Abstract
By a combination of luciferase and fluorescence methods adenine nucleotide pools in Neurospora crassa have been examined under various conditions of growth and metabolic inhibition. During sustained exponential growth (25 C, shaking liquid cultures), the intracellular adenosine 5′-triphosphate (ATP) concentration, [ATP]i, rises slowly from the conidial level near 1 mM (1 mmol/kg of cell water) to a maximum of 2.0 to 2.5 mM at 14 h, after which it slowly declines. The adenosine 5′-diphosphate and adenosine 5′-monophosphate (AMP) curves show two peaks, at 8 and 20 h, with a minimum at 16 h. The “energy charge” function varies around a mean of 0.72 throughout the period of exponential growth. Transferral of growing cells to buffer lacking a nitrogen source stabilizes the [ATP]i near 2.5 mM, apparently independent of the cell age, and most studies of metabolic inhibitors were carried out on cells grown 14 to 16 h and then shifted to N-free buffer. Under these conditions sudden respiratory blockade (cyanide) produces exponential decay of ATP with a time constant of about 5.7 s (half-time of 3.9 s), and at a rate which implies a minimal ATP turnover of 0.44 mM/min. This figure is about one-third the rate (1.17 mM/min) which would be calculated from steady-state respiration, a discrepancy which may partly be accounted for by transphosphorylation from appreciable amounts of non-adenine nucleoside di- and triphosphates present in Neurospora. For all three adenine nucleotides, the transients associated with sudden respiratory blockade include overshoots or undershoots of several minutes duration, which are consistent with feedback regulation of glycolysis by the AMP/ATP ratio.
Full text
PDF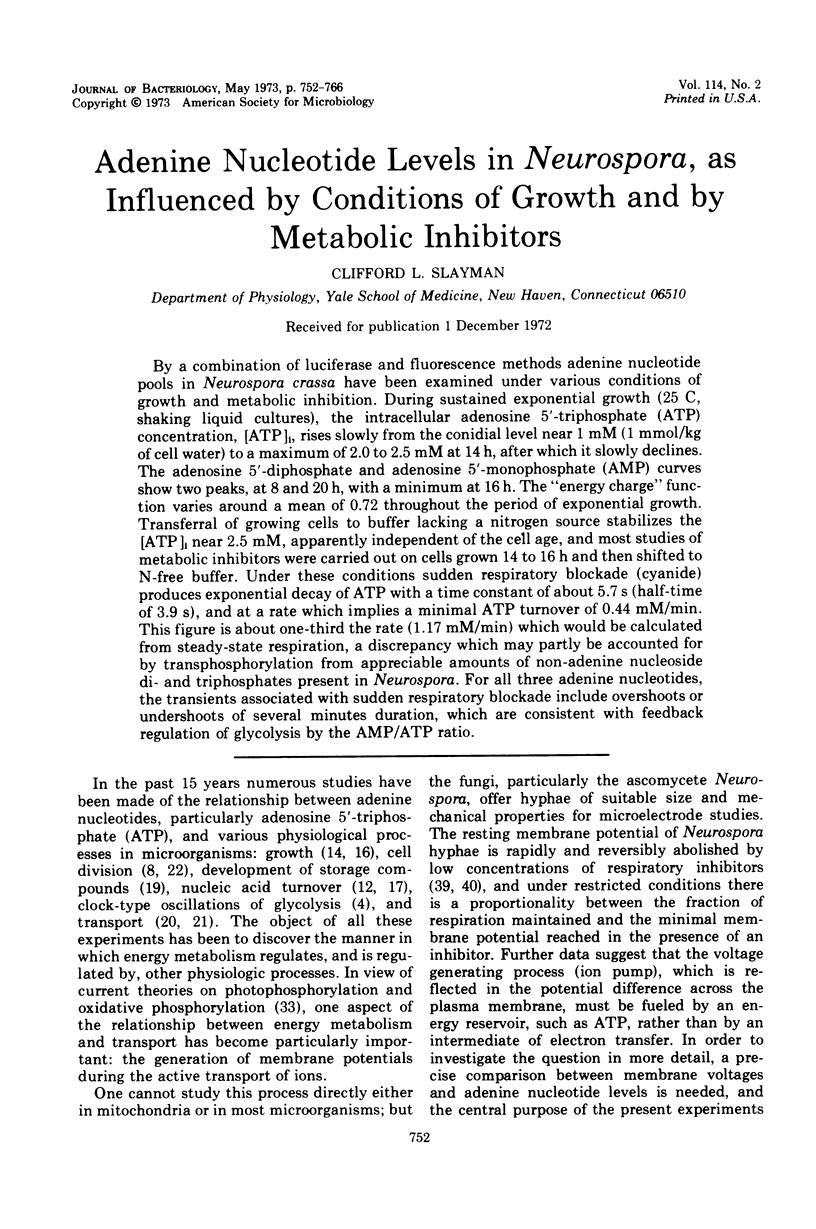
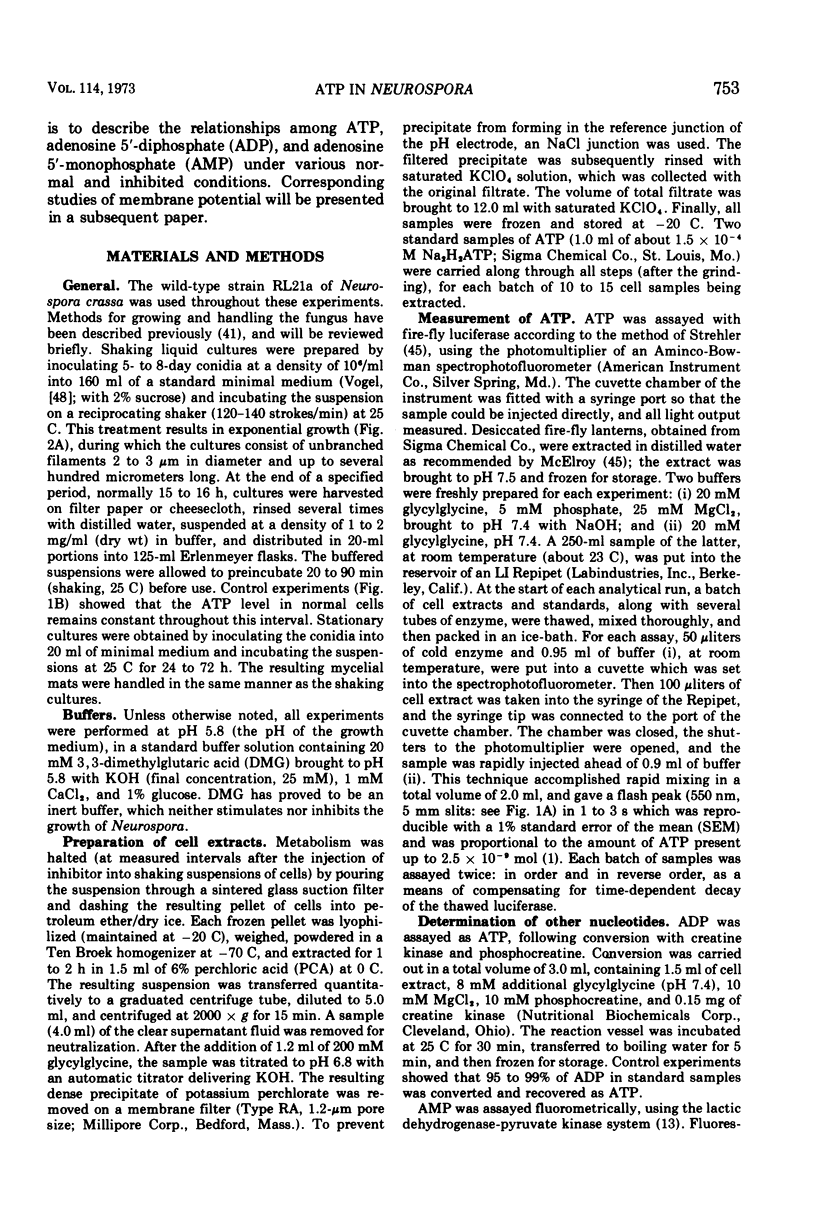
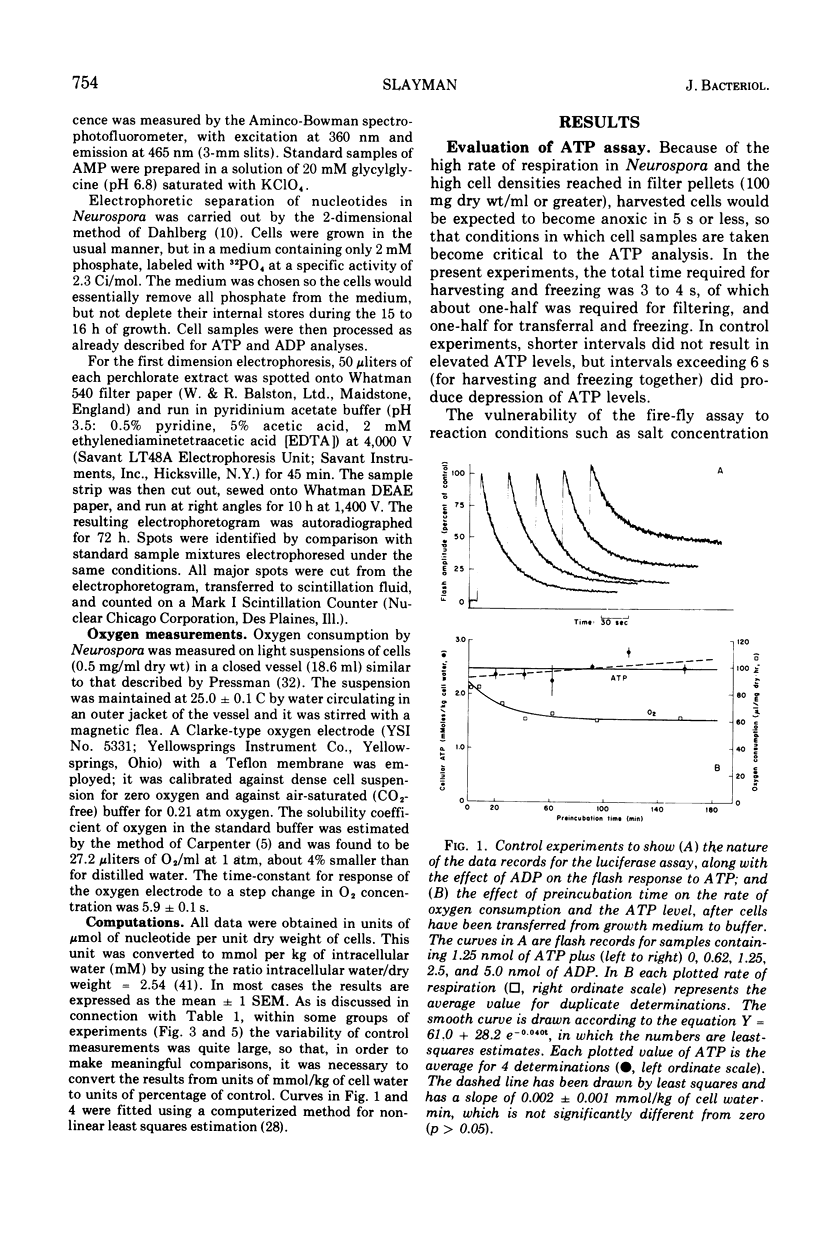
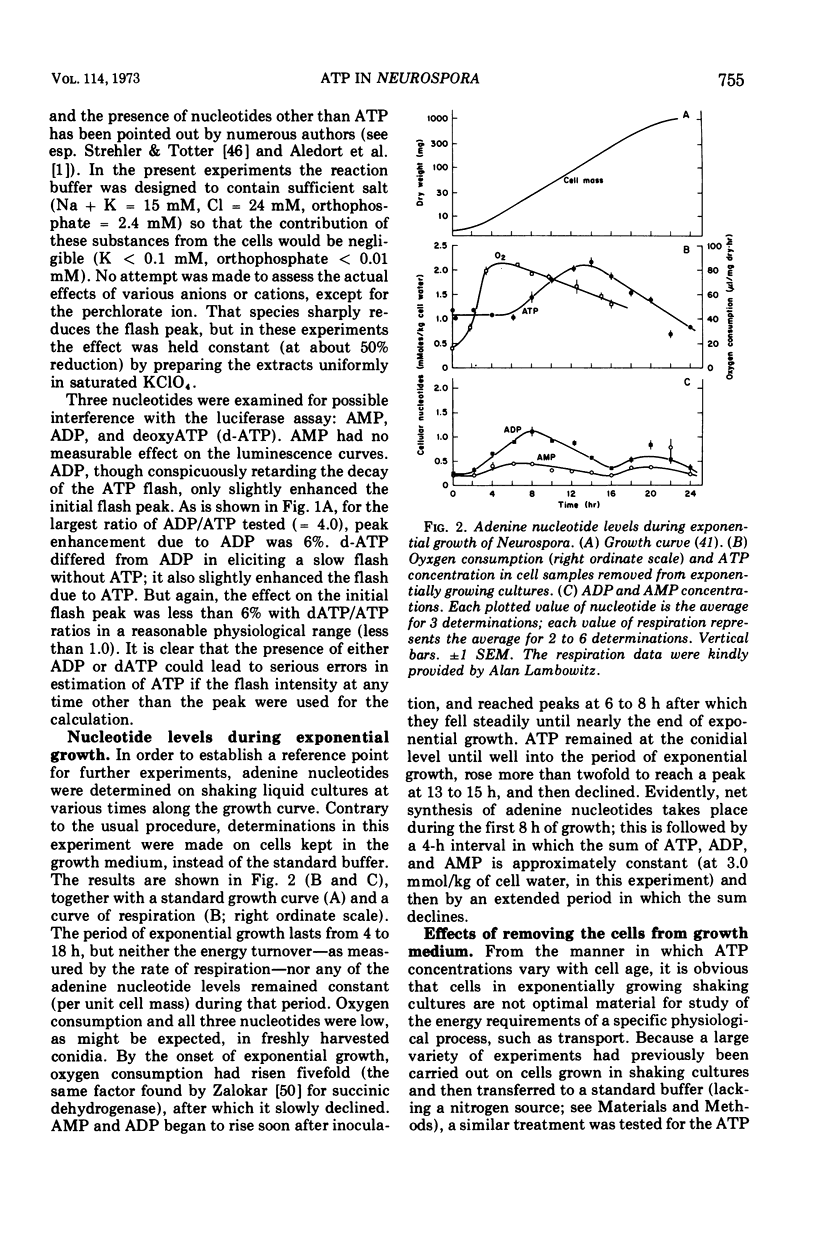
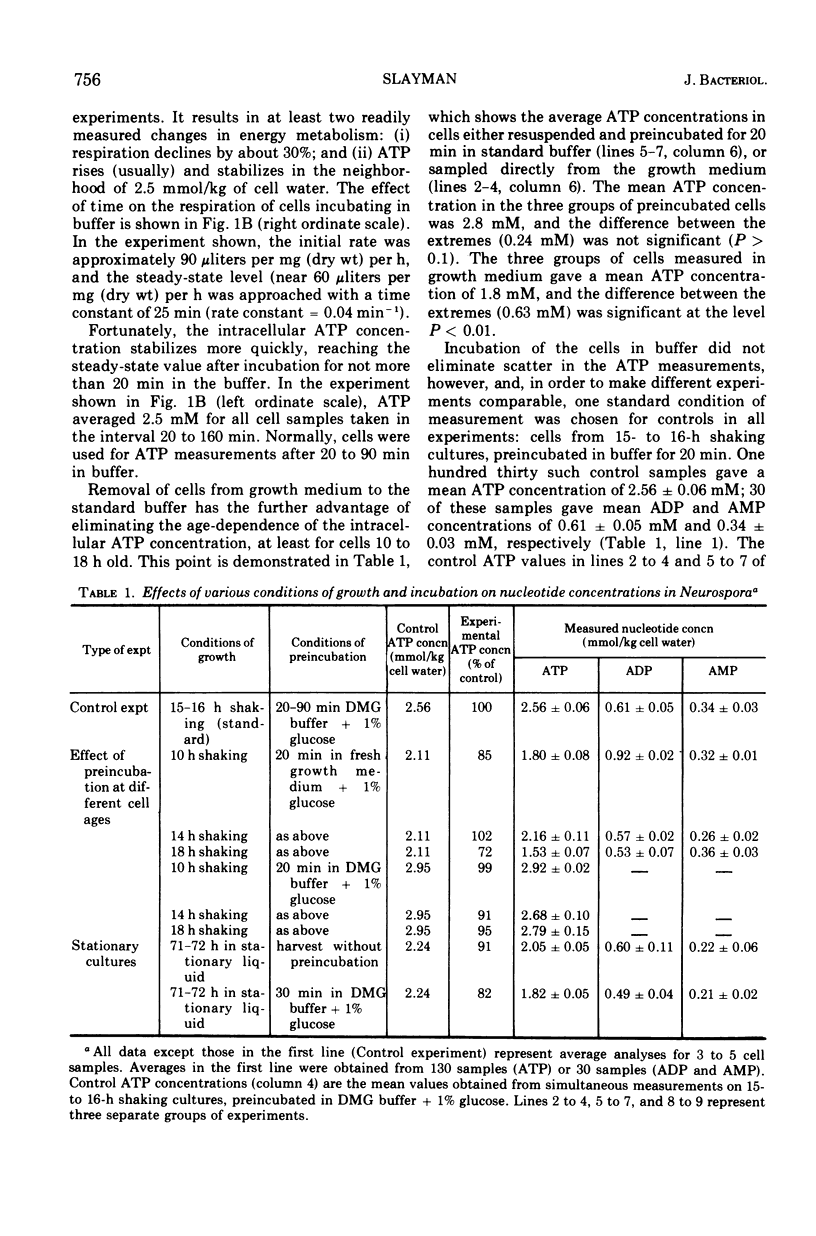
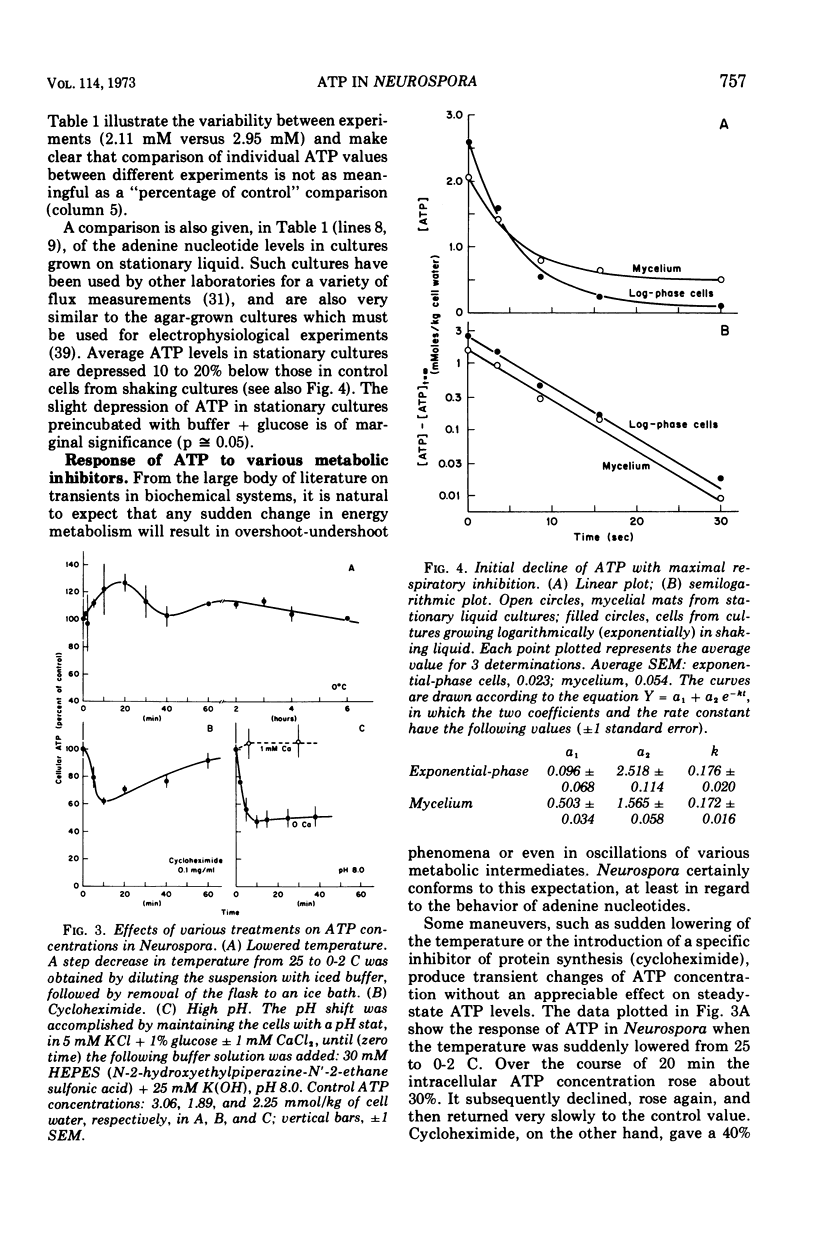
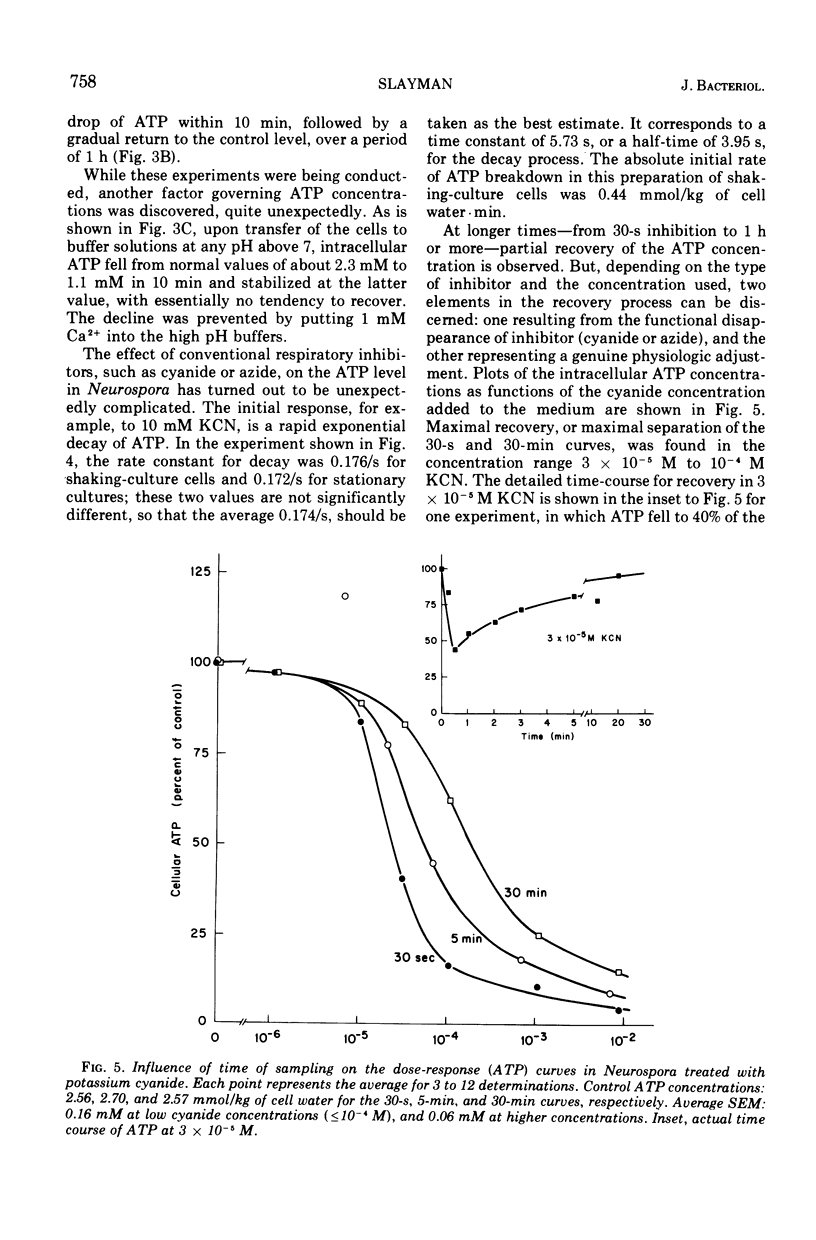
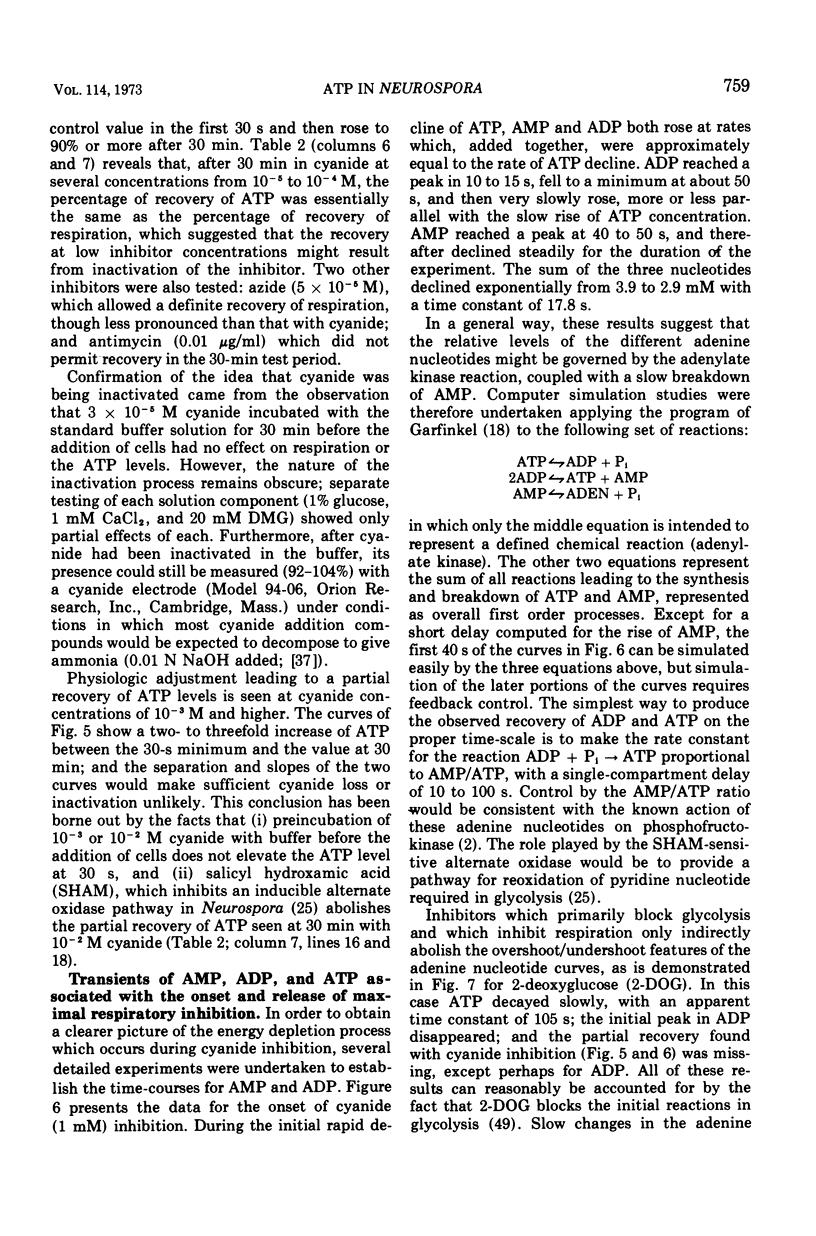
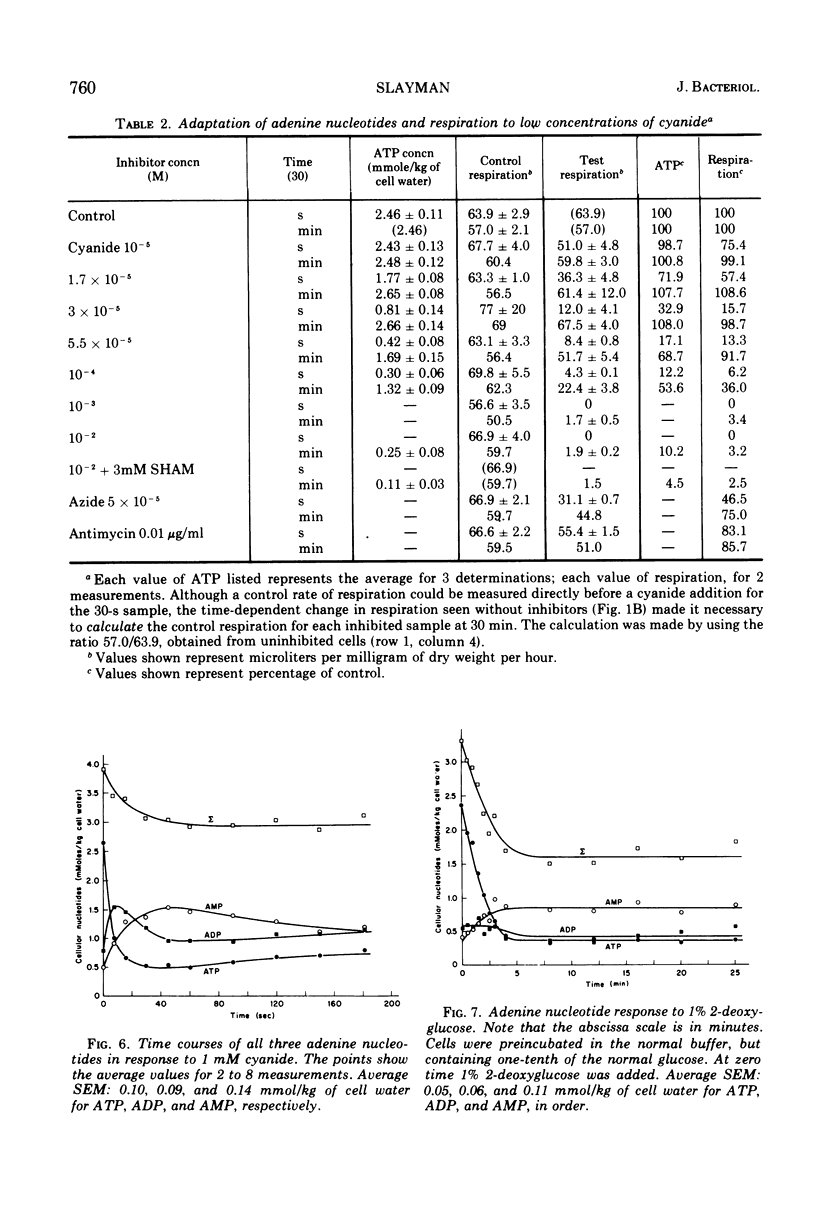
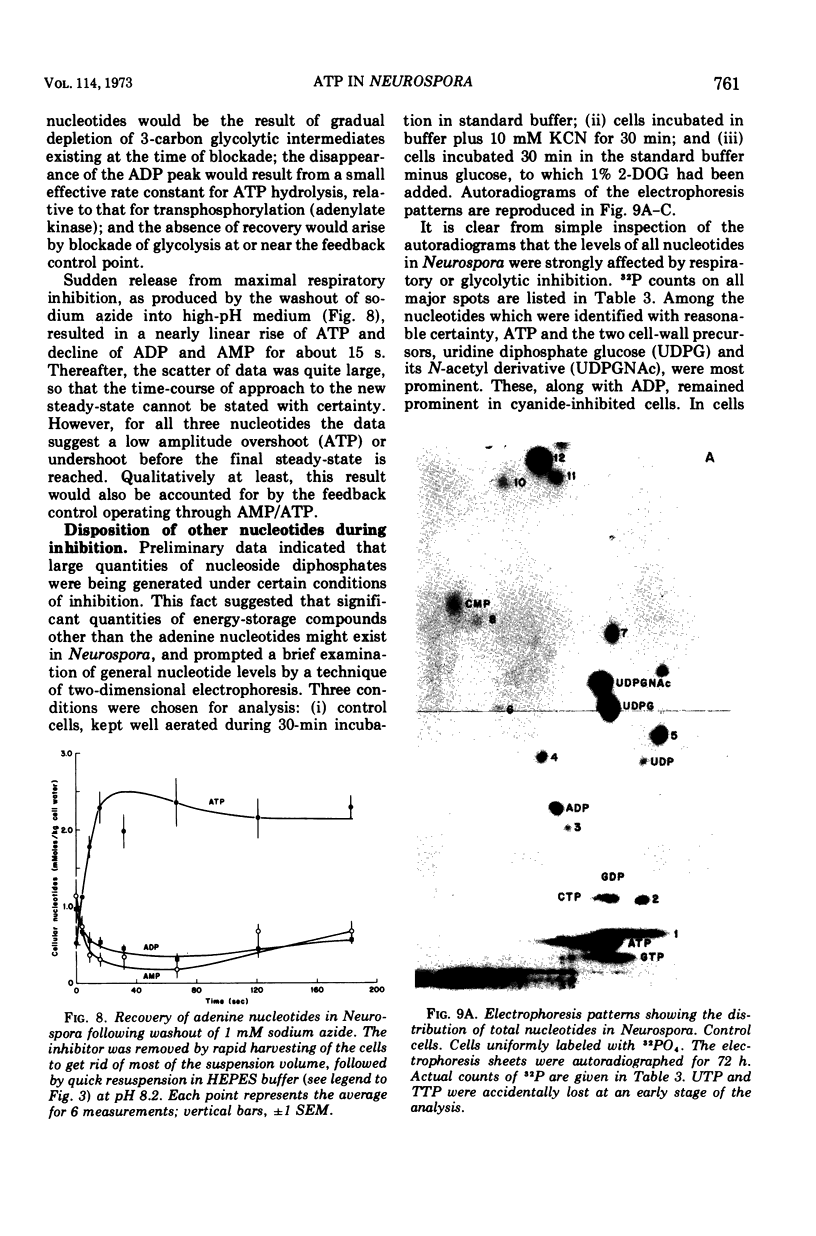
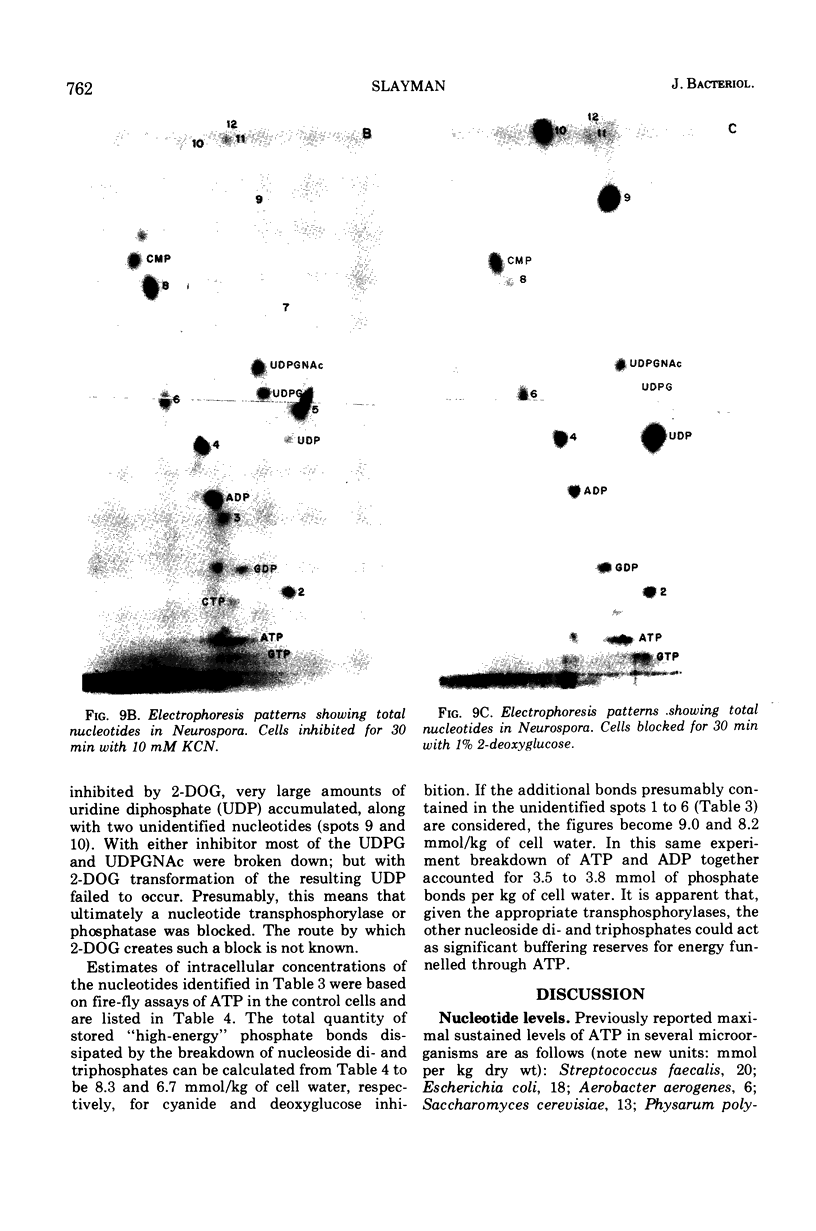
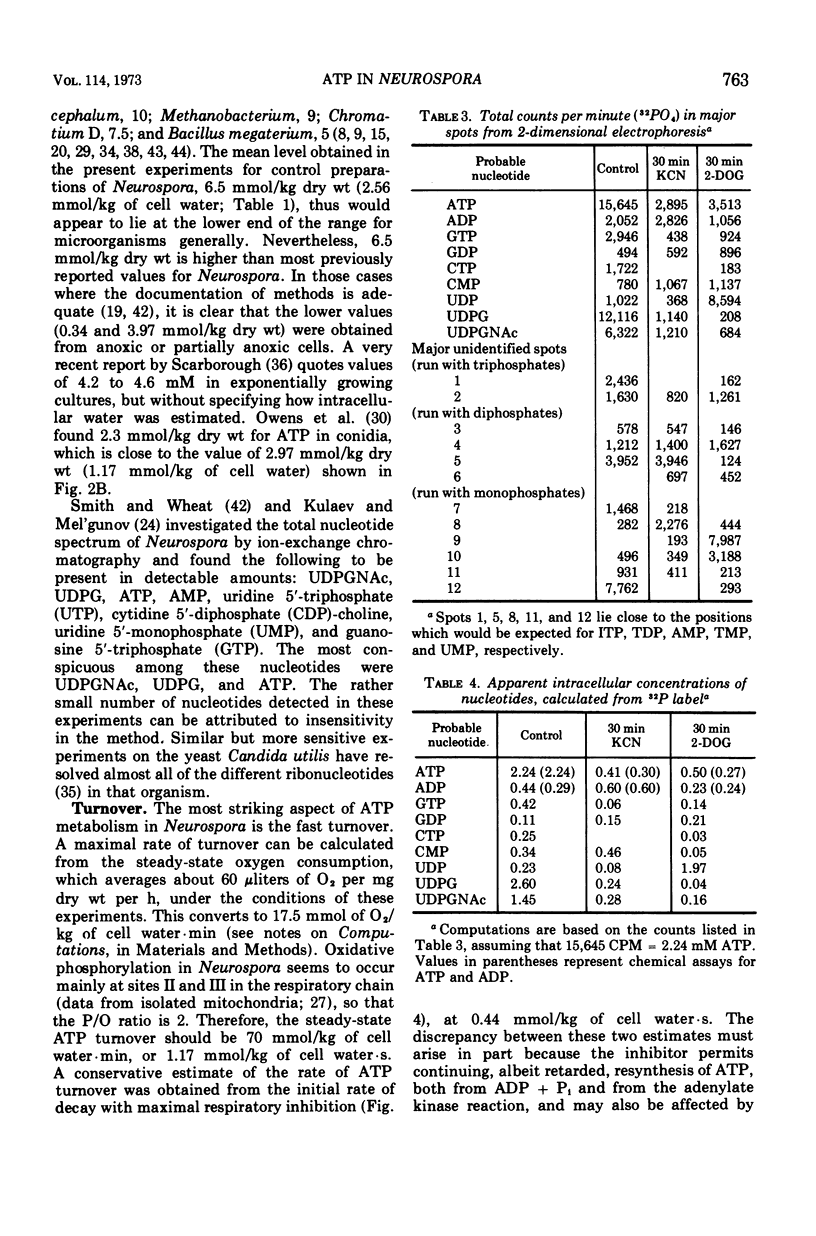
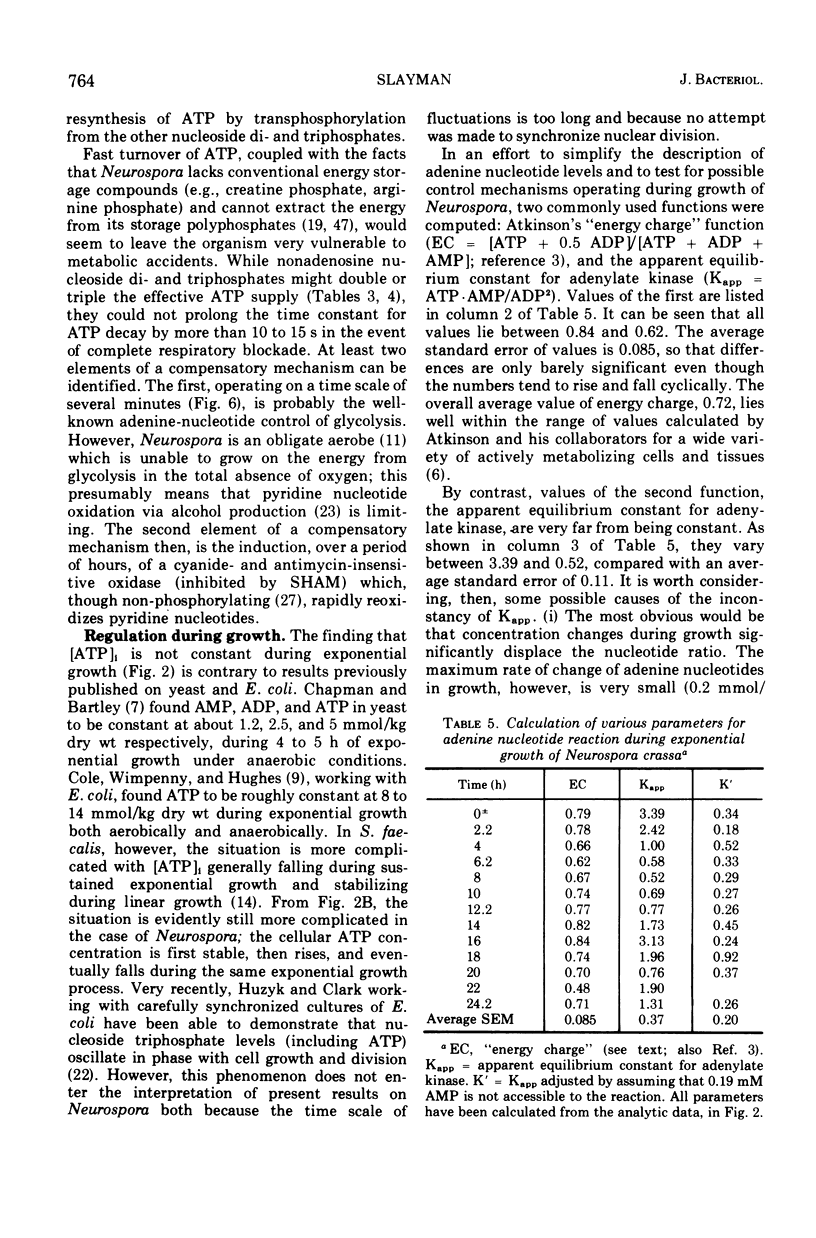
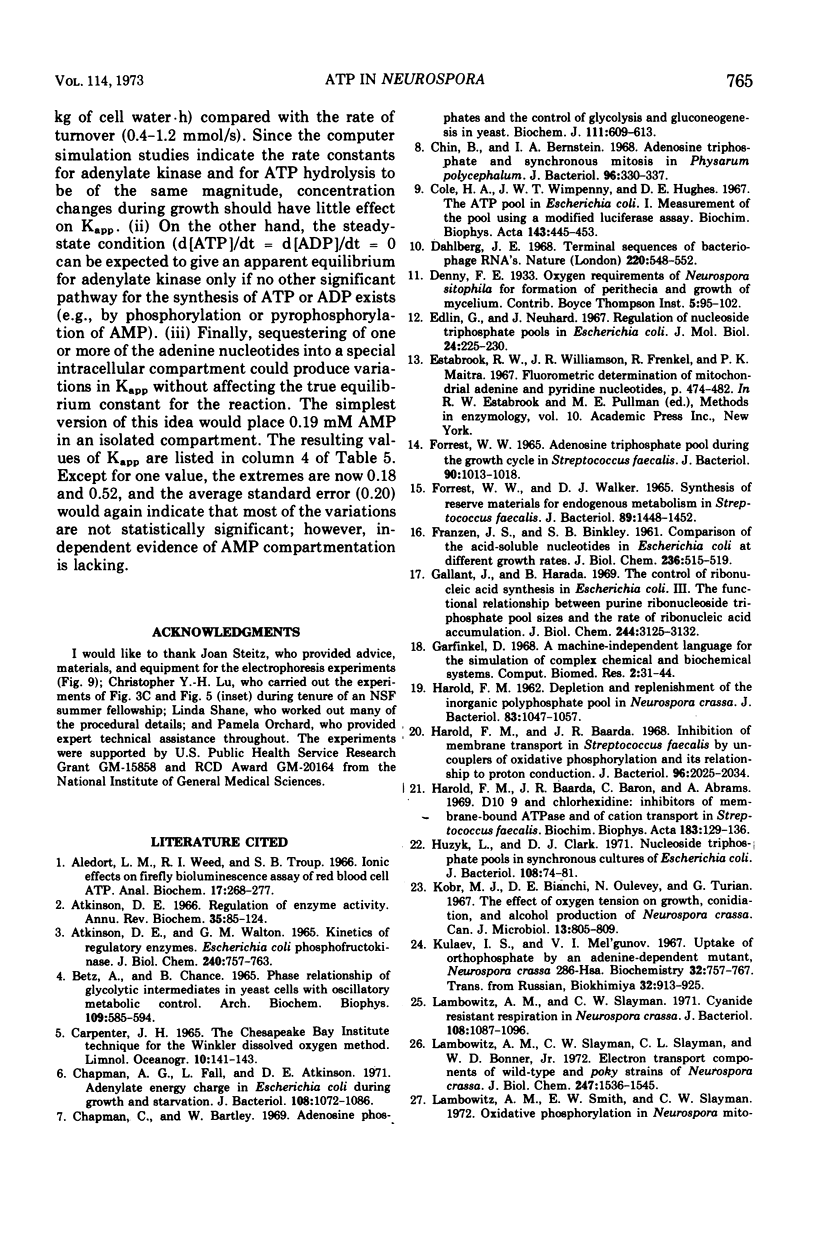
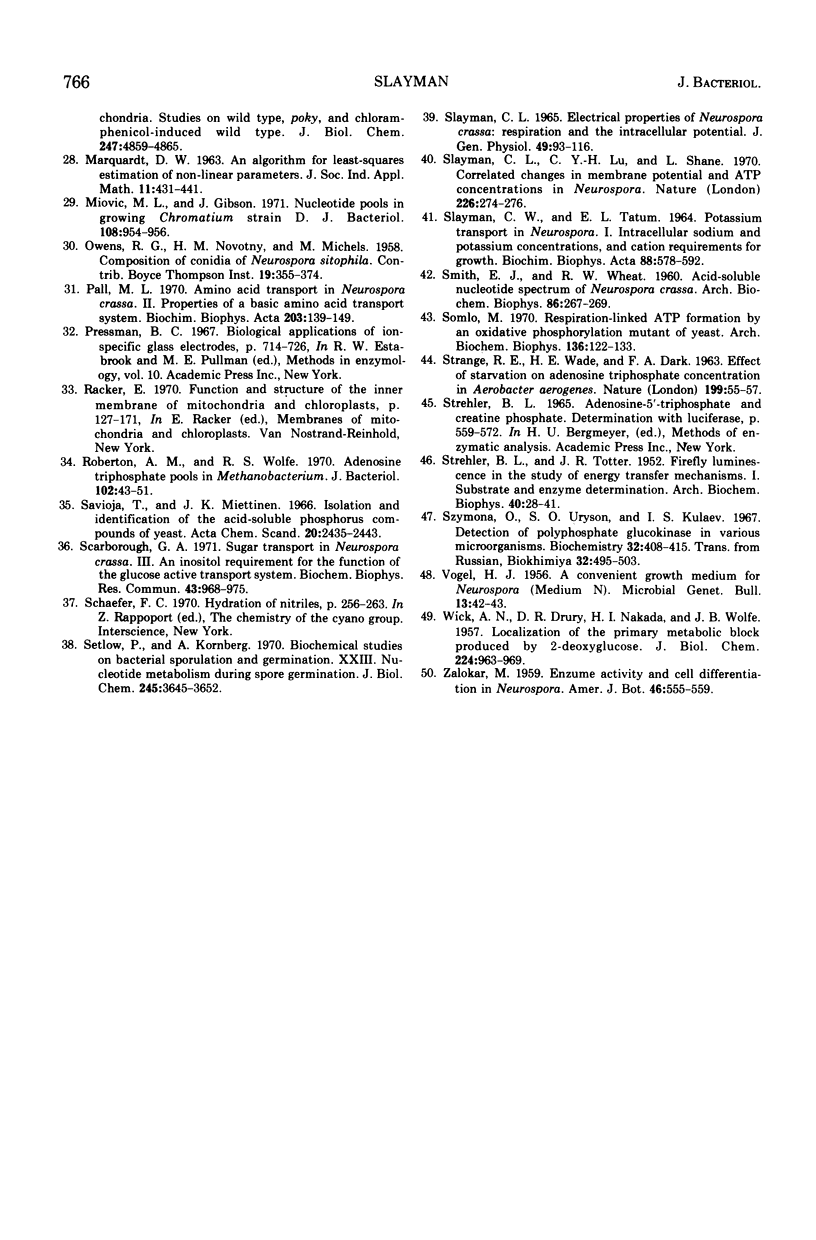
Images in this article
Selected References
These references are in PubMed. This may not be the complete list of references from this article.
- ATKINSON D. E., WALTON G. M. KINETICS OF REGULATORY ENZYMES. ESCHERICHIA COLI PHOSPHOFRUCTOKINASE. J Biol Chem. 1965 Feb;240:757–763. [PubMed] [Google Scholar]
