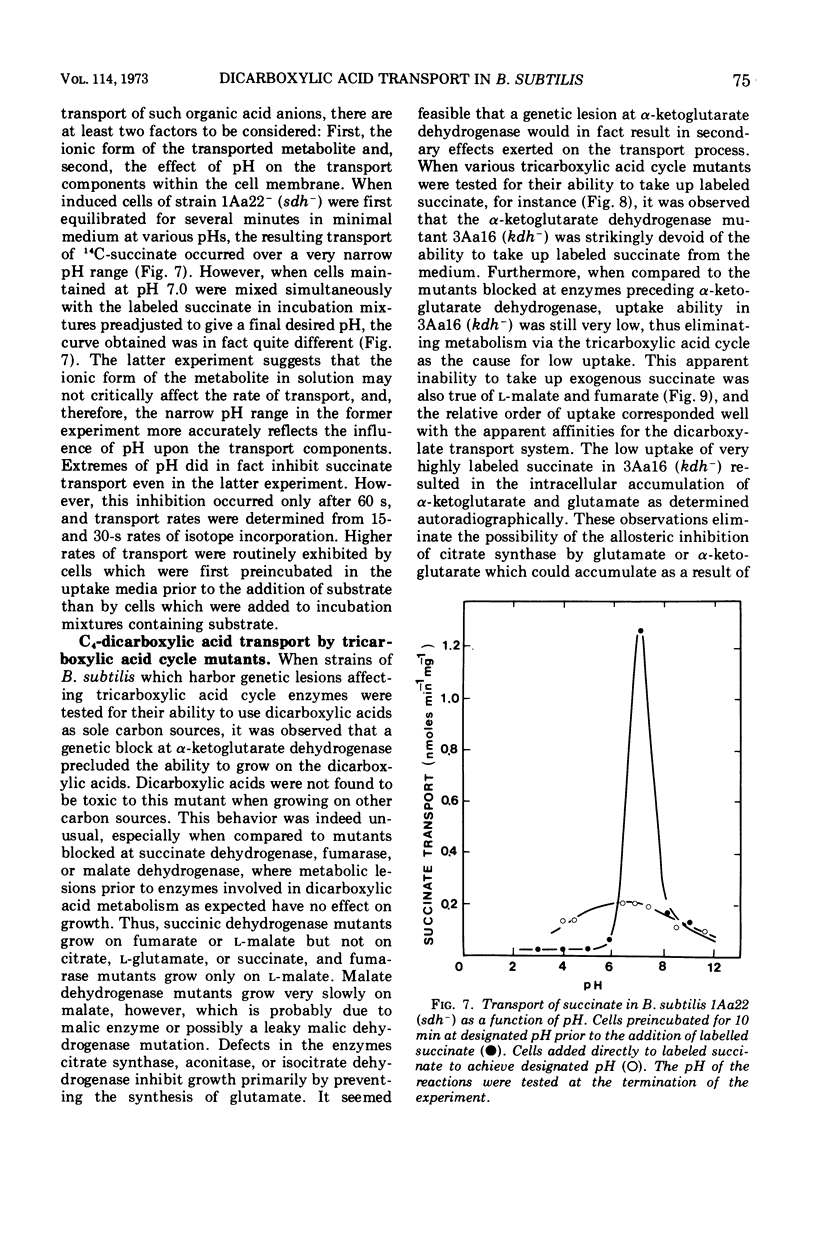
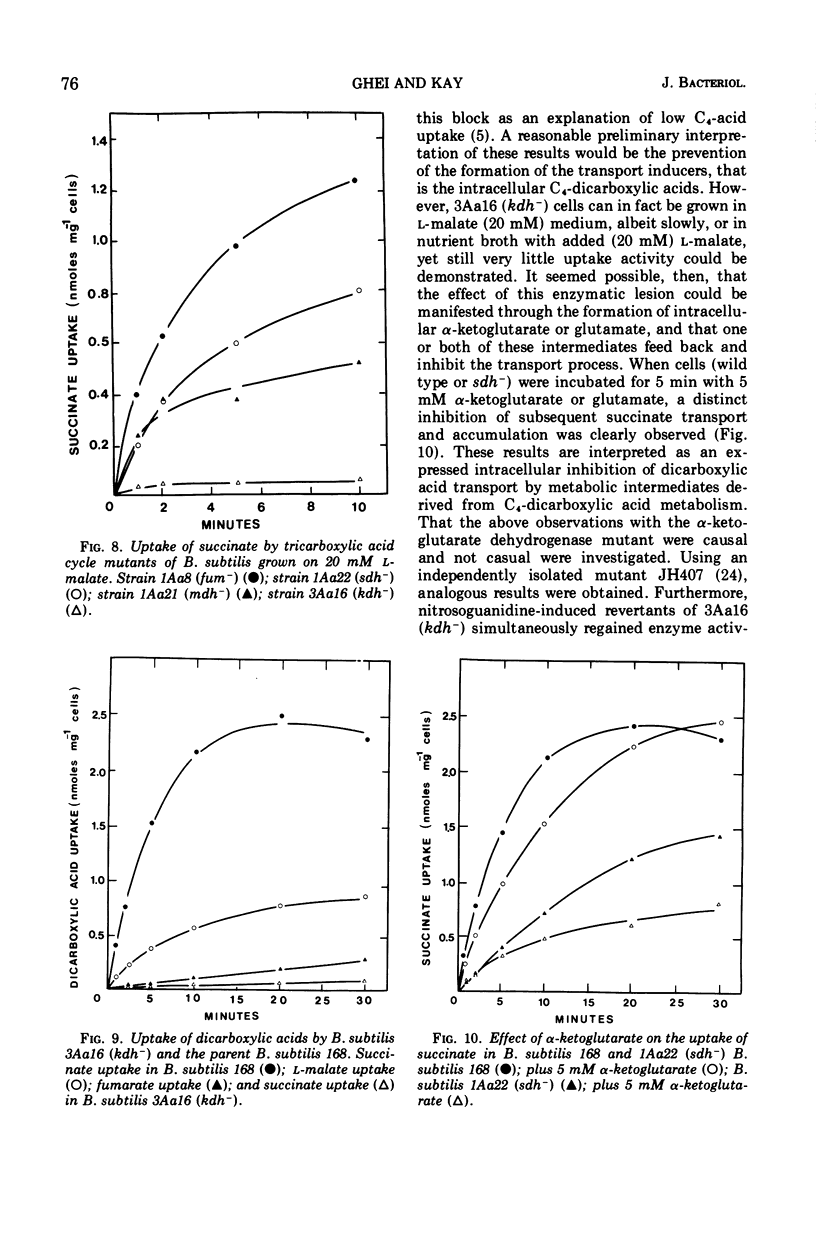
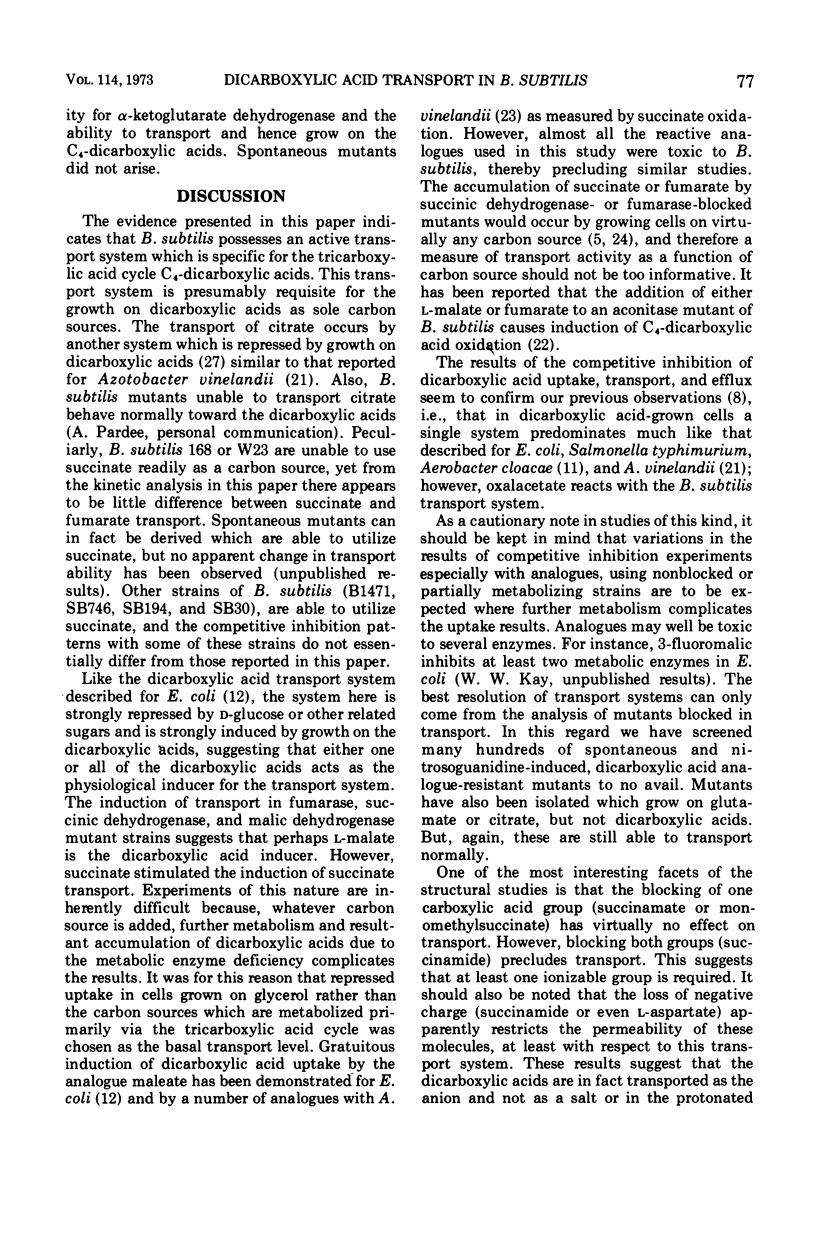
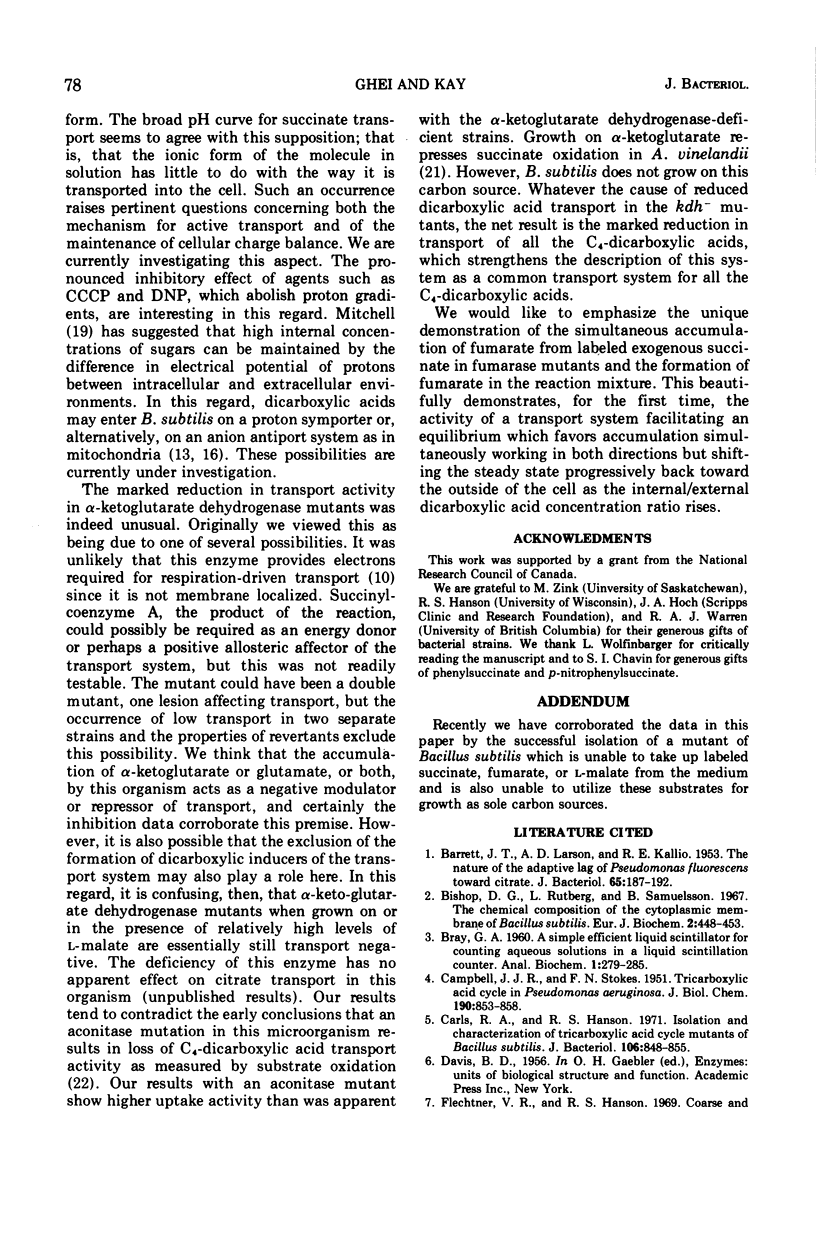
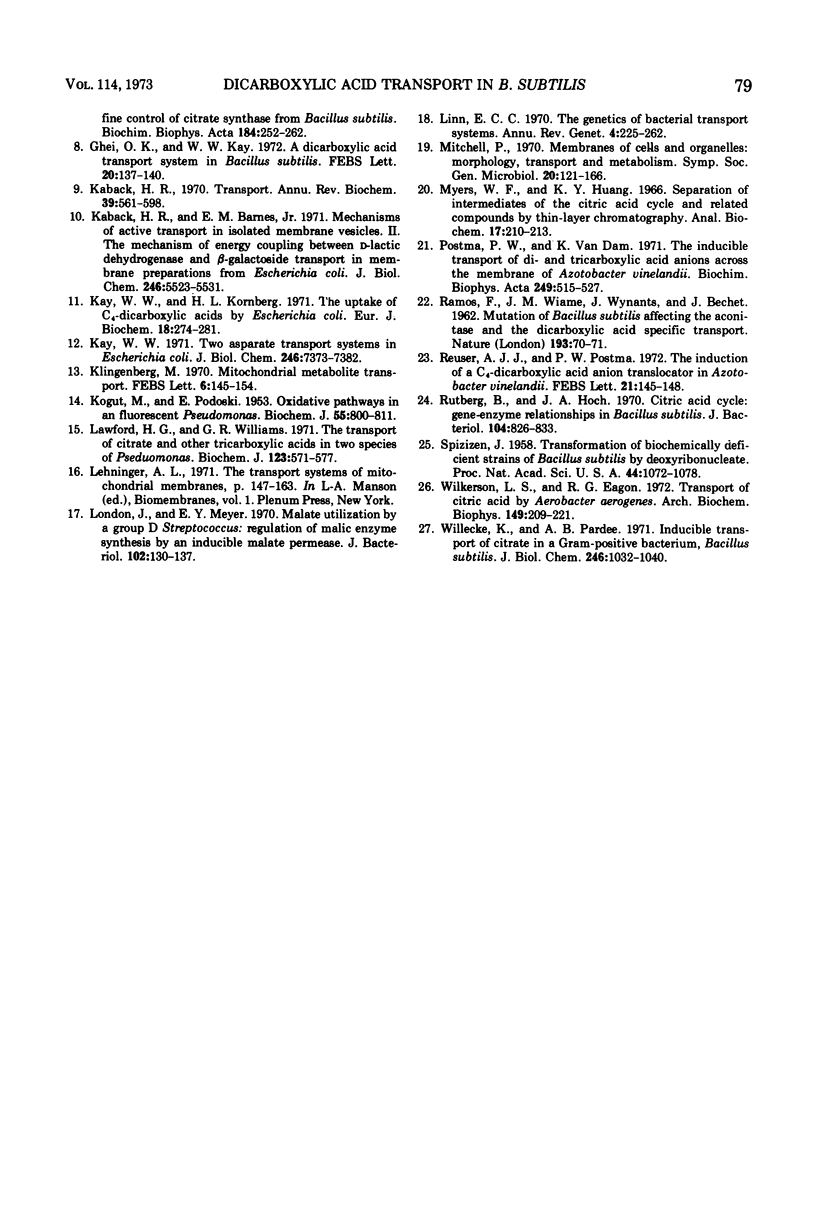
Images in this article
Selected References
These references are in PubMed. This may not be the complete list of references from this article.
- BARRETT J. T., LARSON A. D., KALLIO R. E. The nature of the adaptive lag of Pseudomonas fluorescens toward citrate. J Bacteriol. 1953 Feb;65(2):187–192. doi: 10.1128/jb.65.2.187-192.1953. [DOI] [PMC free article] [PubMed] [Google Scholar]
- Bishop D. G., Rutberg L., Samuelsson B. The chemical composition of the cytoplasmic membrane of Bacillus subtilis. Eur J Biochem. 1967 Nov;2(4):448–453. doi: 10.1111/j.1432-1033.1967.tb00158.x. [DOI] [PubMed] [Google Scholar]
