Abstract
2-Keto-3-deoxy-gluconate (KDG), an intermediate of the hexuronate pathway in Escherichia coli K-12, is utilized as the sole carbon source only in strains derepressed for the specific KDG-uptake system. KDG is metabolized to pyruvate and glyceraldehyde-3-phosphate via the inducible enzymes KDG-kinase and 2-keto-3-deoxy-6-phosphate-gluconate (KDPG) aldolase. However, another inducible pathway, where the KDG is the branch point, has been demonstrated. Genetic studies of the KDG degradative pathway reported in this paper led to the location of KDG kinase-negative and pleiotropic constitutive mutations. The kdgK locus, presumably the structural gene of the kinase, occurs at min 69 and is co-transducible with xyl. The mutants, simultaneously constitutive for the uptake, kinase, and aldolase, define a kdgR locus at min 36 between the co-transducible markers kdgA and oldD. As to the nature of the control exerted by the kdgR product, we have shown the following. (i) Thermosensitive mutants of the kdgR locus are inducible at low temperature but derepressed at 42 C for the three operons—kdgT (transport system), kdgK, and kdgA (KDPG aldolase). (ii) The kdgR+ allele is dominant to the kdgR constitutive allele. (iii) A deletion in kdgA extending into the regulatory gene, kdgR, leads to a constitutive expression of the nondeleted operons—kdgT and kdgK. These properties demonstrate that the kdg regulon is negatively controlled by the kdgR product. It is presumed that differences in operator and in promotor structures could explain the strong decoordination, respectively, in the induction and catabolic repression, of these three enzymes activities.
Full text
PDF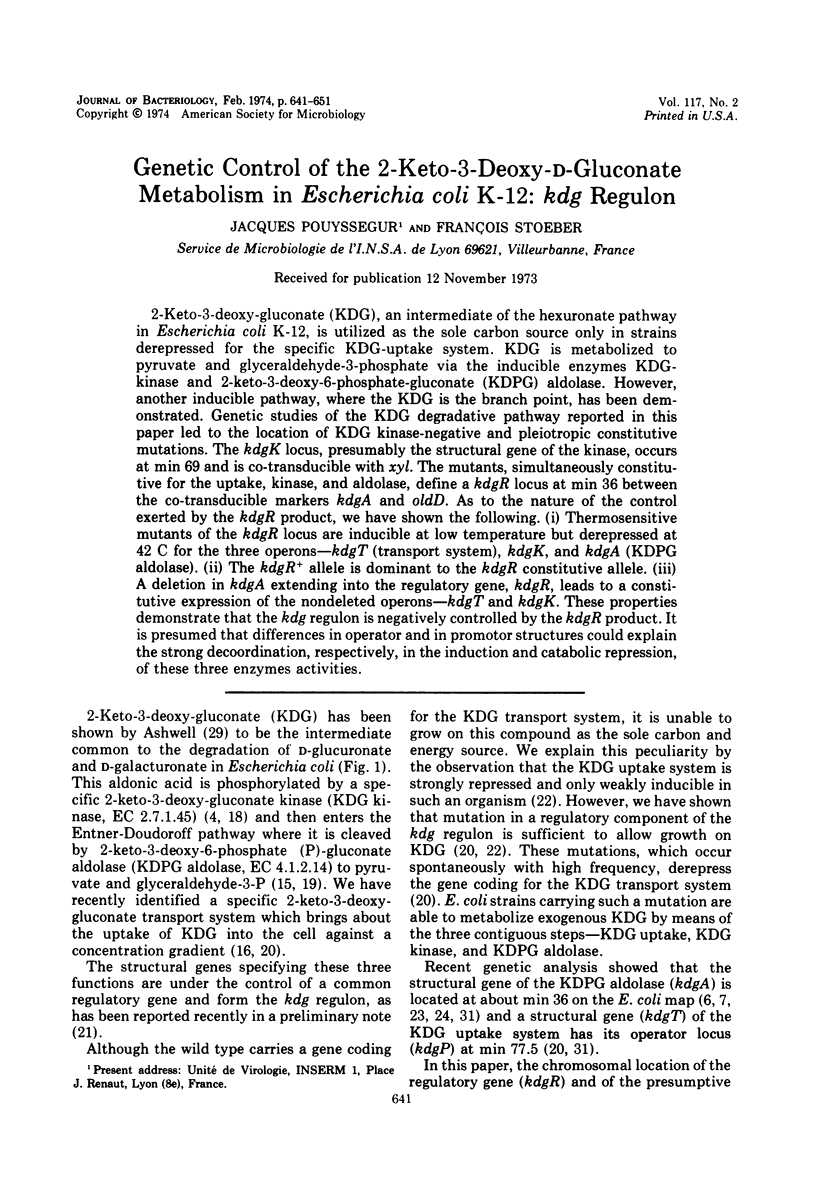
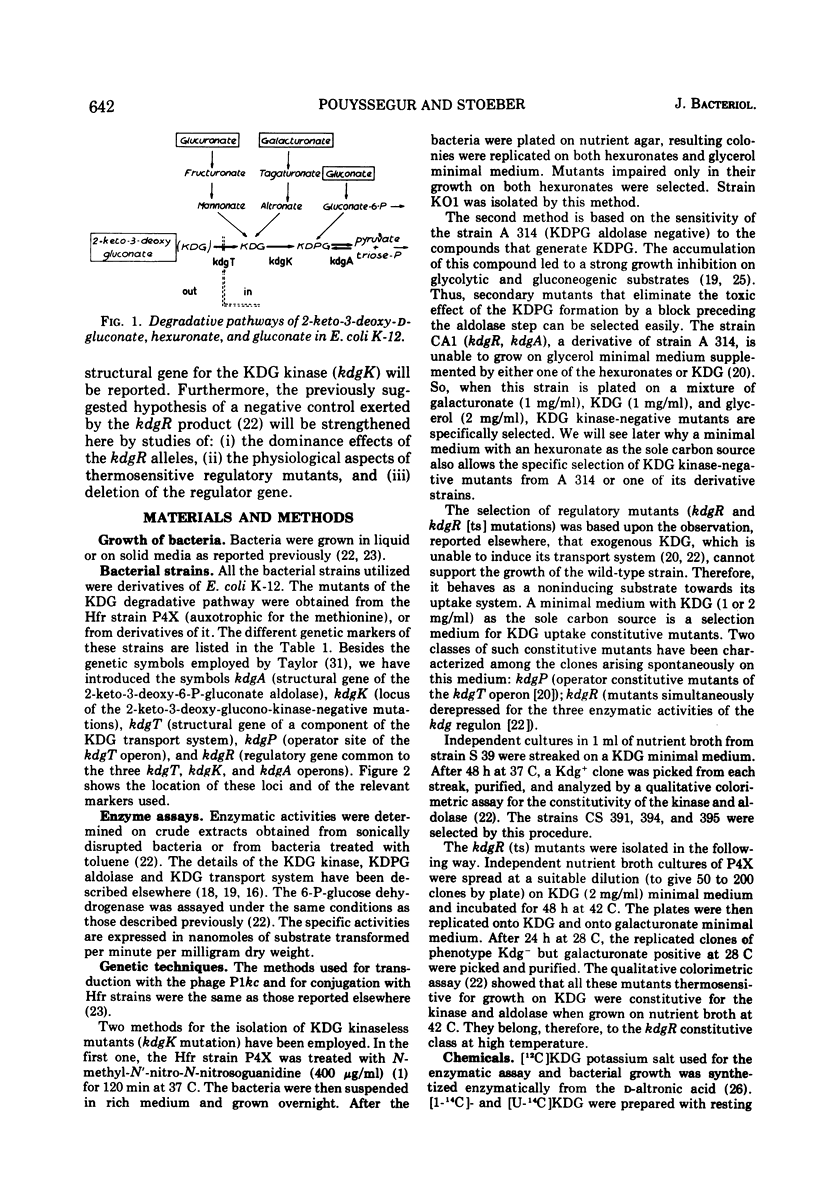
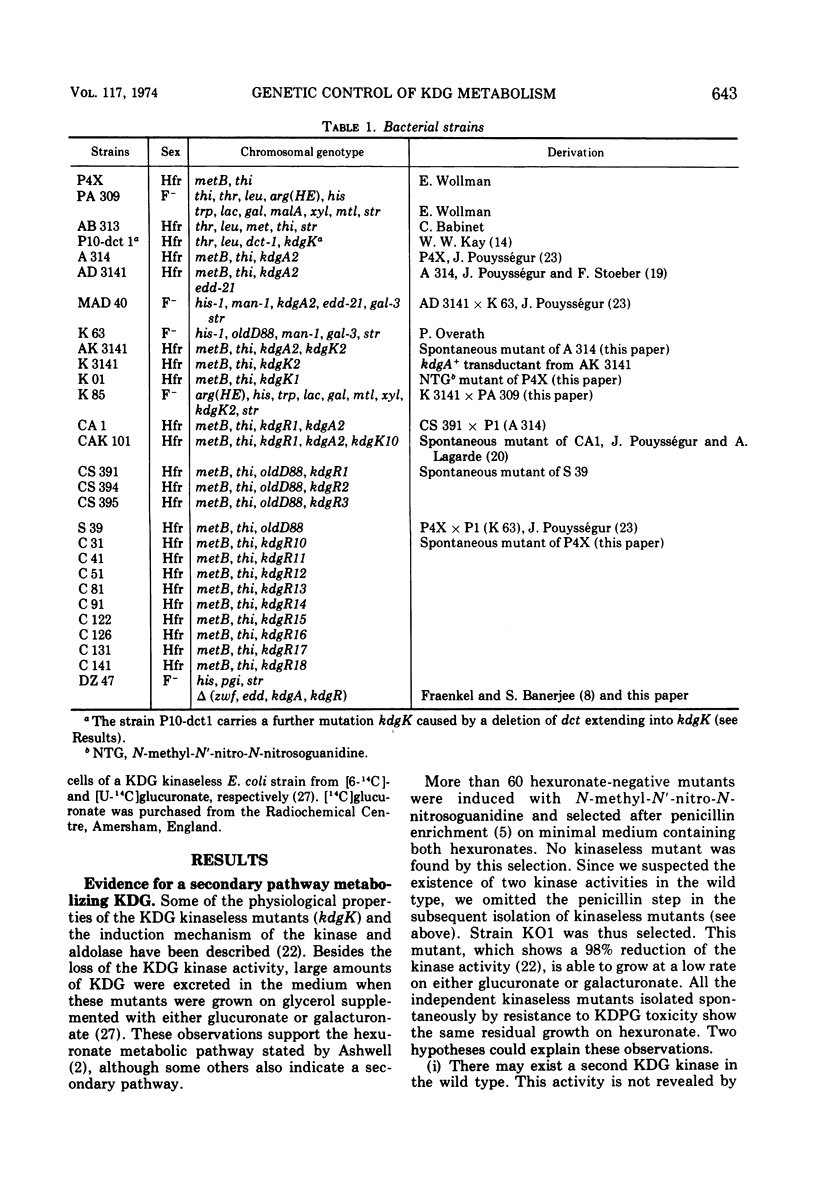
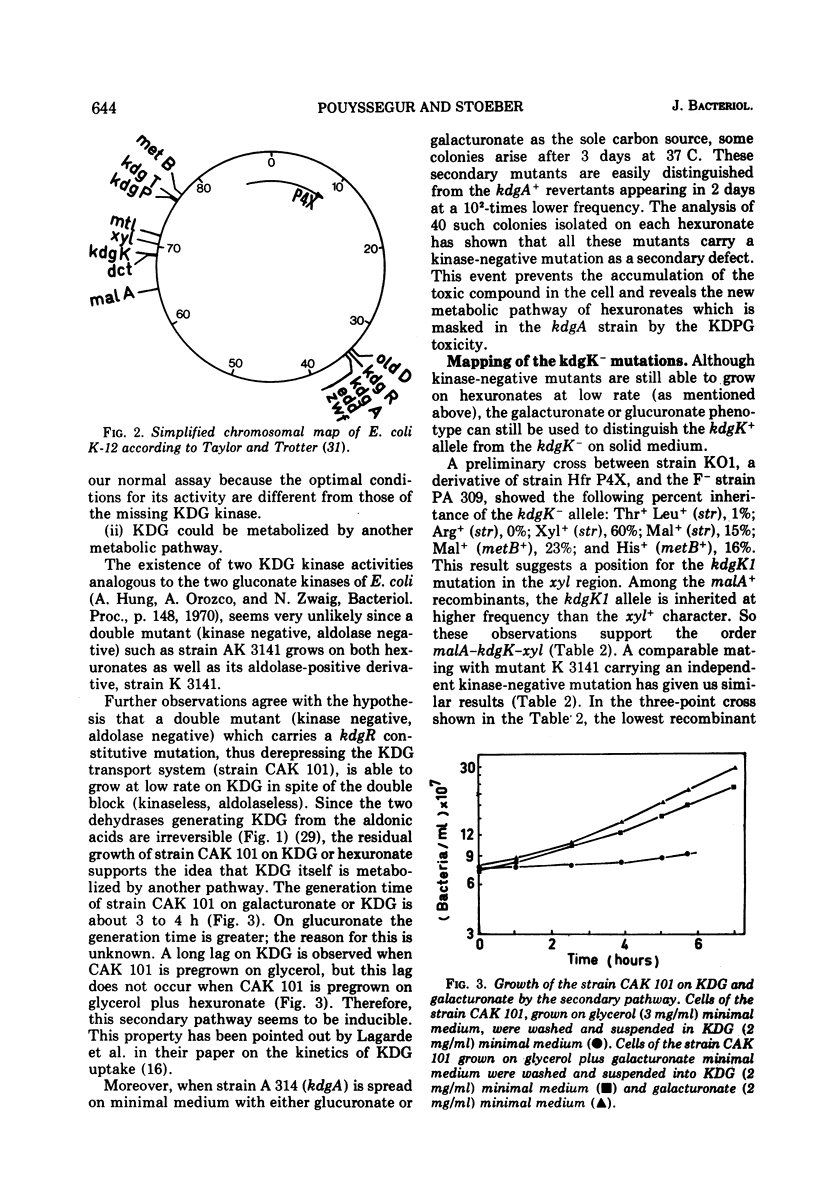
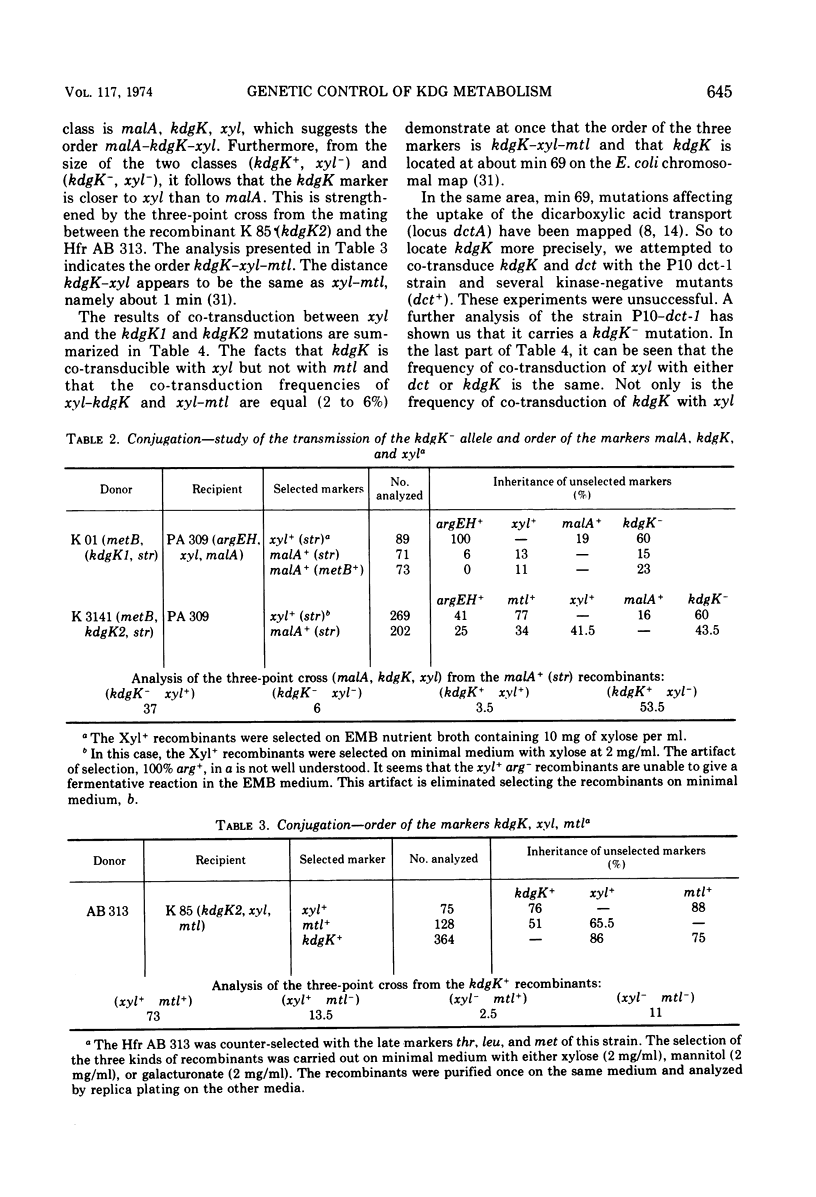
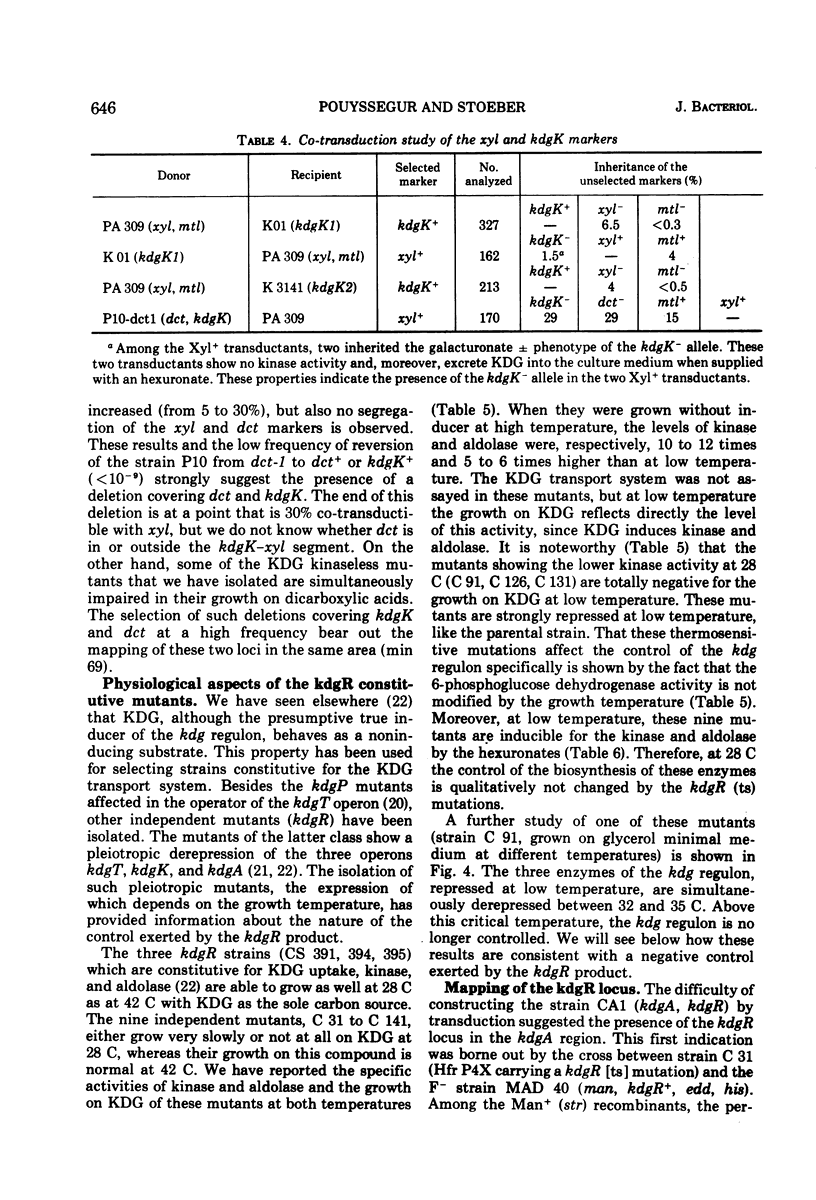
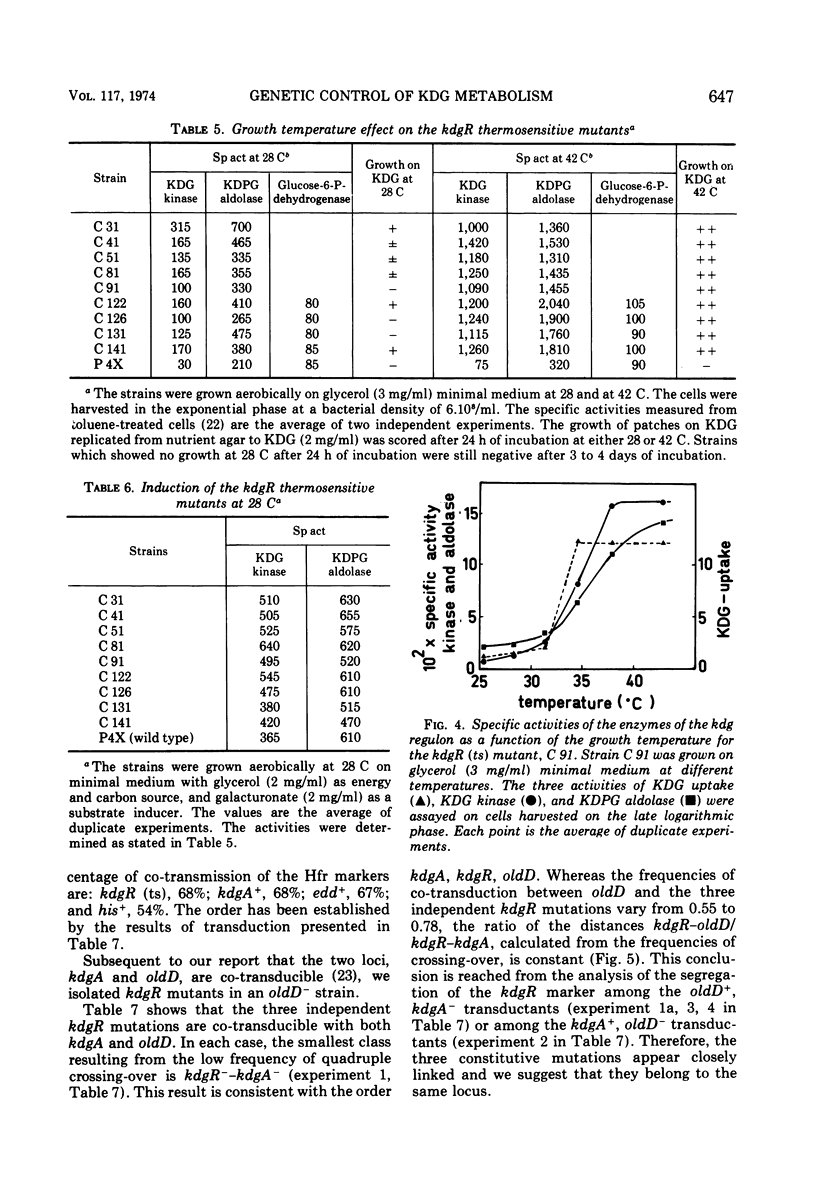
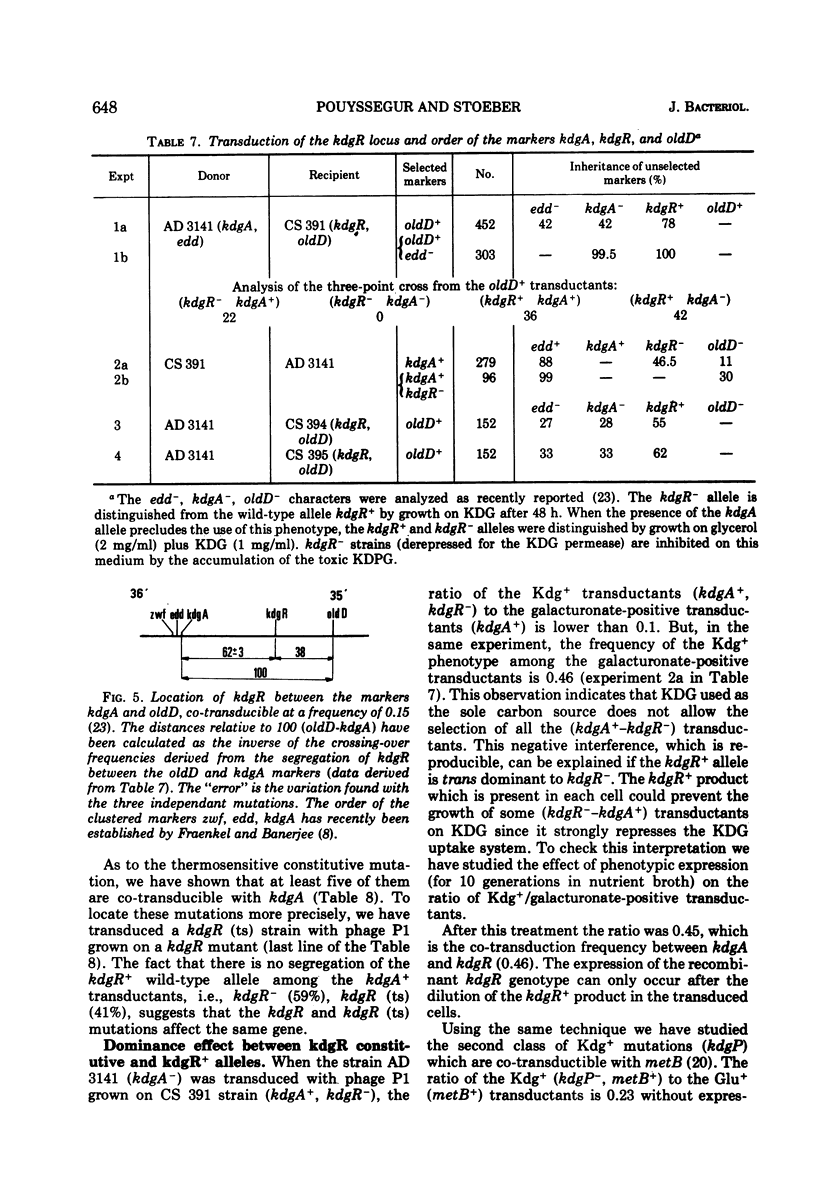
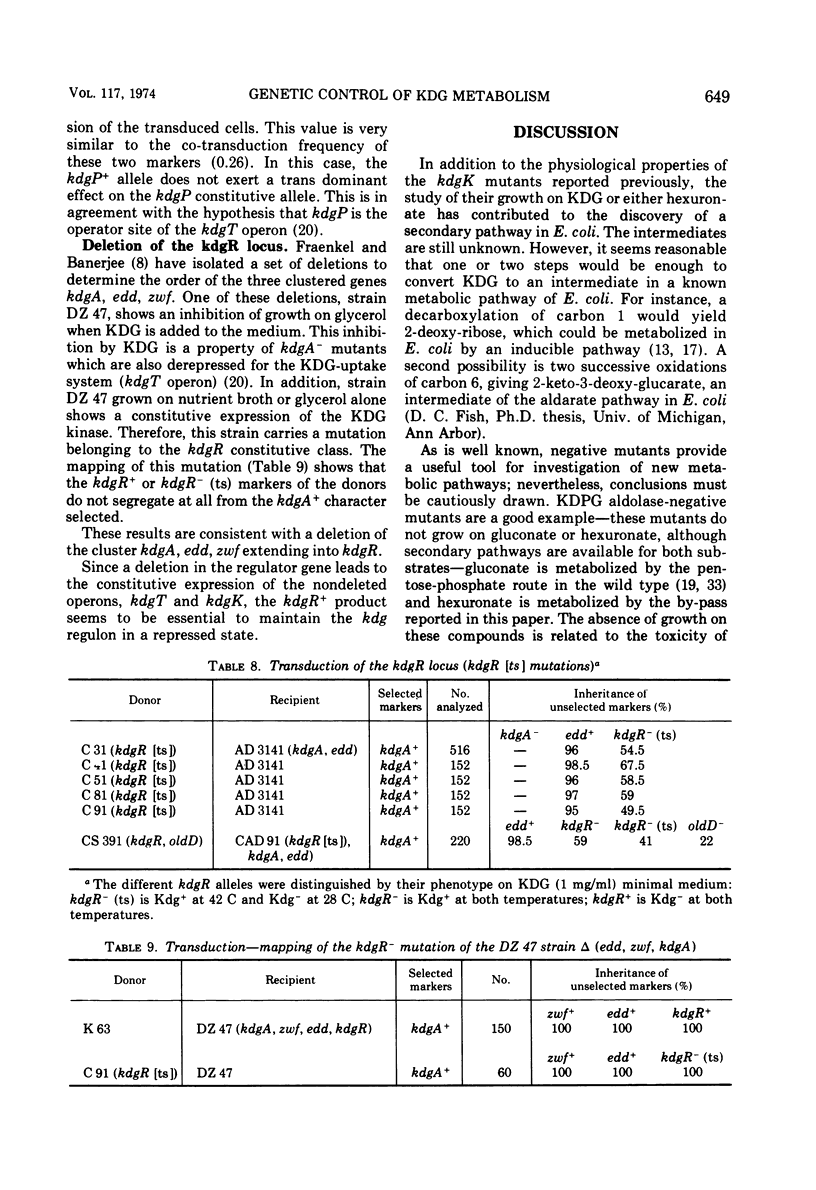
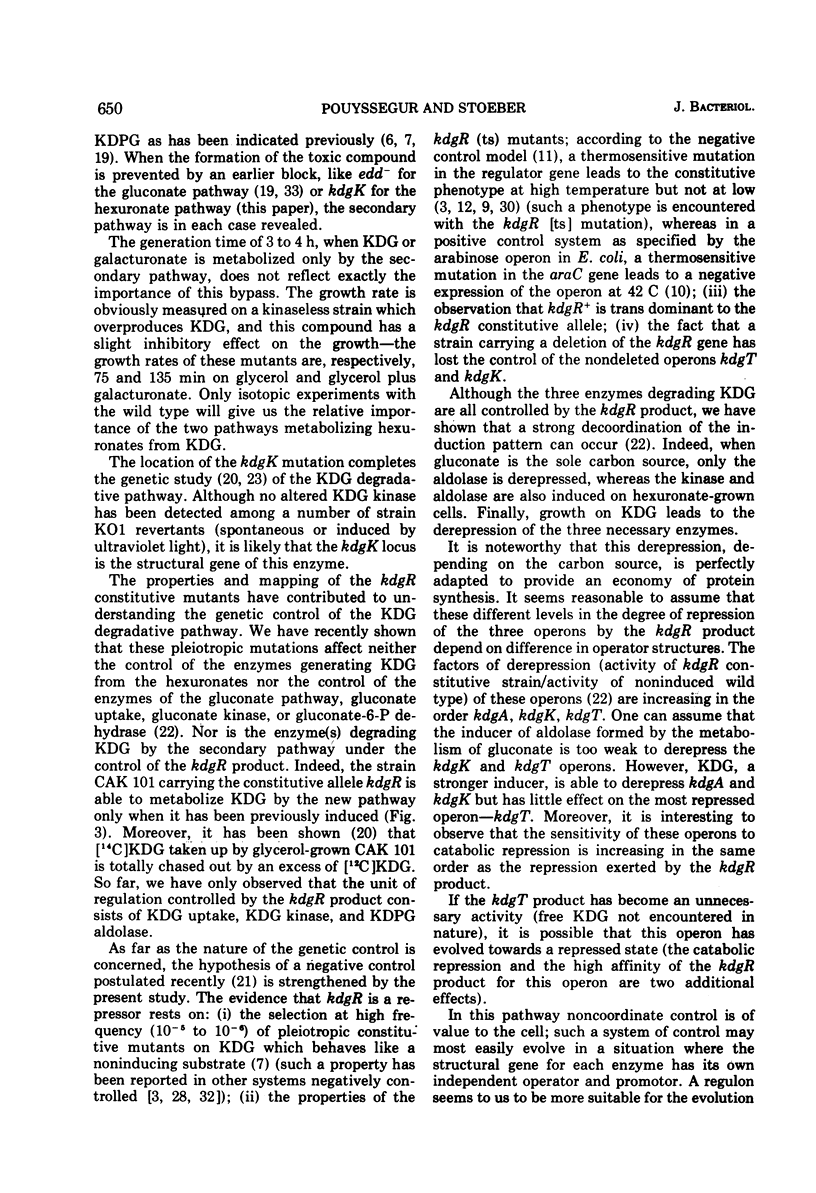
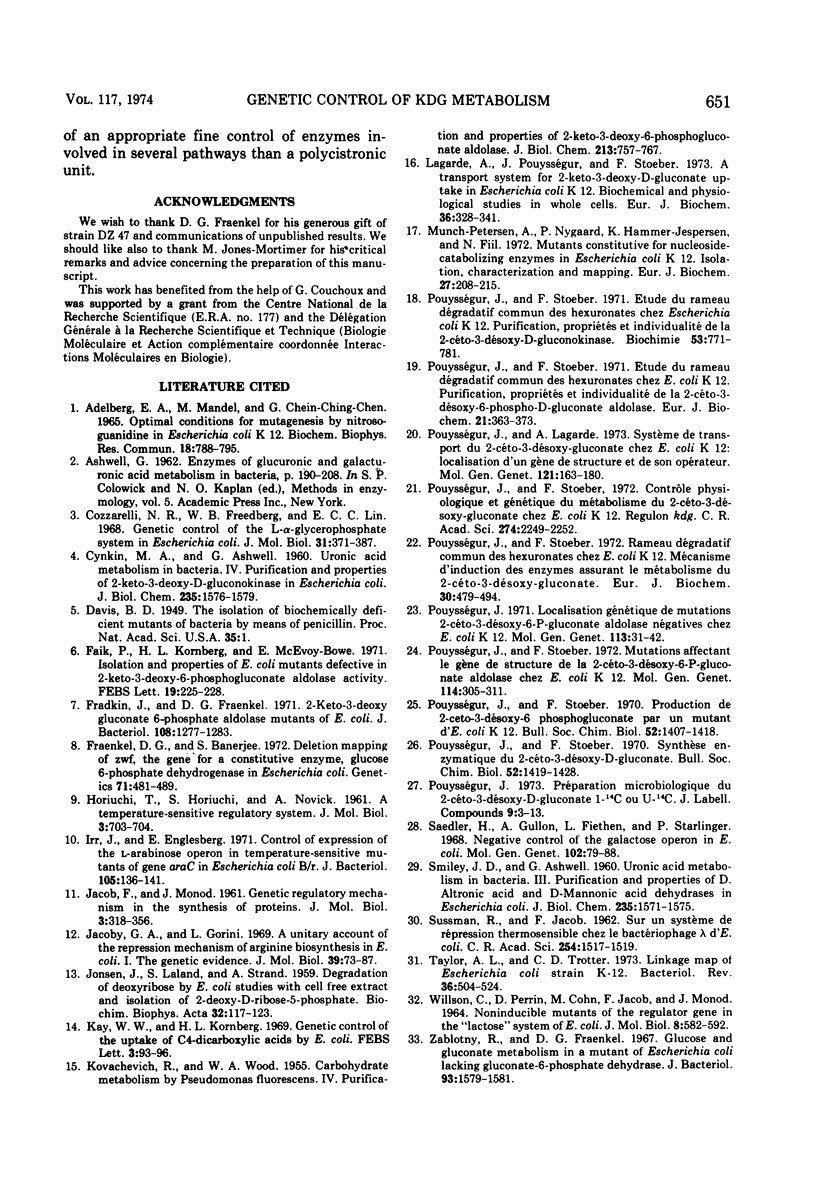
Selected References
These references are in PubMed. This may not be the complete list of references from this article.
