Abstract
When treated with chloramphenicol, Escherichia coli 15T minus produces two new species (IV and V) of transfer ribonucleic acid specific for phenylalanine in addition to the major normal species (II) and two minor normal species (I and III), which are seen as distinct components upon fractionation by chromatography on columns of benzoylated diethylaminoethyl-cellulose. Species IV is produced when cells are grown in iron-deficient medium and is, therefore, probably deficient in the 2-methylthio modification of N-6-(delta-2-isopentenyl) adenosine. A new minor species (Va) also appears under those conditions. All of the new components elute earlier than the major normal species. Addition of chloramphenicol to iron-deficient cells leads to the production of species V, and that production is blocked by rifampin, as is the production of species IV. Thus, species IV and V appear to be transcriptional products. Although E. coli 15T minus appears to be rel plus, starvation for methionine or cysteine leads to the accumulation of species IV (without addition of chloramphenicol); rifampin blocks the accumulation. Species V is still produced on addition of chloramphenicol to starved cultures. Starvation for arginine or tryptophan does not alter the chromatographic profile from the normal case. Treatment with permanganate indicates that species II and IV contain isopentenyladenosine but that species V does not. Species V appears to be deficient in both isopentenyl and methylthio modifications of adenosine and perhaps at least one other modification, because removing the isopentenyl moiety from adenosine does not convert species IV into species V, but converts it into species Va. A precursor relationship among species V, VI, and II is suggested by following the chromatographic profile of phenylalanine transfer