- CAMPBELL J. J. R., STOKES F. N. Tricarboxylic acid cycle in Pseudomonas aeruginosa. J Biol Chem. 1951 Jun;190(2):853–858. [PubMed] [Google Scholar]
- Carls R. A., Hanson R. S. Isolation and characterization of tricarboxylic acid cycle mutants of Bacillus subtilis. J Bacteriol. 1971 Jun;106(3):848–855. doi: 10.1128/jb.106.3.848-855.1971. [DOI] [PMC free article] [PubMed] [Google Scholar]
- Flechtner V. R., Hanson R. S. Coarse and fine control of citrate synthase from Bacillus subtilis. Biochim Biophys Acta. 1969 Jul 30;184(2):252–262. doi: 10.1016/0304-4165(69)90027-0. [DOI] [PubMed] [Google Scholar]
- Ghei Om K., Kay William W. A Dicarboxyclic acid transport system in Bacillus subtilis. FEBS Lett. 1972 Feb 1;20(2):137–140. doi: 10.1016/0014-5793(72)80777-4. [DOI] [PubMed] [Google Scholar]
- KOGUT M., PODOSKI E. P. Oxidative pathways in a fluorescent Pseudomonas. Biochem J. 1953 Dec;55(5):800–811. doi: 10.1042/bj0550800. [DOI] [PMC free article] [PubMed] [Google Scholar]