- CYNKIN M. A., ASHWELL G. Uronic acid metabolism in bacteria. IV. Purification and properties of 2-keto-3-deoxy-D-gluconokinase in Escherichia coli. J Biol Chem. 1960 Jun;235:1576–1579. [PubMed] [Google Scholar]
- Cozzarelli N. R., Freedberg W. B., Lin E. C. Genetic control of L-alpha-glycerophosphate system in Escherichia coli. J Mol Biol. 1968 Feb 14;31(3):371–387. doi: 10.1016/0022-2836(68)90415-4. [DOI] [PubMed] [Google Scholar]
- Davis B. D. The Isolation of Biochemically Deficient Mutants of Bacteria by Means of Penicillin. Proc Natl Acad Sci U S A. 1949 Jan;35(1):1–10. doi: 10.1073/pnas.35.1.1. [DOI] [PMC free article] [PubMed] [Google Scholar]
- Faik P., Kornberg H. L., McEvoy-Bowe E. Isolation and properties of Escherichia coli mutants defective in 2-keto 3-deoxy 6-phosphogluconate aldolase activity. FEBS Lett. 1971 Dec 15;19(3):225–228. doi: 10.1016/0014-5793(71)80519-7. [DOI] [PubMed] [Google Scholar]
- Fradkin J. E., Fraenkel D. G. 2-keto-3-deoxygluconate 6-phosphate aldolase mutants of Escherichia coli. J Bacteriol. 1971 Dec;108(3):1277–1283. doi: 10.1128/jb.108.3.1277-1283.1971. [DOI] [PMC free article] [PubMed] [Google Scholar]
