Abstract
A mutant of Escherichia coli strain CanR 22 has been isolated which is resistant to growth inhibition by canavanine, an analogue of arginine. The properties of this strain and of another canavanine-resistant mutant, JC182-5 (isolated by Celis et al. [5]), were studied. The mutation is pleiotropic in that it results in a reduction in the activity of two distinct permeases, the arginine-specific and lysine-arginine-ornithine transport systems. The lesion maps at min 56 of the E. coli linkage map, at or near the argP locus. Although strain CanR 22 excretes arginine, this excretion appears to result from reduced ability to concentrate arginine, rather than the loss of transport ability being the result of excretion. This conclusion is based on findings with a canavanine-resistant strain auxotrophic for arginine, which exhibits transport properties similar to those of the prototrophic strains. Additionally, growth in the presence of arginine or ornithine results in a repression of the activity of the two basic amino acid transport systems. Neither the arginine-specific nor the lysine-arginine-ornithine binding proteins of the mutant cells show significant alterations in terms of amount, physical properties, or kinetic parameters. These observations lead to the proposal of a model for the two basic amino acid transport systems in which two carrier proteins with different specificities interact with a common energy coupling mechanism. A lesion in the gene (or one of the genes) for this coupling mechanism can confer canavanine resistance.
Full text
PDF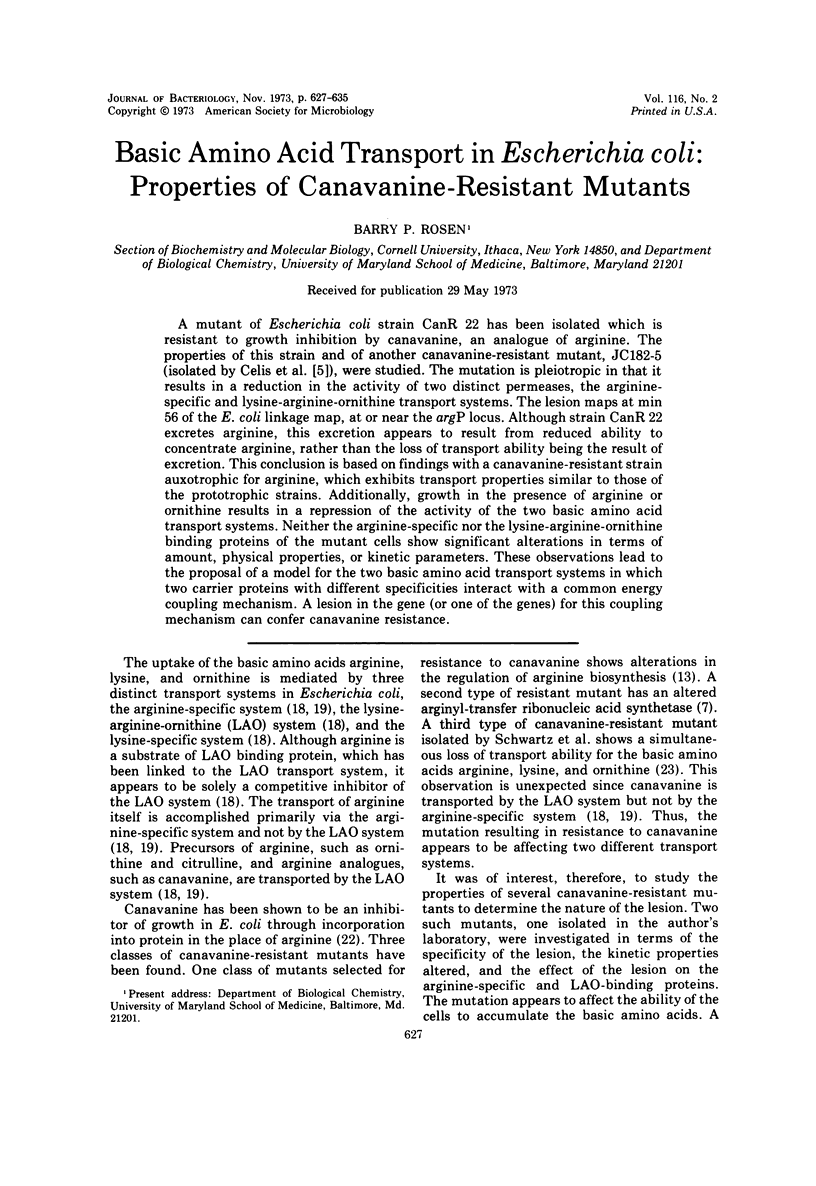
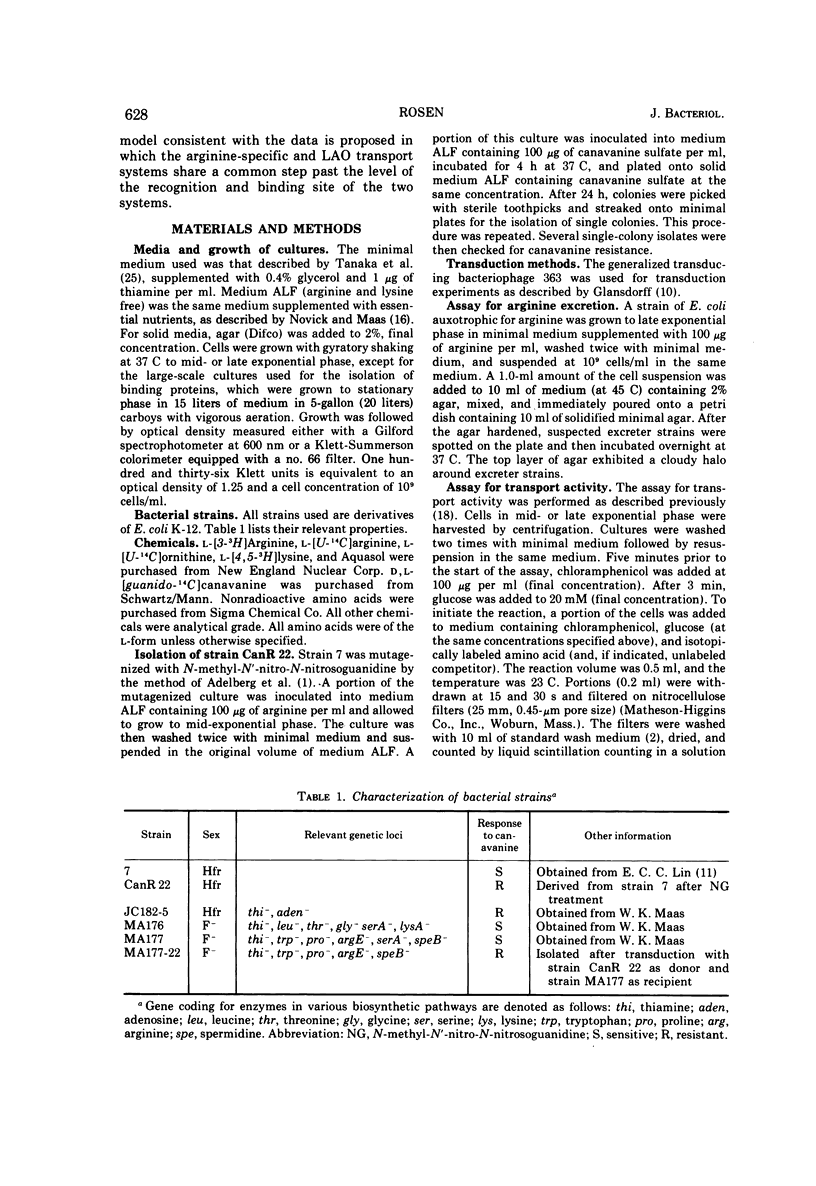
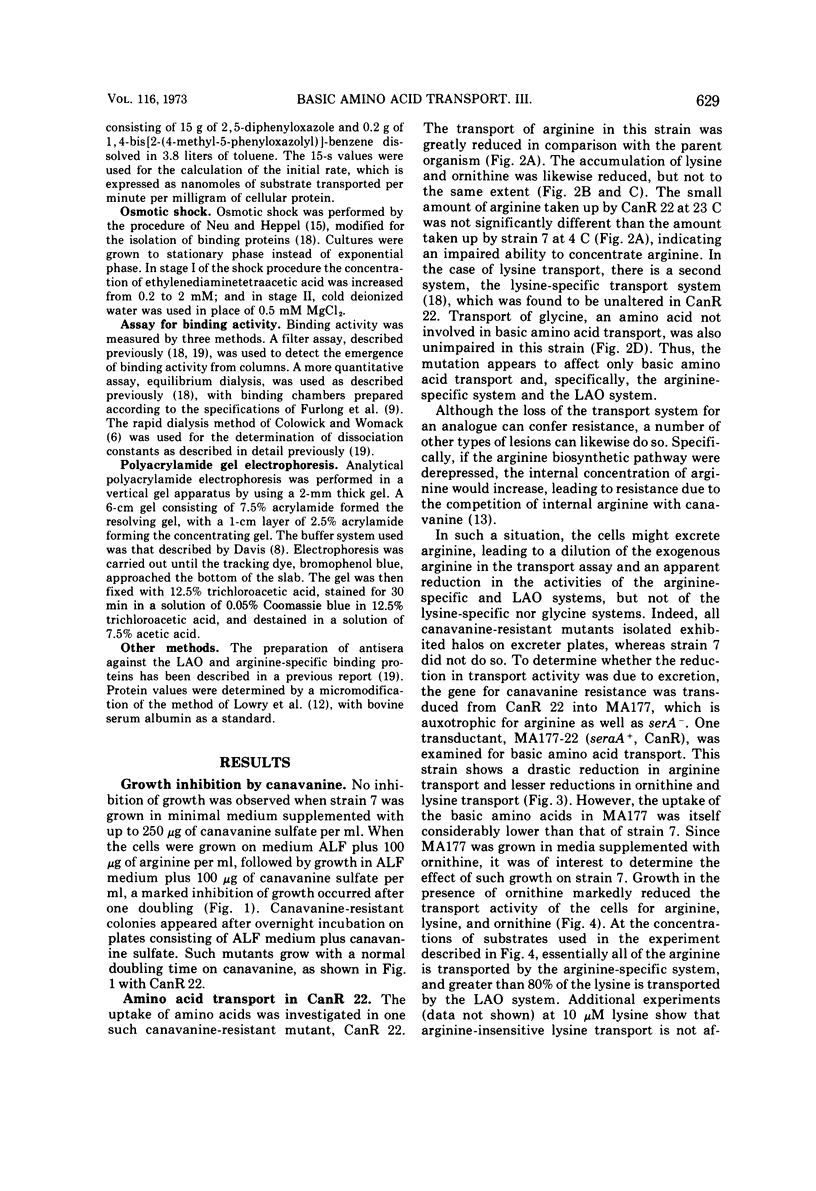