- Aledort L. M., Weed R. I., Troup S. B. Ionic effects on firefly bioluminescence assay of red blood cell ATP. Anal Biochem. 1966 Nov;17(2):268–277. doi: 10.1016/0003-2697(66)90205-3. [DOI] [PubMed] [Google Scholar]
- BETZ A., CHANCE B. PHASE RELATIONSHIP OF GLYCOLYTIC INTERMEDIATES IN YEAST CELLS WITH OSCILLATORY METABOLIC CONTROL. Arch Biochem Biophys. 1965 Mar;109:585–594. doi: 10.1016/0003-9861(65)90404-2. [DOI] [PubMed] [Google Scholar]
- Chapman A. G., Fall L., Atkinson D. E. Adenylate energy charge in Escherichia coli during growth and starvation. J Bacteriol. 1971 Dec;108(3):1072–1086. doi: 10.1128/jb.108.3.1072-1086.1971. [DOI] [PMC free article] [PubMed] [Google Scholar]
- Chapman C., Bartley W. Adenosine phosphates and the control of glycolysis and gluconeogenesis in yeast. Biochem J. 1969 Mar;111(5):609–613. doi: 10.1042/bj1110609. [DOI] [PMC free article] [PubMed] [Google Scholar]
- Chin B., Bernstein I. A. Adenosine triphosphate and synchronous mitosis in Physarum polycephalum. J Bacteriol. 1968 Aug;96(2):330–337. doi: 10.1128/jb.96.2.330-337.1968. [DOI] [PMC free article] [PubMed] [Google Scholar]