- Kaback H. R., Barnes E. M., Jr Mechanisms of active transport in isolated membrane vesicles. II. The mechanism of energy coupling between D-lactic dehydrogenase and beta-galactoside transport in membrane preparations from Escherichia coli. J Biol Chem. 1971 Sep 10;246(17):5523–5531. [PubMed] [Google Scholar]
- Kaback H. R. Transport. Annu Rev Biochem. 1970;39:561–598. doi: 10.1146/annurev.bi.39.070170.003021. [DOI] [PubMed] [Google Scholar]
- Kay W. W., Kornberg H. L. The uptake of C4-dicarboxylic acids by Escherichia coli. Eur J Biochem. 1971 Jan;18(2):274–281. doi: 10.1111/j.1432-1033.1971.tb01240.x. [DOI] [PubMed] [Google Scholar]
- Kay W. W. Two aspartate transport systems in Escherichia coli. J Biol Chem. 1971 Dec 10;246(23):7373–7382. [PubMed] [Google Scholar]
- Klingenberg M. Mitochondria metabolite transport. FEBS Lett. 1970 Feb 16;6(3):145–154. doi: 10.1016/0014-5793(70)80044-8. [DOI] [PubMed] [Google Scholar]
- Lawford H. G., Williams G. R. The transport of citrate and other tricarboxylic acids in two species of Pseudomonas. Biochem J. 1971 Jul;123(4):571–577. doi: 10.1042/bj1230571. [DOI] [PMC free article] [PubMed] [Google Scholar]
- Lin E. C. The genetics of bacterial transport systems. Annu Rev Genet. 1970;4:225–262. doi: 10.1146/annurev.ge.04.120170.001301. [DOI] [PubMed] [Google Scholar]
- London J., Meyer E. Y. Malate utilization by a group D Streptococcus: regulation of malic enzyme synthesis by an inducible malate permease. J Bacteriol. 1970 Apr;102(1):130–137. doi: 10.1128/jb.102.1.130-137.1970. [DOI] [PMC free article] [PubMed] [Google Scholar]