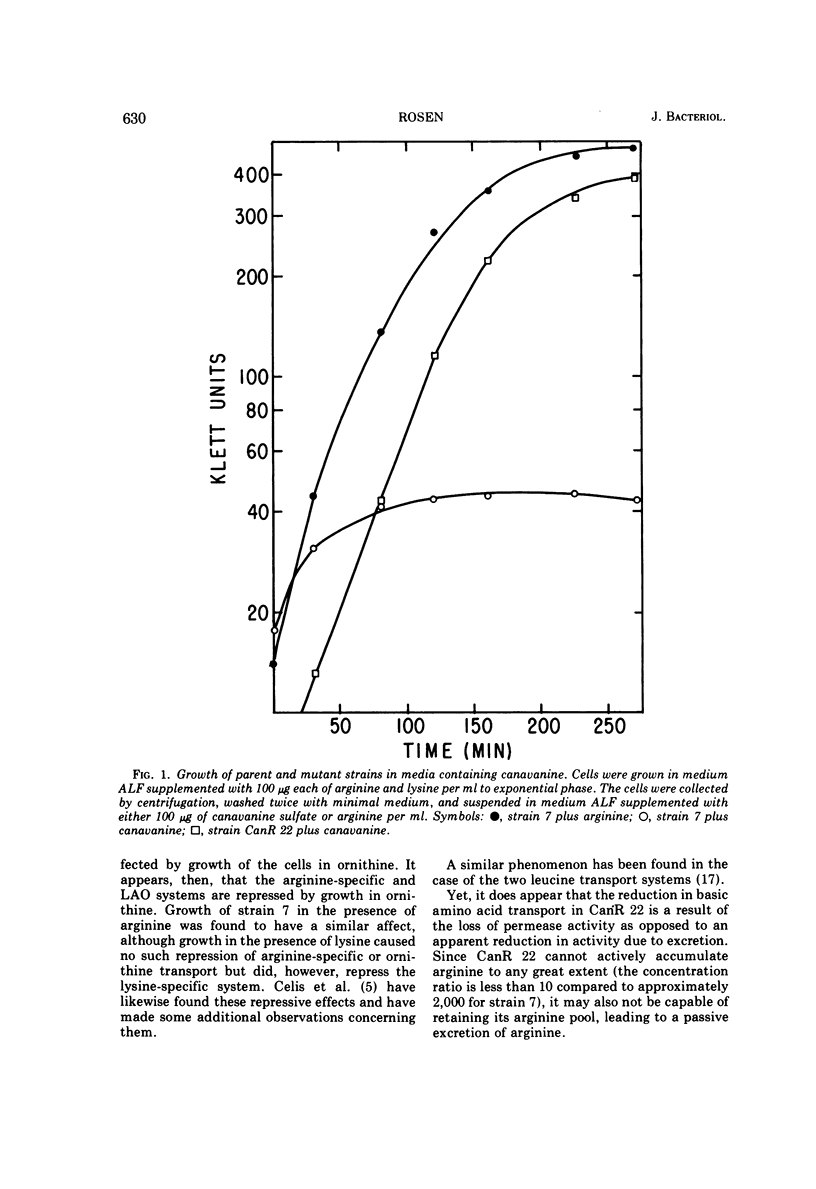
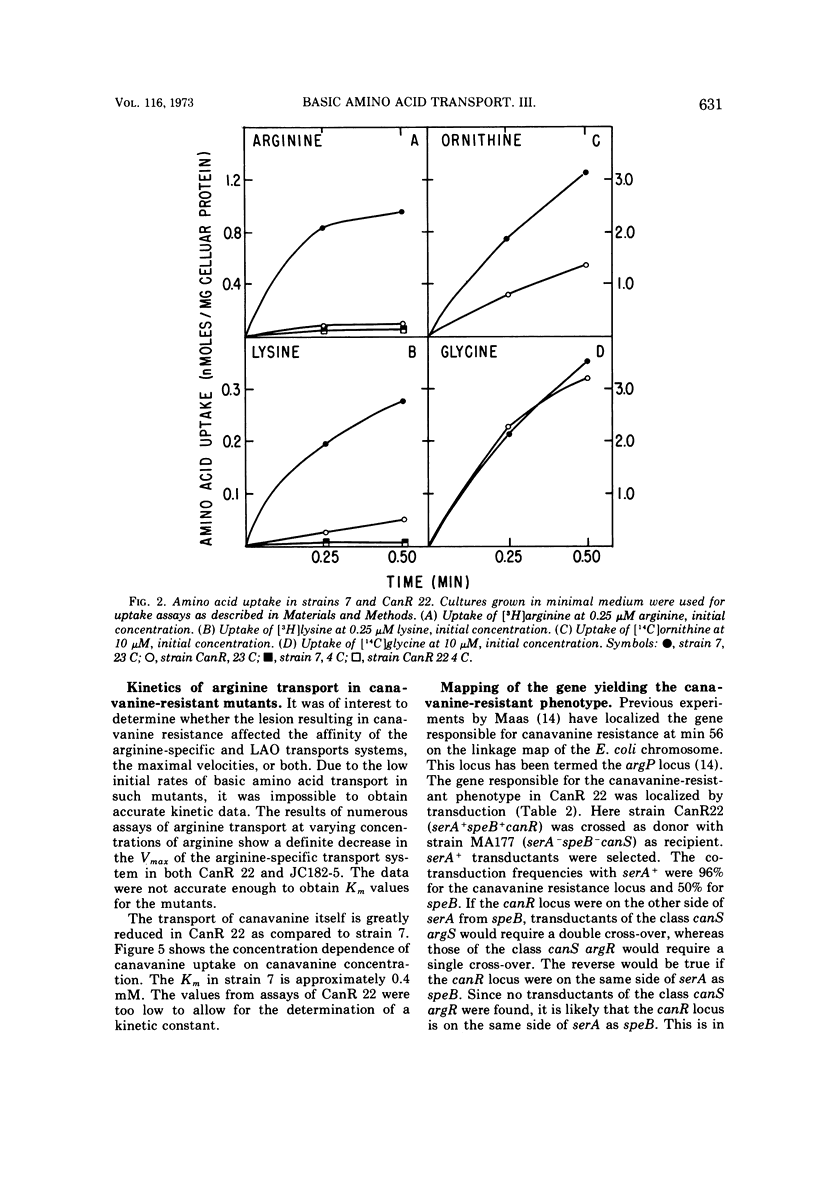
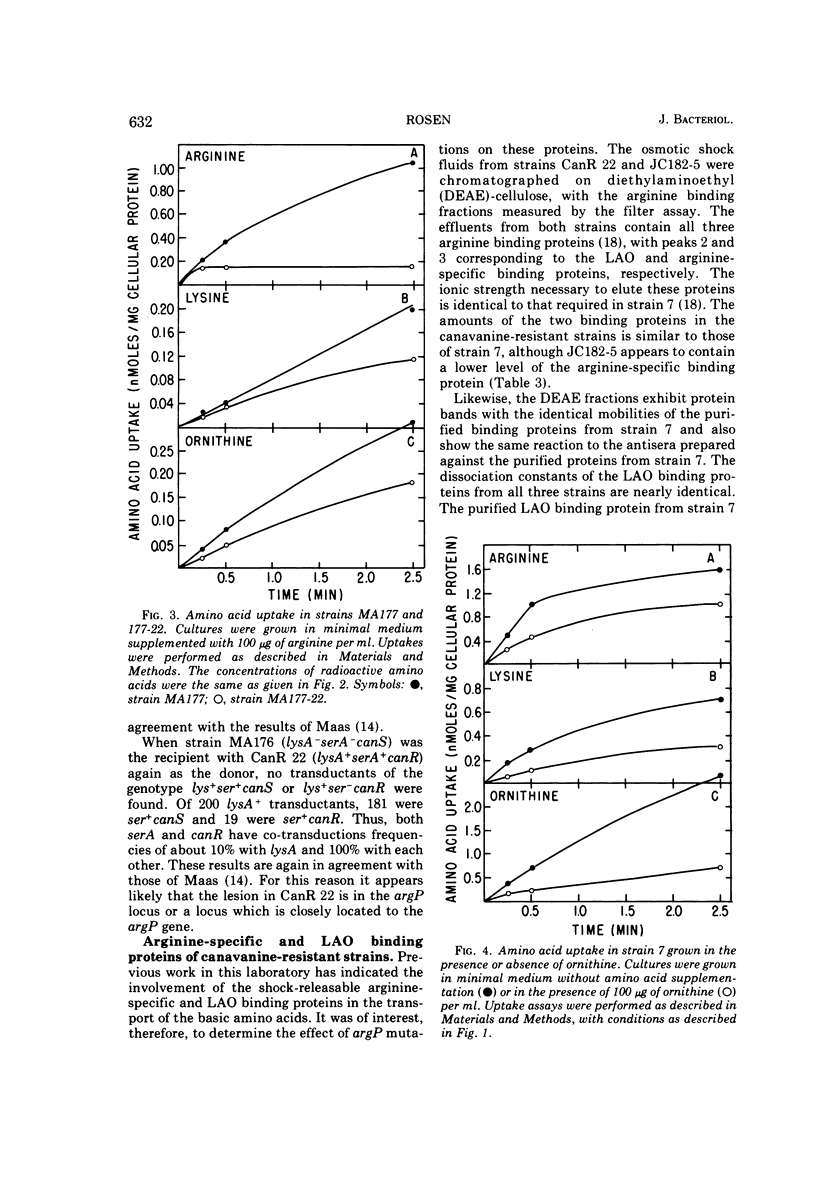
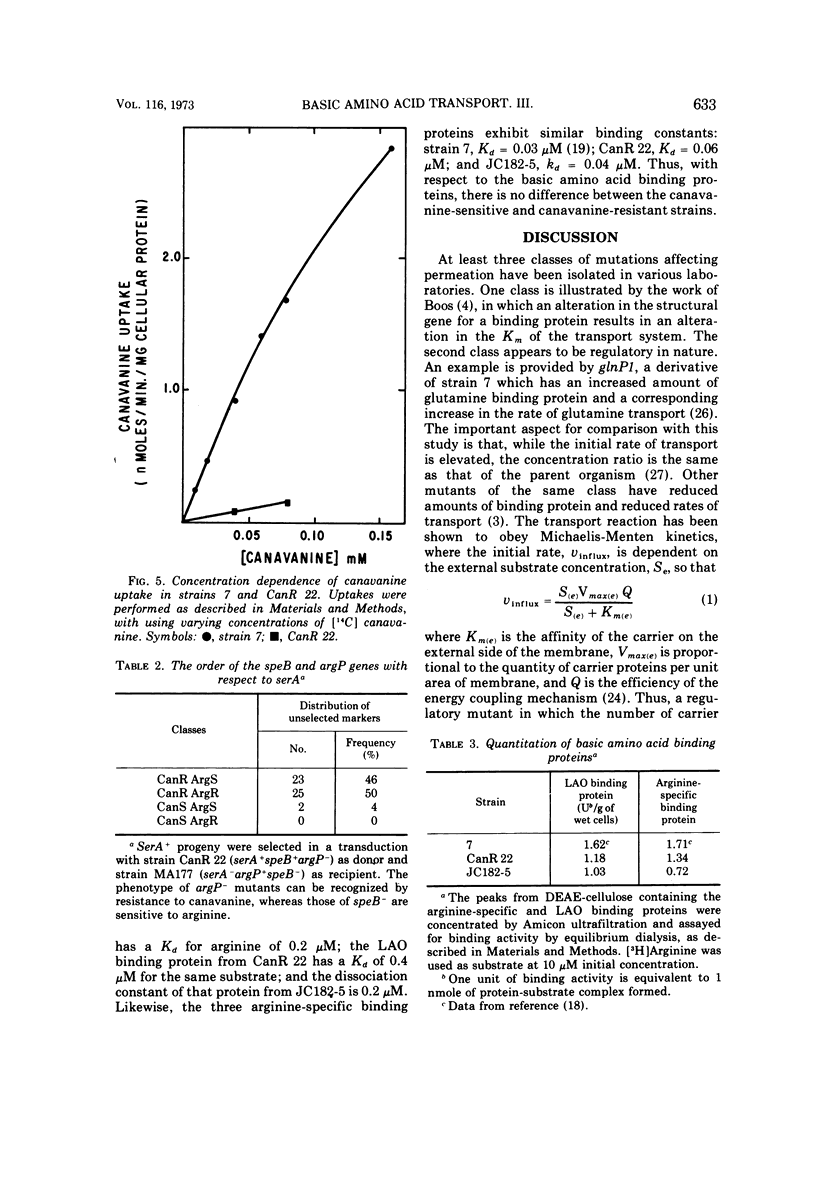
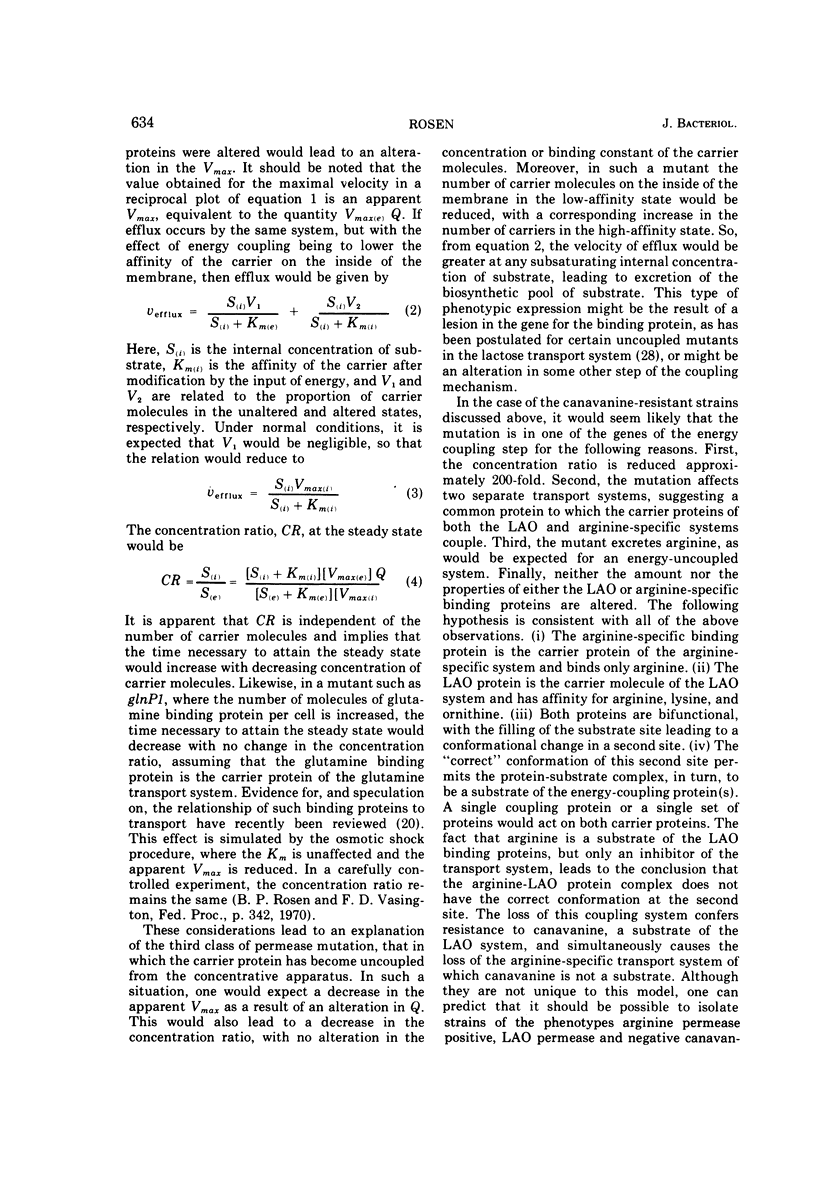
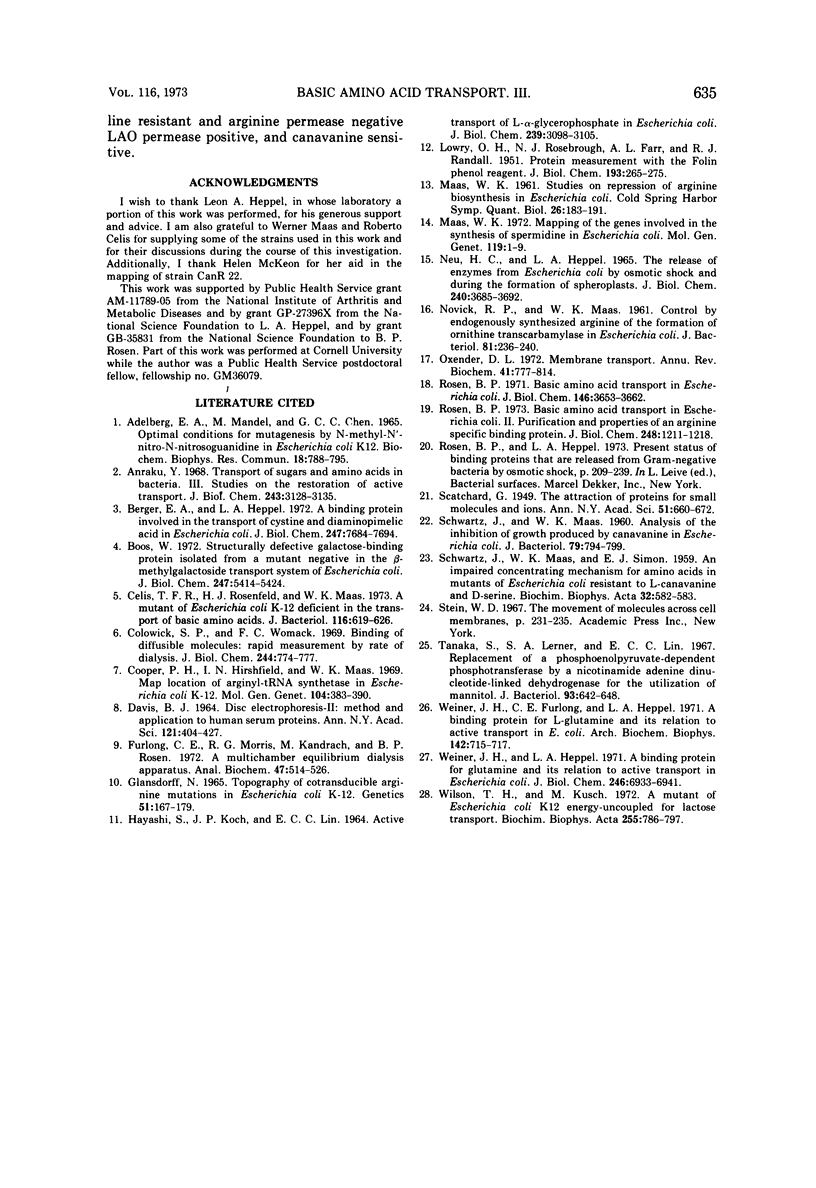
Selected References
These references are in PubMed. This may not be the complete list of references from this article.
- Anraku Y. Transport of sugars and amino acids in bacteria. 3. Studies on the restoration of active transport. J Biol Chem. 1968 Jun 10;243(11):3128–3135. [PubMed] [Google Scholar]
- Berger E. A., Heppel L. A. A binding protein involved in the transport of cystine and diaminopimelic acid in Escherichia coli. J Biol Chem. 1972 Dec 10;247(23):7684–7694. [PubMed] [Google Scholar]
- Boos W. Structurally defective galactose-binding protein isolated from a mutant negative in the -methylgalactoside transport system of Escherichia coli. J Biol Chem. 1972 Sep 10;247(17):5414–5424. [PubMed] [Google Scholar]