- Cole H. A., Wimpenny J. W., Hughes D. E. The ATP pool in Escherichia coli. I. Measurement of the pool using modified luciferase assay. Biochim Biophys Acta. 1967;143(3):445–453. doi: 10.1016/0005-2728(67)90050-3. [DOI] [PubMed] [Google Scholar]
- Dahlberg J. E. Terminal sequences of bacteriophage RNAs. Nature. 1968 Nov 9;220(5167):548–552. doi: 10.1038/220548a0. [DOI] [PubMed] [Google Scholar]
- Edlin G., Neuhard J. Regulation of nucleoside triphosphate pools in Escherichia coli. J Mol Biol. 1967 Mar 14;24(2):225–230. doi: 10.1016/0022-2836(67)90328-2. [DOI] [PubMed] [Google Scholar]
- FORREST W. W., WALKER D. J. SYNTHESIS OF RESERVE MATERIALS FOR ENDOGENOUS METABOLISM IN STREPTOCOCCUS FAECALIS. J Bacteriol. 1965 Jun;89:1448–1452. doi: 10.1128/jb.89.6.1448-1452.1965. [DOI] [PMC free article] [PubMed] [Google Scholar]
- FRANZEN J. S., BINKLEY S. B. Comparison of the acid-soluble nucleotides in Escherichia coli at different growth rates. J Biol Chem. 1961 Feb;236:515–519. [PubMed] [Google Scholar]
- Forrest W. W. Adenosine triphosphate pool during the growth cycle in Streptococcus faecalis. J Bacteriol. 1965 Oct;90(4):1013–1018. doi: 10.1128/jb.90.4.1013-1018.1965. [DOI] [PMC free article] [PubMed] [Google Scholar]
