Abstract
The orientation and morphology of the endothelium lining the cardiovascular system may result from hemodynamic forces acting on the endothelial cells. To investigate the flow effects at the membrane level, we have examined the variations of the fluorescence intensity of two membrane-sensitive dyes, merocyanine 540 and bis(1,3-diethylthiobarbiturate)trimethineoxonol, (i) as a function of flow shear stress and (ii) with the onset or cessation of the flow. We found a time-dependent decrease in fluorescence intensity with the onset of the flow with an exponential approach to steady state of the order of 1 min. The process is reversible; when the flow is stopped the fluorescence intensity returns to its original value. The polarization of the endothelial cell membranes or, more precisely, the amplitude of the fluorescence intensity responses is an increasing function of the shear stress (up to 120 dynes/cm2). Assuming the equilibrium potential for K+ is more hyperpolarized than the resting potential and using valinomycin, we have deduced from the sign of the ionophore effects that the flow hyperpolarizes the endothelial cell membrane.
Full text
PDF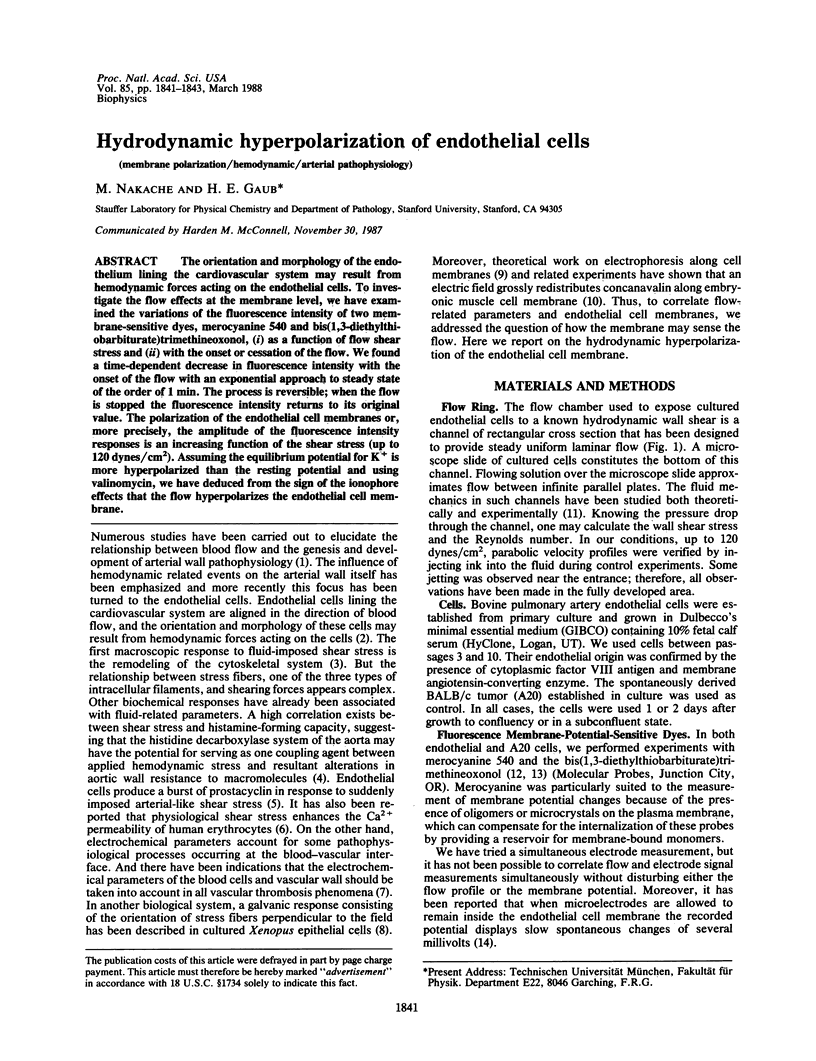
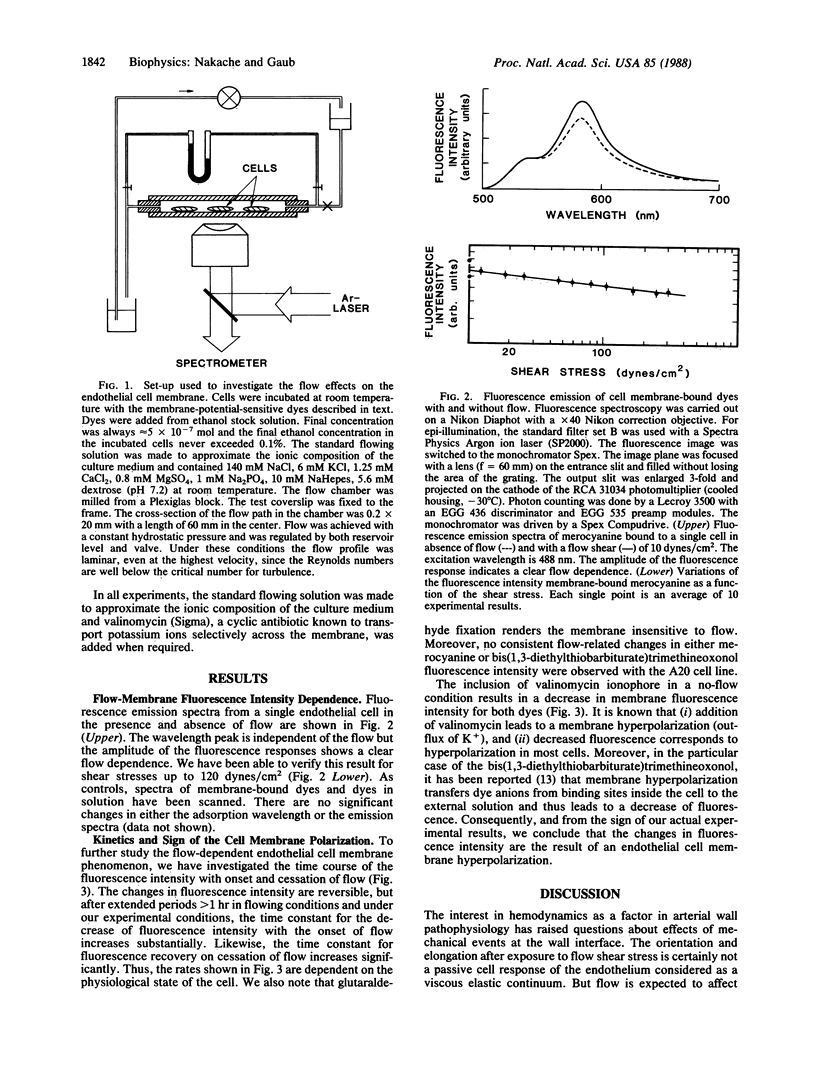
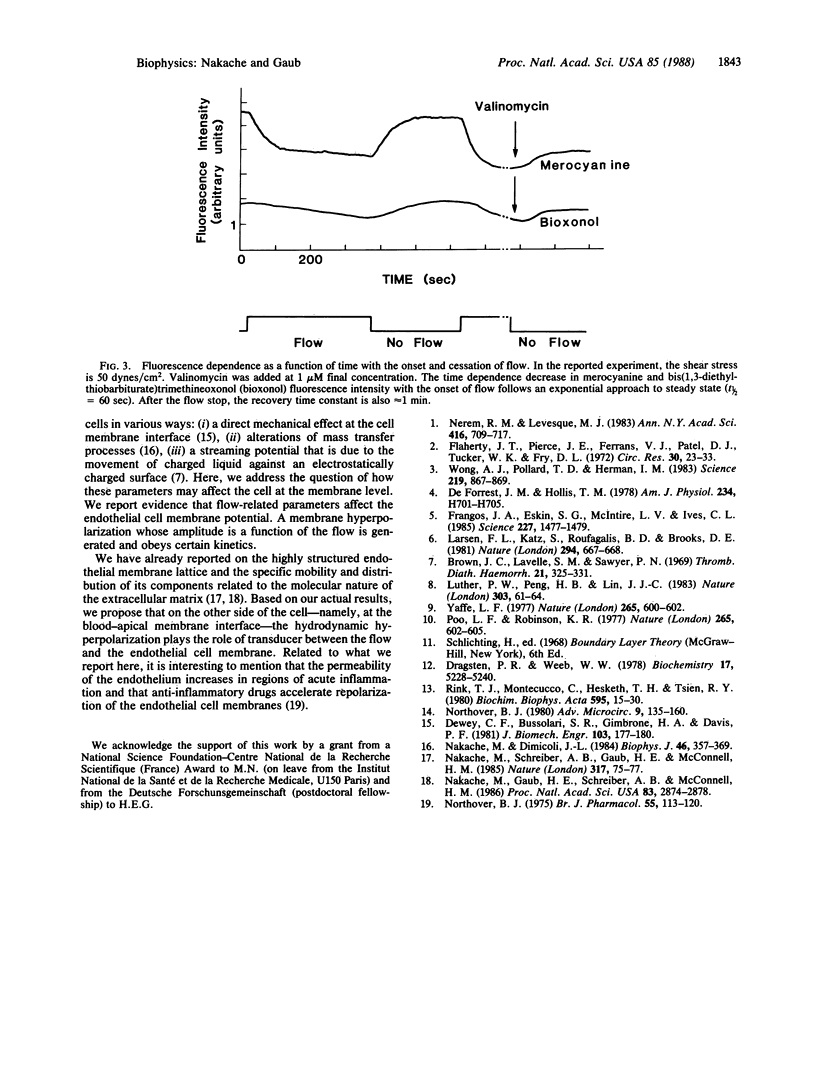
Selected References
These references are in PubMed. This may not be the complete list of references from this article.