- Fraenkel D. G., Banerjee S. Deletion mapping of zwf, the gene for a constitutive enzyme, glucose 6-phosphate dehydrogenase in Escherichia coli. Genetics. 1972 Aug;71(4):481–489. doi: 10.1093/genetics/71.4.481. [DOI] [PMC free article] [PubMed] [Google Scholar]
- HORIUCHI T., HORIUCHI S., NOVICK A. A temperature-sensitive regulatory system. J Mol Biol. 1961 Oct;3:703–704. doi: 10.1016/s0022-2836(61)80035-1. [DOI] [PubMed] [Google Scholar]
- Irr J., Englesberg E. Control of expression of the L-arabinose operon in temperature-sensitive mutants of gene araC in Escherichia coli B-r. J Bacteriol. 1971 Jan;105(1):136–141. doi: 10.1128/jb.105.1.136-141.1971. [DOI] [PMC free article] [PubMed] [Google Scholar]
- JACOB F., MONOD J. Genetic regulatory mechanisms in the synthesis of proteins. J Mol Biol. 1961 Jun;3:318–356. doi: 10.1016/s0022-2836(61)80072-7. [DOI] [PubMed] [Google Scholar]
- JONSEN J., LALAND S., STRAND A. Degradation of deoxyribose by E. coli; studies with cell-free extract and isolation of 2-deoxy-D-ribose 5-phosphate. Biochim Biophys Acta. 1959 Mar;32(1):117–123. doi: 10.1016/0006-3002(59)90559-1. [DOI] [PubMed] [Google Scholar]