- Gallant J., Harada B. The control of ribonucleic acid synthesis in Escherichia coli. 3. The functional relationship between purine ribonucleoside triphosphate pool sizes and the rate of ribonucleic acid accumulation. J Biol Chem. 1969 Jun 25;244(12):3125–3132. [PubMed] [Google Scholar]
- Garfinkel D. A machine-independent language for the simulation of complex chemical and biochemical systems. Comput Biomed Res. 1968 Aug;2(1):31–44. doi: 10.1016/0010-4809(68)90006-2. [DOI] [PubMed] [Google Scholar]
- HAROLD F. M. Depletion and replenishment of the inorganic polyphosphate pool in Neurospora crassa. J Bacteriol. 1962 May;83:1047–1057. doi: 10.1128/jb.83.5.1047-1057.1962. [DOI] [PMC free article] [PubMed] [Google Scholar]
- Harold F. M., Baarda J. R., Baron C., Abrams A. Dio 9 and chlorhexidine: inhibitors of membrane-bound ATPase and of cation transport in Streptococcus faecalis. Biochim Biophys Acta. 1969 Jun 3;183(1):129–136. doi: 10.1016/0005-2736(69)90136-9. [DOI] [PubMed] [Google Scholar]
- Harold F. M., Baarda J. R. Inhibition of membrane transport in Streptococcus faecalis by uncouplers of oxidative phosphorylation and its relationship to proton conduction. J Bacteriol. 1968 Dec;96(6):2025–2034. doi: 10.1128/jb.96.6.2025-2034.1968. [DOI] [PMC free article] [PubMed] [Google Scholar]