- Brown J. C., Lavelle S. M., Sawyer P. N. Relationship between electrical and spontaneous thrombosis. Coagulation inhibition of intrinsic system. Thromb Diath Haemorrh. 1969 Apr 30;21(2):325–331. [PubMed] [Google Scholar]
- DeForrest J. M., Hollis T. M. Shear stress and aortic histamine synthesis. Am J Physiol. 1978 Jun;234(6):H701–H705. doi: 10.1152/ajpheart.1978.234.6.H701. [DOI] [PubMed] [Google Scholar]
- Dewey C. F., Jr, Bussolari S. R., Gimbrone M. A., Jr, Davies P. F. The dynamic response of vascular endothelial cells to fluid shear stress. J Biomech Eng. 1981 Aug;103(3):177–185. doi: 10.1115/1.3138276. [DOI] [PubMed] [Google Scholar]
- Dragsten P. R., Webb W. W. Mechanism of the membrane potential sensitivity of the fluorescent membrane probe merocyanine 540. Biochemistry. 1978 Nov 28;17(24):5228–5240. doi: 10.1021/bi00617a024. [DOI] [PubMed] [Google Scholar]
- Flaherty J. T., Pierce J. E., Ferrans V. J., Patel D. J., Tucker W. K., Fry D. L. Endothelial nuclear patterns in the canine arterial tree with particular reference to hemodynamic events. Circ Res. 1972 Jan;30(1):23–33. doi: 10.1161/01.res.30.1.23. [DOI] [PubMed] [Google Scholar]
- Frangos J. A., Eskin S. G., McIntire L. V., Ives C. L. Flow effects on prostacyclin production by cultured human endothelial cells. Science. 1985 Mar 22;227(4693):1477–1479. doi: 10.1126/science.3883488. [DOI] [PubMed] [Google Scholar]
- Jaffe L. F. Electrophoresis along cell membranes. Nature. 1977 Feb 17;265(5595):600–602. doi: 10.1038/265600a0. [DOI] [PubMed] [Google Scholar]
- Larsen F. L., Katz S., Roufogalis B. D., Brooks D. E. Physiological shear stresses enhance the Ca2+ permeability of human erythrocytes. Nature. 1981 Dec 17;294(5842):667–668. doi: 10.1038/294667a0. [DOI] [PubMed] [Google Scholar]
- Luther P. W., Peng H. B., Lin J. J. Changes in cell shape and actin distribution induced by constant electric fields. Nature. 1983 May 5;303(5912):61–64. doi: 10.1038/303061a0. [DOI] [PubMed] [Google Scholar]
- Nakache M., Dimicoli J. L. Topological analysis of wall mass transport using a luminescent immobilized enzymatic system. Biophys J. 1984 Sep;46(3):357–369. doi: 10.1016/S0006-3495(84)84032-1. [DOI] [PMC free article] [PubMed] [Google Scholar]
- Nakache M., Gaub H. E., Schreiber A. B., McConnell H. M. Topological and modulated distribution of surface markers on endothelial cells. Proc Natl Acad Sci U S A. 1986 May;83(9):2874–2878. doi: 10.1073/pnas.83.9.2874. [DOI] [PMC free article] [PubMed] [Google Scholar]
- Nakache M., Schreiber A. B., Gaub H., McConnell H. M. Heterogeneity of membrane phospholipid mobility in endothelial cells depends on cell substrate. Nature. 1985 Sep 5;317(6032):75–77. doi: 10.1038/317075a0. [DOI] [PubMed] [Google Scholar]
- Nerem R. M., Levesque M. J. Fluid dynamics as a factor in the localization of atherogenesis. Ann N Y Acad Sci. 1983;416:709–719. doi: 10.1111/j.1749-6632.1983.tb35222.x. [DOI] [PubMed] [Google Scholar]
- Northover B. J. Effect of anti-inflammatory drugs on the membrane potential of vascular endothelial cells in vitro. Br J Pharmacol. 1975 Jan;53(1):113–120. doi: 10.1111/j.1476-5381.1975.tb07337.x. [DOI] [PMC free article] [PubMed] [Google Scholar]
- Poo M., Robinson K. R. Electrophoresis of concanavalin A receptors along embryonic muscle cell membrane. Nature. 1977 Feb 17;265(5595):602–605. doi: 10.1038/265602a0. [DOI] [PubMed] [Google Scholar]
- Rink T. J., Montecucco C., Hesketh T. R., Tsien R. Y. Lymphocyte membrane potential assessed with fluorescent probes. Biochim Biophys Acta. 1980;595(1):15–30. doi: 10.1016/0005-2736(80)90243-6. [DOI] [PubMed] [Google Scholar]
- Wong A. J., Pollard T. D., Herman I. M. Actin filament stress fibers in vascular endothelial cells in vivo. Science. 1983 Feb 18;219(4586):867–869. doi: 10.1126/science.6681677. [DOI] [PubMed] [Google Scholar]