- Jacoby G. A., Gorini L. A unitary account of the repression mechanism of arginine biosynthesis in Escherichia coli. I. The genetic evidence. J Mol Biol. 1969 Jan 14;39(1):73–87. doi: 10.1016/0022-2836(69)90334-9. [DOI] [PubMed] [Google Scholar]
- KOVACHEVICH R., WOOD W. A. Carbohydrate metabolism by Pseudomonas fluorescens. IV. Purification and properties of 2-keto-3-deoxy-6-phosphogluconate aldolase. J Biol Chem. 1955 Apr;213(2):757–767. [PubMed] [Google Scholar]
- Kay W. W., Kornberg H. L. Genetic control of the uptake of C(4)-dicarboxylic acids by Escherichia coli. FEBS Lett. 1969 Apr;3(2):93–96. doi: 10.1016/0014-5793(69)80105-5. [DOI] [PubMed] [Google Scholar]
- Lagarde A. E., Pouysségur J. M., Stoeber F. R. A transport system for 2-keto-3-deoxy-D-gluconate uptake in Escherichia coli K12. Biochemical and physiological studies in whole cells. Eur J Biochem. 1973 Jul 16;36(2):328–341. doi: 10.1111/j.1432-1033.1973.tb02917.x. [DOI] [PubMed] [Google Scholar]
- Munch-Petersen A., Nygaard P., Hammer-Jespersen K., Fiil N. Mutants constitutive for nucleoside-catabolizing enzymes in Escherichia coli K12. Isolation, charactrization and mapping. Eur J Biochem. 1972 May 23;27(2):208–215. doi: 10.1111/j.1432-1033.1972.tb01828.x. [DOI] [PubMed] [Google Scholar]