- Huzyk L., Clark D. J. Nucleoside triphosphate pools in synchronous cultures of Escherichia coli. J Bacteriol. 1971 Oct;108(1):74–81. doi: 10.1128/jb.108.1.74-81.1971. [DOI] [PMC free article] [PubMed] [Google Scholar]
- Kobr M. J., Bianchi D. E., Oulevey N., Turian G. The effect of oxygen tension on growth, conidiation, and alcohol production of Neurospora crassa. Can J Microbiol. 1967 Jul;13(7):805–809. doi: 10.1139/m67-106. [DOI] [PubMed] [Google Scholar]
- Lambowitz A. M., Slayman C. W. Cyanide-resistant respiration in Neurospora crassa. J Bacteriol. 1971 Dec;108(3):1087–1096. doi: 10.1128/jb.108.3.1087-1096.1971. [DOI] [PMC free article] [PubMed] [Google Scholar]
- Lambowitz A. M., Slayman C. W., Slayman C. L., Bonner W. D., Jr The electron transport components of wild type and poky strains of Neurospora crassa. J Biol Chem. 1972 Mar 10;247(5):1536–1545. [PubMed] [Google Scholar]
- Miović M. L., Gibson J. Nucleotide pools in growing Chromatium strain D. J Bacteriol. 1971 Nov;108(2):954–956. doi: 10.1128/jb.108.2.954-956.1971. [DOI] [PMC free article] [PubMed] [Google Scholar]
- Pall M. L. Amino acid transport in Neurospora crassa. II. Properties of a basic amino acid transport system. Biochim Biophys Acta. 1970 Mar 17;203(1):139–149. doi: 10.1016/0005-2736(70)90044-1. [DOI] [PubMed] [Google Scholar]