ribonucleic acid during recovery of E. coli from treatment with chloramphenicol; the various species increase and decrease in a sequential manner.
Full text
PDF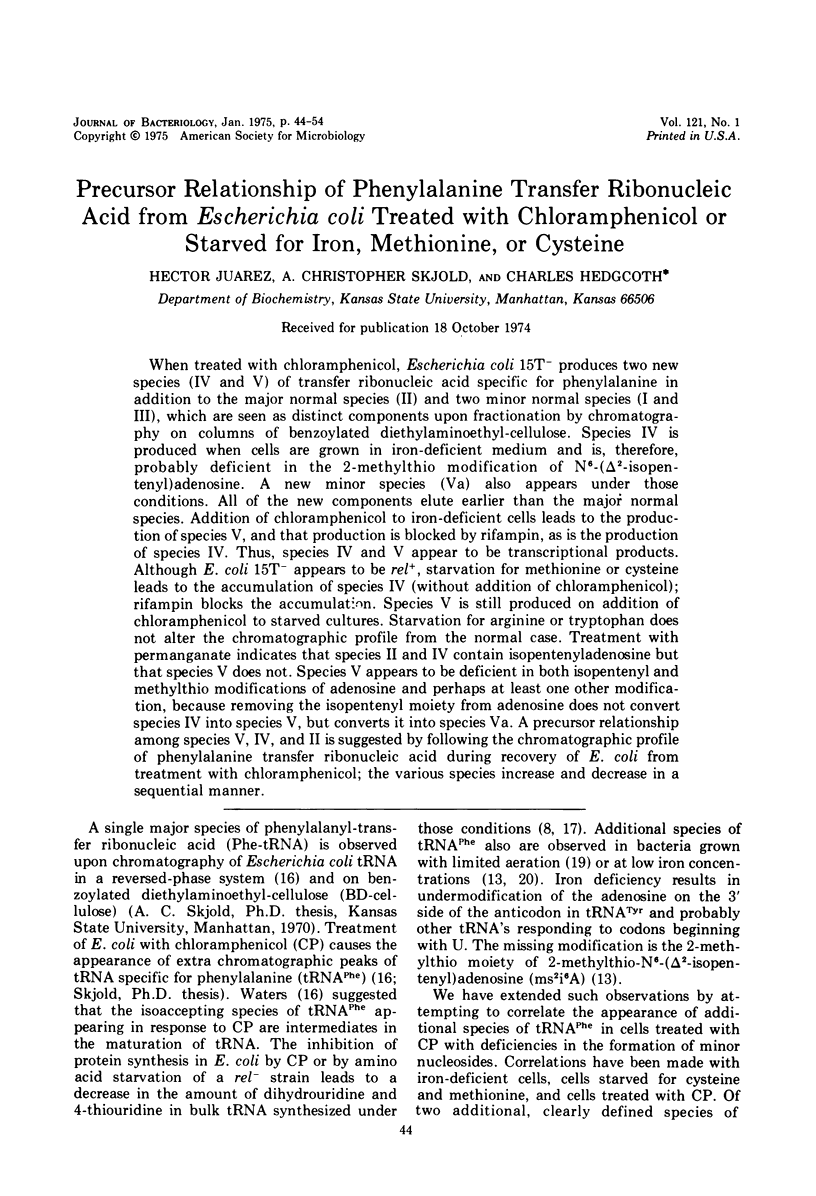
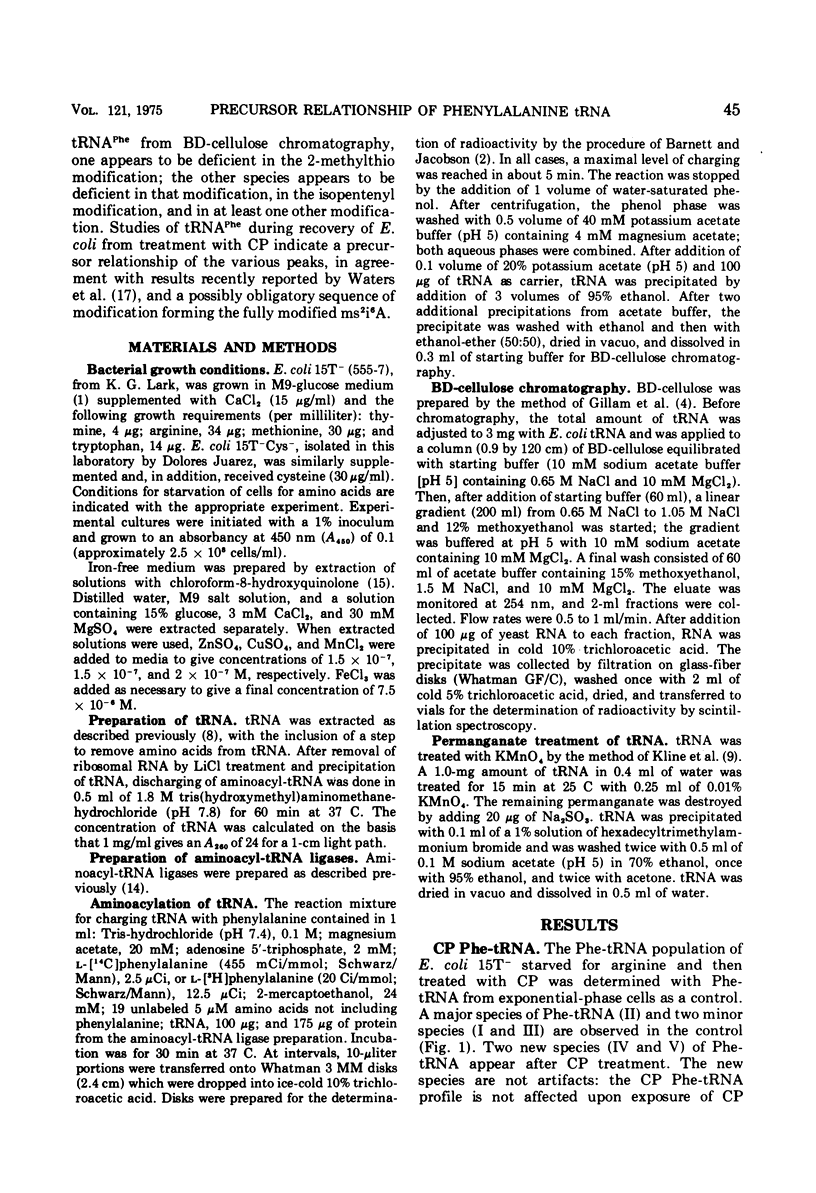
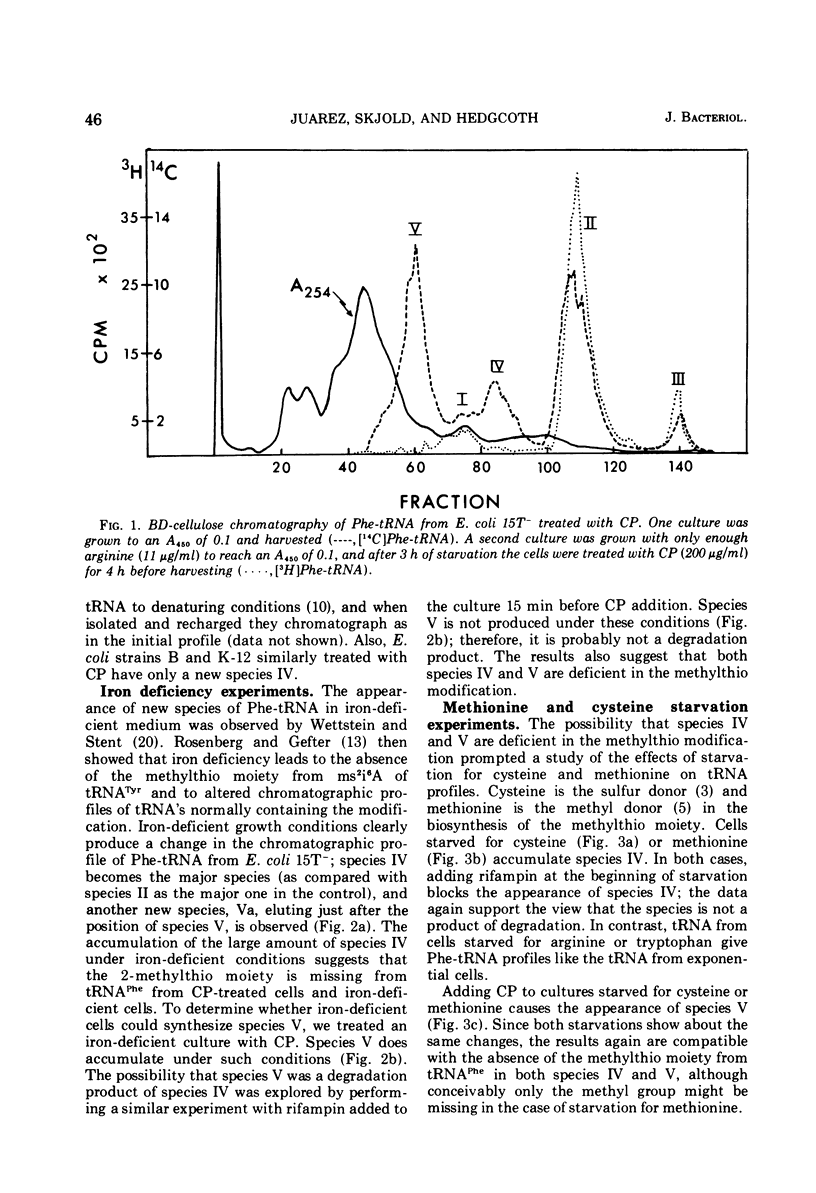
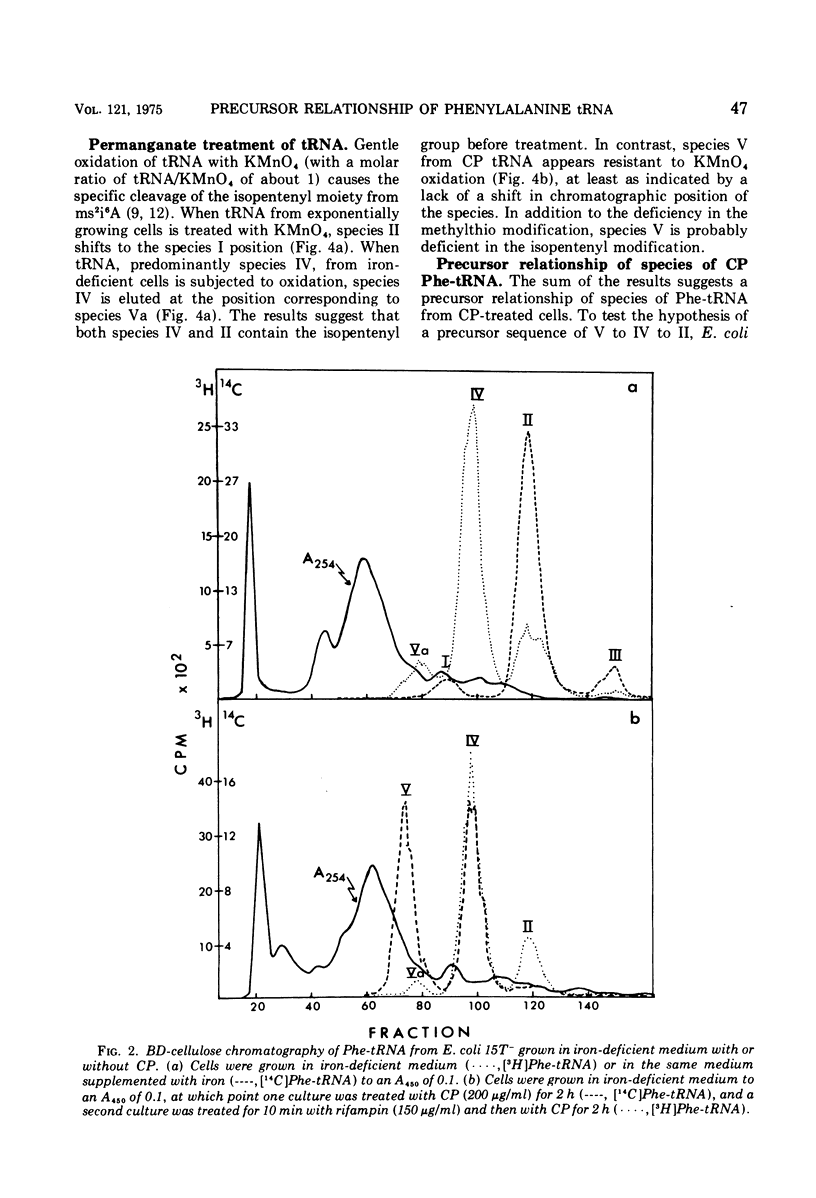
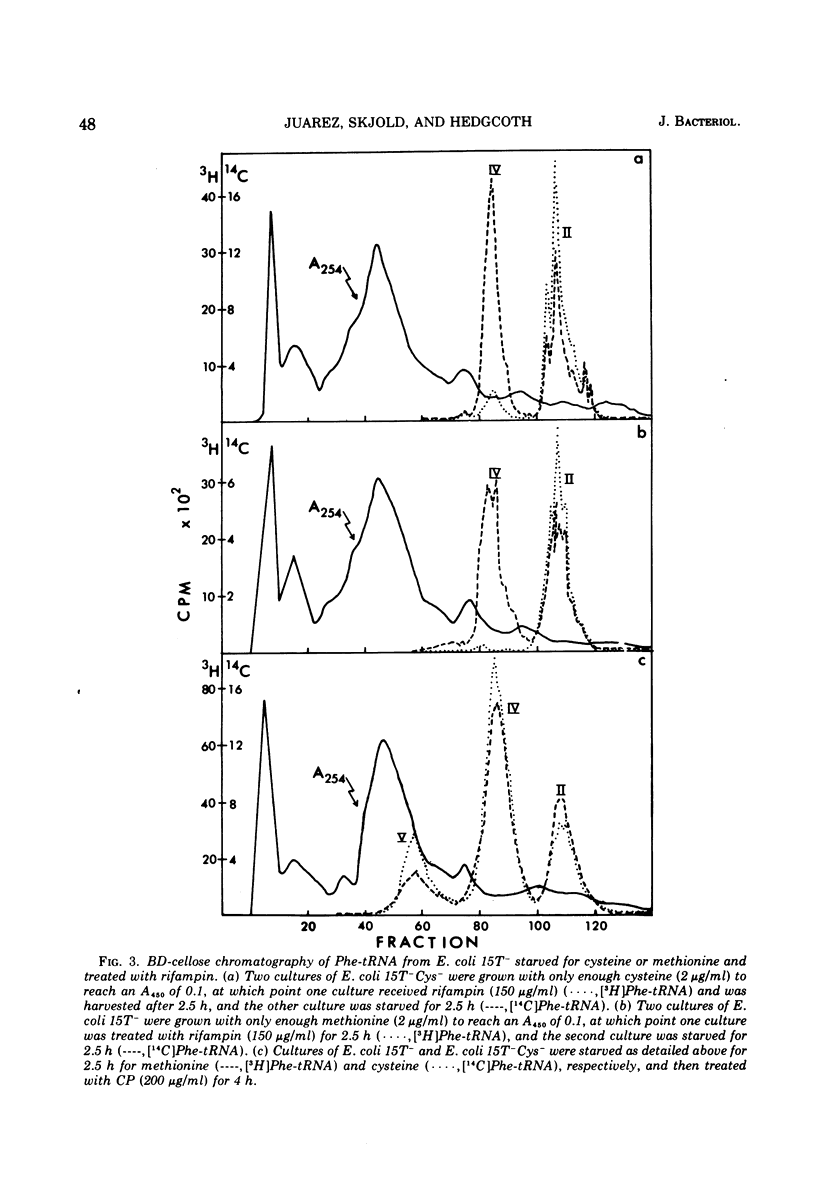