- Pouyssegur J. M. Localisation génétique de mutations 2-céto-3-désoxy-6-P-gluconate aldolase négatives chez E. coli K 12. Mol Gen Genet. 1971;113(1):31–42. [PubMed] [Google Scholar]
- Pouyssegur J., Stoeber F. Etude du rameu dégradatif commun des hexuronates chez Escherichia coli K 12. Purification, propriétés et individualité de la 2-céto-3-désoxy-D-gluconokinase. Biochimie. 1971;53(6):771–781. [PubMed] [Google Scholar]
- Pouyssegur J., Stoeber F. Production de 2-céto-3-désoxy-6-phospho-gluconate par un mutant d'Escherichia coli K 12. Bull Soc Chim Biol (Paris) 1970;52(12):1407–1418. [PubMed] [Google Scholar]
- Pouyssegur J., Stoeber F. Synthèse enzymatique du 2-céto-3-désoxy-D-gluconate. Bull Soc Chim Biol (Paris) 1970;52(12):1419–1428. [PubMed] [Google Scholar]
- Pouysségur J. M., Stoeber F. R. Etude du rameau dégradatif commun des hexuronates chez Escherichia coli K 12. Purification, propriétés et individualité de la 2-céto-3-désoxy-6-phospho-D-gluconate aldolase. Eur J Biochem. 1971 Aug 16;21(3):363–373. doi: 10.1111/j.1432-1033.1971.tb01478.x. [DOI] [PubMed] [Google Scholar]