- Celis T. F., Rosenfeld H. J., Maas W. K. Mutant of Escherichia coli K-12 defective in the transport of basic amino acids. J Bacteriol. 1973 Nov;116(2):619–626. doi: 10.1128/jb.116.2.619-626.1973. [DOI] [PMC free article] [PubMed] [Google Scholar]
- Colowick S. P., Womack F. C. Binding of diffusible molecules by macromolecules: rapid measurement by rate of dialysis. J Biol Chem. 1969 Feb 25;244(4):774–777. [PubMed] [Google Scholar]
- Cooper P. H., Hirshfield I. N., Maas W. K. Map location of arginyl-tRNA synthetase mutations in Escherichia coli K-12. Mol Gen Genet. 1969 Aug 15;104(4):383–390. doi: 10.1007/BF00334238. [DOI] [PubMed] [Google Scholar]
- DAVIS B. J. DISC ELECTROPHORESIS. II. METHOD AND APPLICATION TO HUMAN SERUM PROTEINS. Ann N Y Acad Sci. 1964 Dec 28;121:404–427. doi: 10.1111/j.1749-6632.1964.tb14213.x. [DOI] [PubMed] [Google Scholar]
- Furlong C. E., Morris R. G., Kandrach M., Rosen B. P. A multichamber equilibrium dialysis apparatus. Anal Biochem. 1972 Jun;47(2):514–526. doi: 10.1016/0003-2697(72)90146-7. [DOI] [PubMed] [Google Scholar]
- GLANSDORFF N. TOPOGRAPHY OF COTRANSDUCIBLE ARGININE MUTATIONS IN ESCHERICHIA COLI K-12. Genetics. 1965 Feb;51:167–179. doi: 10.1093/genetics/51.2.167. [DOI] [PMC free article] [PubMed] [Google Scholar]
- HAYASHI S., KOCH J. P., LIN E. C. ACTIVE TRANSPORT OF L-ALPHA-GLYCEROPHOSPHATE IN ESCHERICHIA COLI. J Biol Chem. 1964 Sep;239:3098–3105. [PubMed] [Google Scholar]
- LOWRY O. H., ROSEBROUGH N. J., FARR A. L., RANDALL R. J. Protein measurement with the Folin phenol reagent. J Biol Chem. 1951 Nov;193(1):265–275. [PubMed] [Google Scholar]