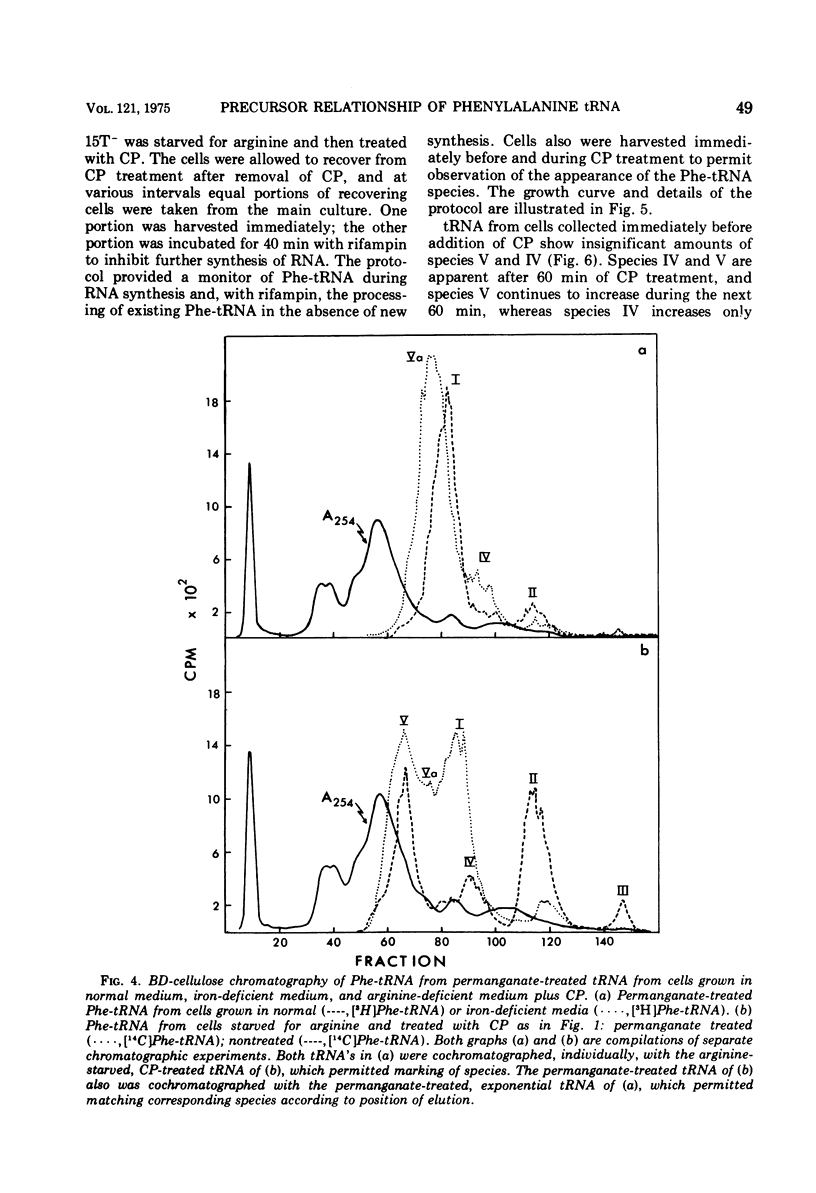
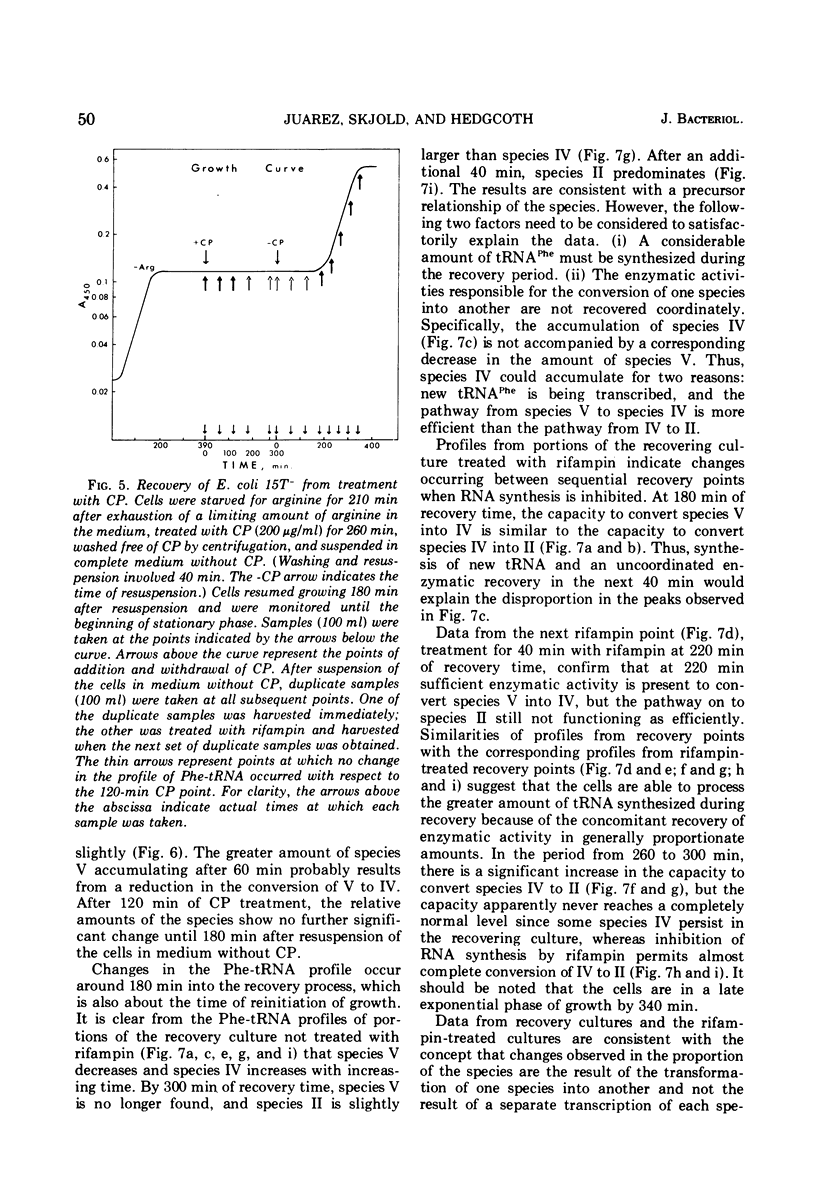
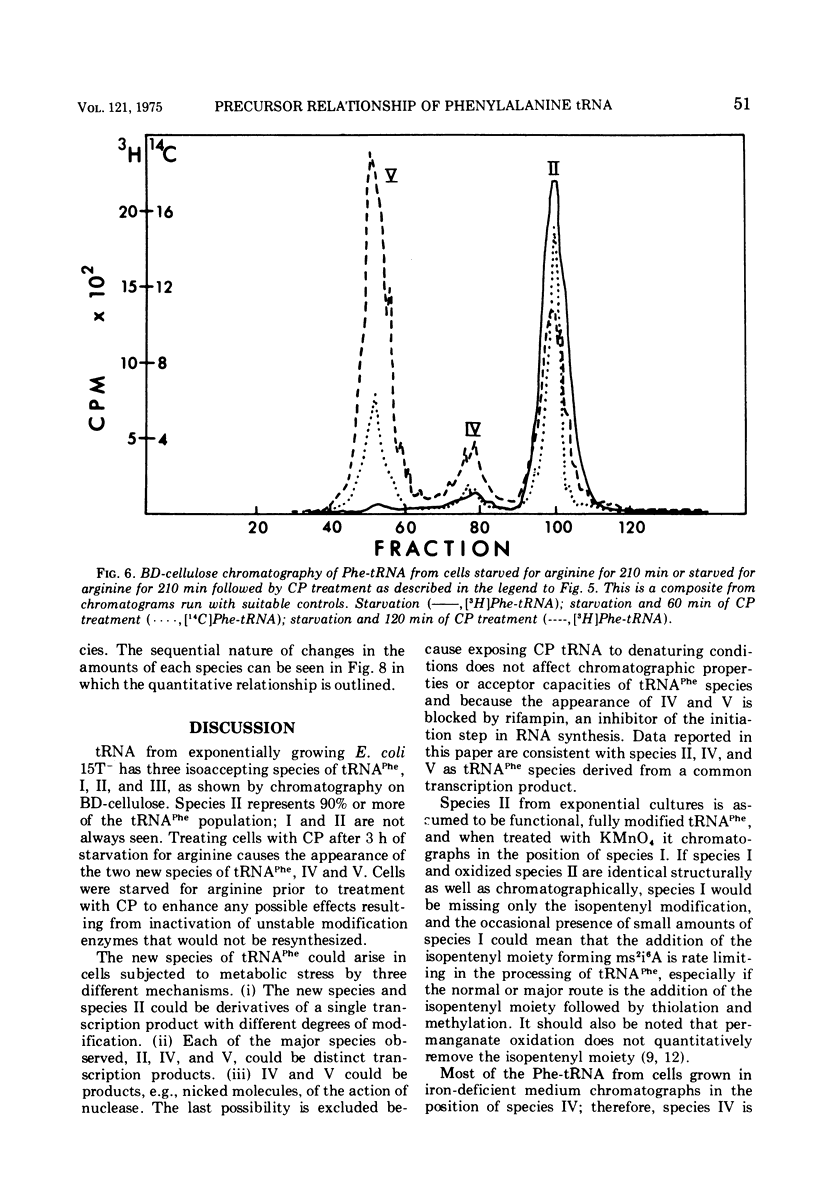
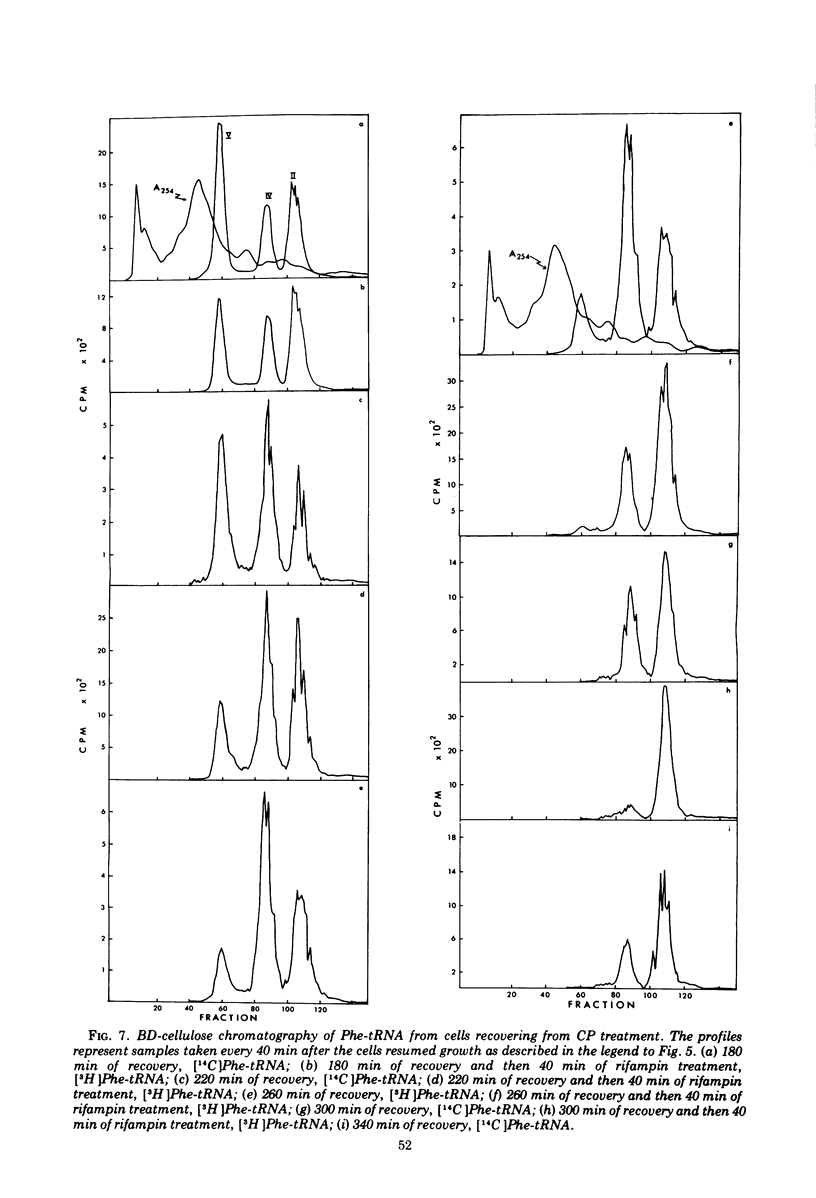
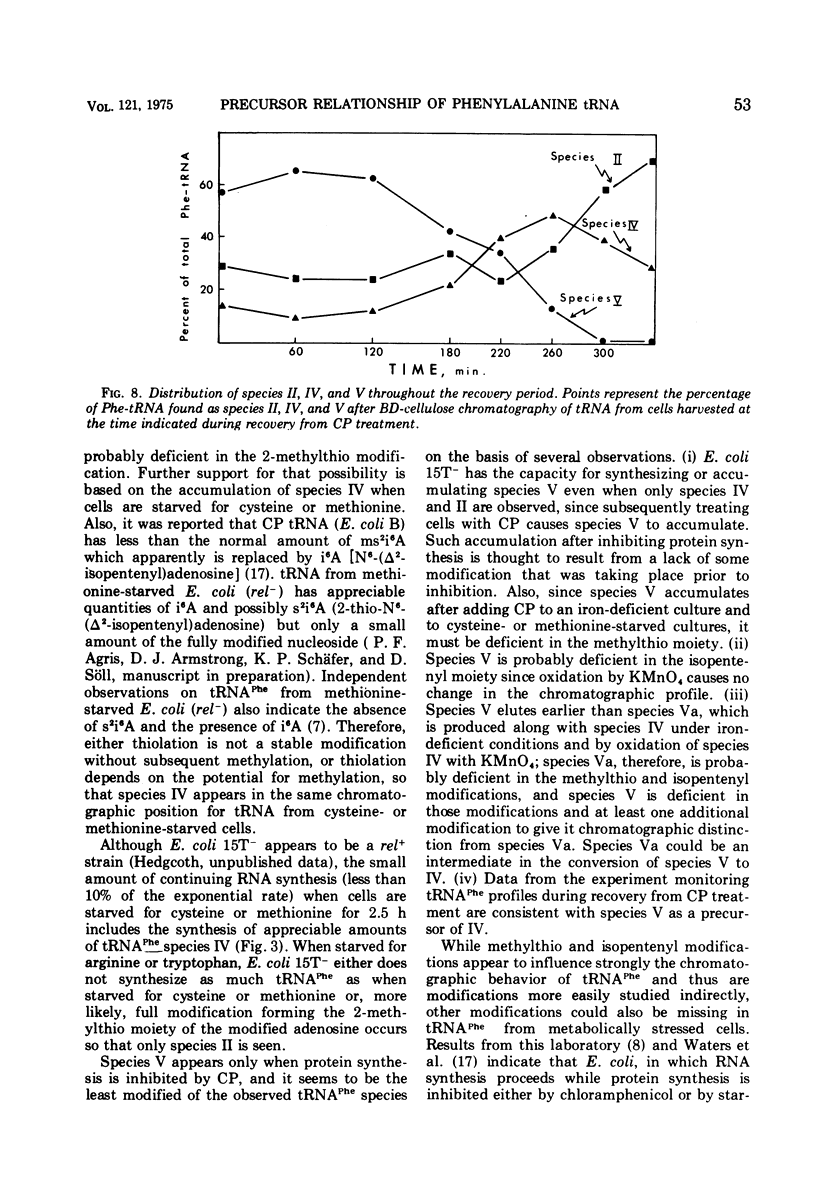
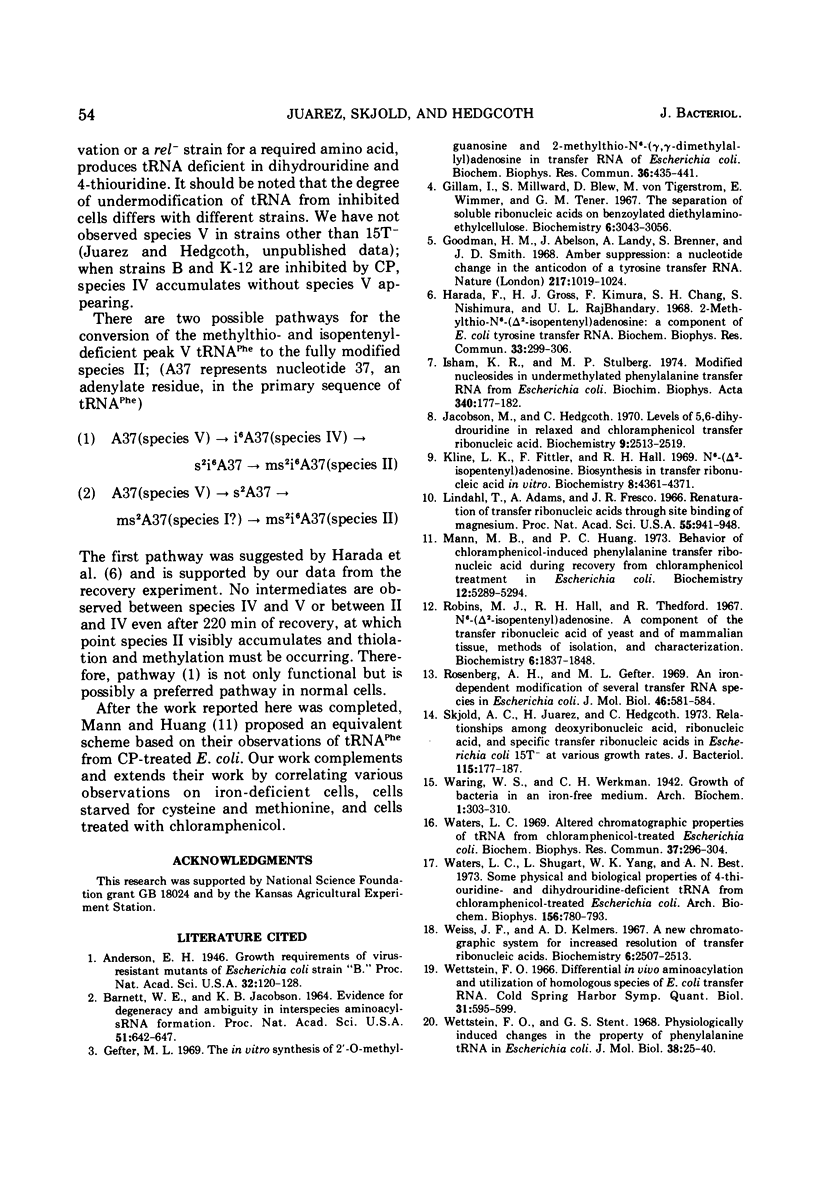
Selected References
These references are in PubMed. This may not be the complete list of references from this article.