- Pouysségur J. M., Stoeber F. R. Rameau dégradatif commun des hexuronates chez Escherichia coli K12. Mécanisme d'induction des enzymes assurant le métabolisme du 2-céto-3-désoxy-gluconate. Eur J Biochem. 1972 Nov 7;30(3):479–494. doi: 10.1111/j.1432-1033.1972.tb02120.x. [DOI] [PubMed] [Google Scholar]
- Pouysségur J., Lagarde A. Système de transport du 2-céto-3-désoxy-gluconate chez E. coli K 12: localisation d'un gène de structure et de son opérateur. Mol Gen Genet. 1973 Mar 1;121(2):163–180. doi: 10.1007/BF00277530. [DOI] [PubMed] [Google Scholar]
- Pouysségur J., Stoeber F. Contrôle physiologique et génétique du métabolisme du 2-céto-3-désoxy-gluconate chez E. coli K 12. Régulon kdg. C R Acad Sci Hebd Seances Acad Sci D. 1972 Apr 10;274(15):2249–2252. [PubMed] [Google Scholar]
- Pouysségur J., Stoeber F. Mutations affectant le gène de structure de la 2-céto-3-désoxy-6-P-gluconate aldolase chez E. coli K 12. Mol Gen Genet. 1972;114(4):305–311. doi: 10.1007/BF00267499. [DOI] [PubMed] [Google Scholar]
- SMILEY J. D., ASHWELL G. Uronic acid metabolism in bacteria. III. Purification and properties of D-altronic acid and D-mannonic acid dehydrases in Escherichia coli. J Biol Chem. 1960 Jun;235:1571–1575. [PubMed] [Google Scholar]