- Roberton A. M., Wolfe R. S. Adenosine triphosphate pools in Methanobacterium. J Bacteriol. 1970 Apr;102(1):43–51. doi: 10.1128/jb.102.1.43-51.1970. [DOI] [PMC free article] [PubMed] [Google Scholar]
- SLAYMAN C. W., TATUM E. L. POTASSIUM TRANSPORT IN NEUROSPORA. I. INTRACELLULAR SODIUM AND POTASSIUM CONCENTRATIONS, AND CATION REQUIREMENTS FOR GROWTH. Biochim Biophys Acta. 1964 Nov 29;88:578–592. [PubMed] [Google Scholar]
- SMITH E. J., WHEAT R. W. Acid-soluble nucleotide spectrum of Neurospora crassa. Arch Biochem Biophys. 1960 Feb;86:267–269. doi: 10.1016/0003-9861(60)90416-1. [DOI] [PubMed] [Google Scholar]
- STRANGE R. E., WADE H. E., DARK F. A. EFFECT OF STARVATION ON ADENOSINE TRIPHOSPHATE CONCENTRATION IN AEROBACTER AEROGENES. Nature. 1963 Jul 6;199:55–57. doi: 10.1038/199055a0. [DOI] [PubMed] [Google Scholar]
- STREHLER B. L., TOTTER J. R. Firefly luminescence in the study of energy transfer mechanisms. I. Substrate and enzyme determination. Arch Biochem Biophys. 1952 Sep;40(1):28–41. doi: 10.1016/0003-9861(52)90070-2. [DOI] [PubMed] [Google Scholar]
- Savioja T., Miettinen J. K. Isolation and identification of the acid-soluble phosphorus compounds of yeast. Acta Chem Scand. 1966;20(9):2435–2443. doi: 10.3891/acta.chem.scand.20-2435. [DOI] [PubMed] [Google Scholar]