- Myers W. F., Huang K. Y. Separation of intermediates of the citric acid cycle and related compounds by thin-layer chromatography. Anal Biochem. 1966 Nov;17(2):210–213. doi: 10.1016/0003-2697(66)90199-0. [DOI] [PubMed] [Google Scholar]
- Postma P. W., van Dam K. The inducible transport of DI- and tricarboxylic acid anions across the membrane of Azotobacter vinelandii. Biochim Biophys Acta. 1971 Dec 3;249(2):515–527. doi: 10.1016/0005-2736(71)90127-1. [DOI] [PubMed] [Google Scholar]
- RAMOS F., WIAME J. M., WYNANTS J., BECHET J. Effect of mutant of Bacillus subtilis on the specific transport of aconitase and dicarboxylic acid. Nature. 1962 Jan 6;193:70–71. doi: 10.1038/193070a0. [DOI] [PubMed] [Google Scholar]
- Reuser A. J.J., Postma P. W. The induction of a C(4)-dicarboxylic acid anion translocator in Azotobacter vinelandii. FEBS Lett. 1972 Mar 15;21(2):145–148. doi: 10.1016/0014-5793(72)80124-8. [DOI] [PubMed] [Google Scholar]
- Rutberg B., Hoch J. A. Citric acid cycle: gene-enzyme relationships in Bacillus subtilis. J Bacteriol. 1970 Nov;104(2):826–833. doi: 10.1128/jb.104.2.826-833.1970. [DOI] [PMC free article] [PubMed] [Google Scholar]
- Spizizen J. TRANSFORMATION OF BIOCHEMICALLY DEFICIENT STRAINS OF BACILLUS SUBTILIS BY DEOXYRIBONUCLEATE. Proc Natl Acad Sci U S A. 1958 Oct 15;44(10):1072–1078. doi: 10.1073/pnas.44.10.1072. [DOI] [PMC free article] [PubMed] [Google Scholar]
- Wilkerson L. S., Eagon R. G. Transport of citric acid by Aerobacter aerogenes. Arch Biochem Biophys. 1972 Mar;149(1):209–221. doi: 10.1016/0003-9861(72)90316-5. [DOI] [PubMed] [Google Scholar]
- Willecke K., Pardee A. B. Inducible transport of citrate in a Gram-positive bacterium, Bacillus subtilis. J Biol Chem. 1971 Feb 25;246(4):1032–1040. [PubMed] [Google Scholar]