- SUSSMAN R., JACOB F. [On a thermosensitive repression system in the Escherichia coli lambda bacteriophage]. C R Hebd Seances Acad Sci. 1962 Feb 19;254:1517–1519. [PubMed] [Google Scholar]
- Saedler H., Gullon A., Fiethen L., Starlinger P. Negative control of the galactose operon in E. coli. Mol Gen Genet. 1968;102(1):79–88. doi: 10.1007/BF00341872. [DOI] [PubMed] [Google Scholar]
- Taylor A. L., Trotter C. D. Linkage map of Escherichia coli strain K-12. Bacteriol Rev. 1972 Dec;36(4):504–524. doi: 10.1128/br.36.4.504-524.1972. [DOI] [PMC free article] [PubMed] [Google Scholar]
- WILLSON C., PERRIN D., COHN M., JACOB F., MONOD J. NON-INDUCIBLE MUTANTS OF THE REGULATOR GENE IN THE "LACTOSE" SYSTEM OF ESCHERICHIA COLI. J Mol Biol. 1964 Apr;8:582–592. doi: 10.1016/s0022-2836(64)80013-9. [DOI] [PubMed] [Google Scholar]
- Zablotny R., Fraenkel D. G. Glucose and gluconate metabolism in a mutant of Escherichia coli lacking gluconate-6-phosphate dehydrase. J Bacteriol. 1967 May;93(5):1579–1581. doi: 10.1128/jb.93.5.1579-1581.1967. [DOI] [PMC free article] [PubMed] [Google Scholar]