- Anderson E. H. Growth Requirements of Virus-Resistant Mutants of Escherichia Coli Strain "B". Proc Natl Acad Sci U S A. 1946 May;32(5):120–128. doi: 10.1073/pnas.32.5.120. [DOI] [PMC free article] [PubMed] [Google Scholar]
- BARNETT W. E., JACOBSON K. B. EVIDENCE FOR DEGENERACY AND AMBIGUITY IN INTERSPECIES AMINOACYL-SRNA FORMATION. Proc Natl Acad Sci U S A. 1964 Apr;51:642–647. doi: 10.1073/pnas.51.4.642. [DOI] [PMC free article] [PubMed] [Google Scholar]
- Gefter M. L. The in vitro synthesis of 2'-omethylguanosine and 2-methylthio 6N (gamma,gamma, dimethylallyl) adenosine in transfer RNA of Escherichia coli. Biochem Biophys Res Commun. 1969 Aug 7;36(3):435–441. doi: 10.1016/0006-291x(69)90583-x. [DOI] [PubMed] [Google Scholar]
- Gillam I., Millward S., Blew D., von Tigerstrom M., Wimmer E., Tener G. M. The separation of soluble ribonucleic acids on benzoylated diethylaminoethylcellulose. Biochemistry. 1967 Oct;6(10):3043–3056. doi: 10.1021/bi00862a011. [DOI] [PubMed] [Google Scholar]
- Goodman H. M., Abelson J., Landy A., Brenner S., Smith J. D. Amber suppression: a nucleotide change in the anticodon of a tyrosine transfer RNA. Nature. 1968 Mar 16;217(5133):1019–1024. doi: 10.1038/2171019a0. [DOI] [PubMed] [Google Scholar]
- Harada F., Gross H. J., Kimura F., Chang S. H., Nishimura S., RajBhandary U. L. 2-Methylthio N6-(delta 2-isopentenyl) adenosine: a component of E. coli tyrosine transfer RNA. Biochem Biophys Res Commun. 1968 Oct 24;33(2):299–306. doi: 10.1016/0006-291x(68)90784-5. [DOI] [PubMed] [Google Scholar]
- Isham K. R., Stulberg M. P. Modified nucleosides in undermethylated phenylalanine transfer RNA from Escherichia coli. Biochim Biophys Acta. 1974 Mar 8;340(2):177–182. doi: 10.1016/0005-2787(74)90110-5. [DOI] [PubMed] [Google Scholar]
- Jacobson M., Hedgcoth C. Levels of 5,6-dihydrouridine in relaxed and chloramphenicol transfer ribonucleic acid. Biochemistry. 1970 Jun 9;9(12):2513–2519. doi: 10.1021/bi00814a018. [DOI] [PubMed] [Google Scholar]
- Kline L. K., Fittler F., Hall R. H. N6-(delta-2-isopentenyl) adenosine. Biosynthesis in transfer ribonucleic acid in vitro. Biochemistry. 1969 Nov;8(11):4361–4371. doi: 10.1021/bi00839a021. [DOI] [PubMed] [Google Scholar]
- Lindahl T., Adams A., Fresco J. R. Renaturation of transfer ribonucleic acids through site binding of magnesium. Proc Natl Acad Sci U S A. 1966 Apr;55(4):941–948. doi: 10.1073/pnas.55.4.941. [DOI] [PMC free article] [PubMed] [Google Scholar]
- Mann M. B., Huang P. C. Behavior of chloramphenicol-induced phenylalanine transfer ribonucleic acid during recovery from chloramphenicol treatment in Escherichia coli. Biochemistry. 1973 Dec 18;12(26):5289–5294. doi: 10.1021/bi00750a011. [DOI] [PubMed] [Google Scholar]
- Robins M. J., Hall R. H., Thedford R. N-6-(delta-3-isopentenyl) adenosine. A component of the transfer ribonucleic acid of yeast and of mammalian tissue, methods of isolation, and characterization. Biochemistry. 1967 Jun;6(6):1837–1848. doi: 10.1021/bi00858a035. [DOI] [PubMed] [Google Scholar]
- Rosenberg A. H., Gefter M. L. An iron-dependent modification of several transfer RNA species in Escherichia coli. J Mol Biol. 1969 Dec 28;46(3):581–584. doi: 10.1016/0022-2836(69)90197-1. [DOI] [PubMed] [Google Scholar]
- Skjold A. C., Juarez H., Hedgcoth C. Relationships among deoxyribonucleic acid, ribonucleic acid, and specific transfer ribonucleic acids in Escherichia coli 15T - at various growth rates. J Bacteriol. 1973 Jul;115(1):177–187. doi: 10.1128/jb.115.1.177-187.1973. [DOI] [PMC free article] [PubMed] [Google Scholar]
- Waters L. C. Altered chromatographic properties of tRNA from chloramphenicol-treated Escherichia coli. Biochem Biophys Res Commun. 1969 Oct 8;37(2):296–304. doi: 10.1016/0006-291x(69)90734-7. [DOI] [PubMed] [Google Scholar]
- Waters L. C., Shugart L., Yang W. K., Best A. N. Some physical and biological properties of 4-thiouridine- and dihydrouridine-deficient tRNA from chloramphenicol-treated Escherichia coli. Arch Biochem Biophys. 1973 Jun;156(2):780–793. doi: 10.1016/0003-9861(73)90332-9. [DOI] [PubMed] [Google Scholar]
- Weiss J. F., Kelmers A. D. A new chromatographic system for increased resolution of transfer ribonucleic acids. Biochemistry. 1967 Aug;6(8):2507–2513. doi: 10.1021/bi00860a030. [DOI] [PubMed] [Google Scholar]
- Wettstein F. O. Differential in vivo aminoacylation and utilization of homologous species of E. coli transfer RNA. Cold Spring Harb Symp Quant Biol. 1966;31:595–599. doi: 10.1101/sqb.1966.031.01.077. [DOI] [PubMed] [Google Scholar]
- Wettstein F. O., Stent G. S. Physiologically induced changes in the property of phenylalanine tRNA in Escherichia coli. J Mol Biol. 1968 Nov 28;38(1):25–40. doi: 10.1016/0022-2836(68)90126-5. [DOI] [PubMed] [Google Scholar]