- MAAS W. K. Studies on repression of arginine biosynthesis in Escherichia coli. Cold Spring Harb Symp Quant Biol. 1961;26:183–191. doi: 10.1101/sqb.1961.026.01.023. [DOI] [PubMed] [Google Scholar]
- Maas W. K. Mapping of genes involved in the synthesis of spermidine in Escherichia coli. Mol Gen Genet. 1972;119(1):1–9. doi: 10.1007/BF00270439. [DOI] [PubMed] [Google Scholar]
- NOVICK R. P., MAAS W. K. Control by endogenously synthesized arginine of the formation of ornithine transcarbamylase in Escherichia coli. J Bacteriol. 1961 Feb;81:236–240. doi: 10.1128/jb.81.2.236-240.1961. [DOI] [PMC free article] [PubMed] [Google Scholar]
- Neu H. C., Heppel L. A. The release of enzymes from Escherichia coli by osmotic shock and during the formation of spheroplasts. J Biol Chem. 1965 Sep;240(9):3685–3692. [PubMed] [Google Scholar]
- Oxender D. L. Membrane transport. Annu Rev Biochem. 1972;41(10):777–814. doi: 10.1146/annurev.bi.41.070172.004021. [DOI] [PubMed] [Google Scholar]
- Rosen B. P. Basic amino acid transport in Escherichia coli. II. Purification and properties of an arginine-specific binding protein. J Biol Chem. 1973 Feb 25;248(4):1211–1218. [PubMed] [Google Scholar]
- Rosen B. P. Basic amino acid transport in Escherichia coli. J Biol Chem. 1971 Jun 10;246(11):3653–3662. [PubMed] [Google Scholar]
- SCHWARTZ J. H., MAAS W. K. Analysis of the inhibition of growth produced by canavanine in Escherichia coli. J Bacteriol. 1960 Jun;79:794–799. doi: 10.1128/jb.79.6.794-799.1960. [DOI] [PMC free article] [PubMed] [Google Scholar]
- SCHWARTZ J. H., MAAS W. K., SIMON E. J. An impaired concentrating mechanism for amino acids in mutants of Escherichia coli resistant to L-canavanine and D-serine. Biochim Biophys Acta. 1959 Apr;32:582–583. doi: 10.1016/0006-3002(59)90650-x. [DOI] [PubMed] [Google Scholar]
- Tanaka S., Lerner S. A., Lin E. C. Replacement of a phosphoenolpyruvate-dependent phosphotransferase by a nicotinamide adenine dinucleotide-linked dehydrogenase for the utilization of mannitol. J Bacteriol. 1967 Feb;93(2):642–648. doi: 10.1128/jb.93.2.642-648.1967. [DOI] [PMC free article] [PubMed] [Google Scholar]
- Weiner J. H., Furlong C. E., Heppel L. A. A binding protein for L-glutamine and its relation to active transport in E. coli. Arch Biochem Biophys. 1971 Feb;142(2):715–717. doi: 10.1016/0003-9861(71)90538-8. [DOI] [PubMed] [Google Scholar]
- Wilson T. H., Kusch M. A mutant of Escherichia coli K 12 energy-uncoupled for lactose transport. Biochim Biophys Acta. 1972 Mar 17;255(3):786–797. doi: 10.1016/0005-2736(72)90391-4. [DOI] [PubMed] [Google Scholar]