- Scarborough G. A. Sugar transport in Neurospora crassa. 3. An inositol requirement for the function of the glucose active transport system. Biochem Biophys Res Commun. 1971 Jun 4;43(5):968–975. doi: 10.1016/0006-291x(71)90557-2. [DOI] [PubMed] [Google Scholar]
- Setlow P., Kornberg A. Biochemical studies of bacterial sporulation and germination. 23. Nucleotide metabolism during spore germination. J Biol Chem. 1970 Jul 25;245(14):3645–3652. [PubMed] [Google Scholar]
- Slayman C. L. Electrical properties of Neurospora crassa. Respiration and the intracellular potential. J Gen Physiol. 1965 Sep;49(1):93–116. doi: 10.1085/jgp.49.1.93. [DOI] [PMC free article] [PubMed] [Google Scholar]
- Slayman C. L., Lu C. Y., Shane L. Correlated changes in membrane potential and ATP concentrations in Neurospora. Nature. 1970 Apr 18;226(5242):274–276. doi: 10.1038/226274a0. [DOI] [PubMed] [Google Scholar]
- Somlo M. Respiration-linked ATP formation by an "oxidative phosphorylation mutant" of yeast. Arch Biochem Biophys. 1970 Jan;136(1):122–133. doi: 10.1016/0003-9861(70)90334-6. [DOI] [PubMed] [Google Scholar]
- WICK A. N., DRURY D. R., NAKADA H. I., WOLFE J. B. Localization of the primary metabolic block produced by 2-deoxyglucose. J Biol Chem. 1957 Feb;224(2):963–969. [PubMed] [Google Scholar]