Abstract
While many theories of general anesthesia postulate a lipid site of action, there has been no adequate explanation for the lack of anesthetic potency of the highly hydrophobic primary alkanols with more than 12 carbons (the cut-off). Some work suggests that these nonanesthetic alcohols do not dissolve in membranes. Other work contradicts this and suggests that an anesthetic site on a protein provides a better explanation. Here we show that both the anesthetic dodecanol and the nonanesthetic tetradecanol are taken up equally well into the tissues of animals and into isolated postsynaptic membranes. When a group of Rana pipiens tadpoles were treated with dodecanol, half were anesthetized by 4.7 microM (free aqueos concentration), and the corresponding concentration in the tissues was found to be 0.4 mmol per kg wet weight. Prolonged exposure (92 hr) to tetradecanol produced even higher tissue concentrations (0.7 mmol per kg wet weight), yet no anesthetic effects were observed. Furthermore, general anesthetics are thought to act on postsynaptic membranes but both alkanols partitioned into postsynaptic membranes from Torpedo electroplaques. The spin label, 12-doxyl stearate, was incorporated into these membranes. The lipid order parameter it reported was decreased by the anesthetic alcohols (octanol, decanol, and dodecanol), whereas the nonanesthetic alcohols either did not change it significantly (tetradecanol) or actually increased it (hexadecanol and octadecanol). Thus, although lipid solubility is unable to account for the pharmacology of the cut-off in potency of the long-chain alcohols, lipid perturbations provide an accurate description.
Full text
PDF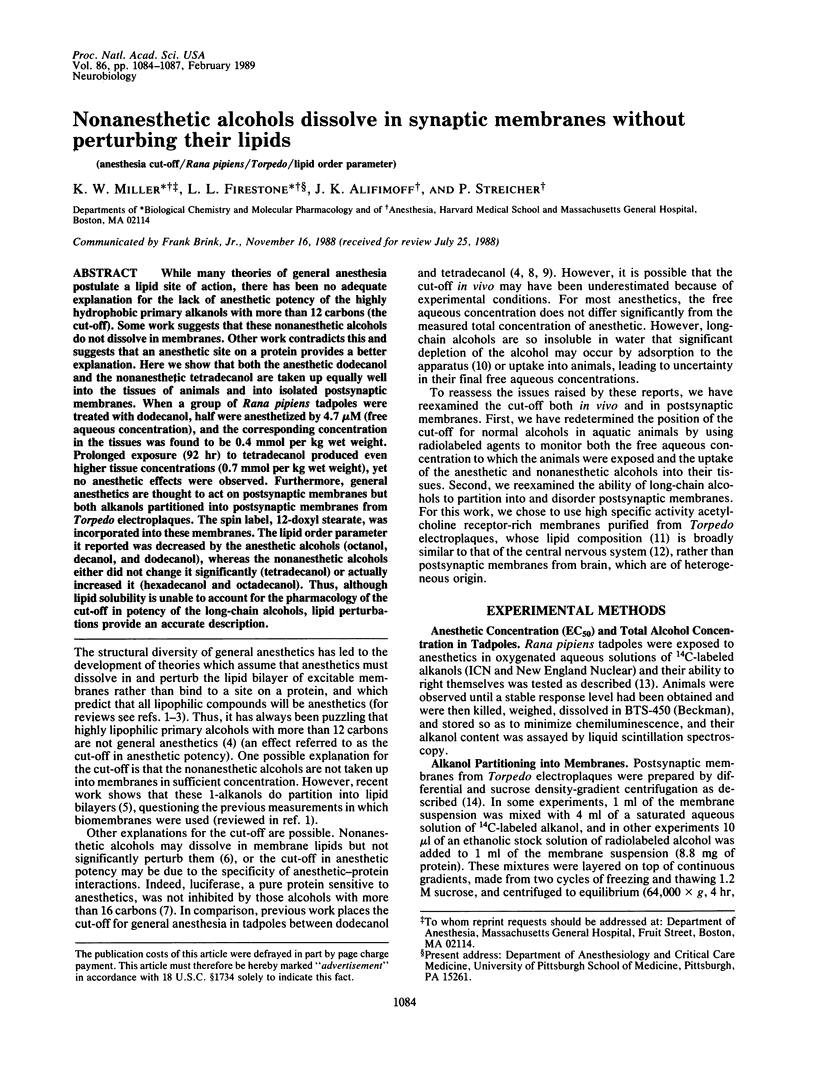
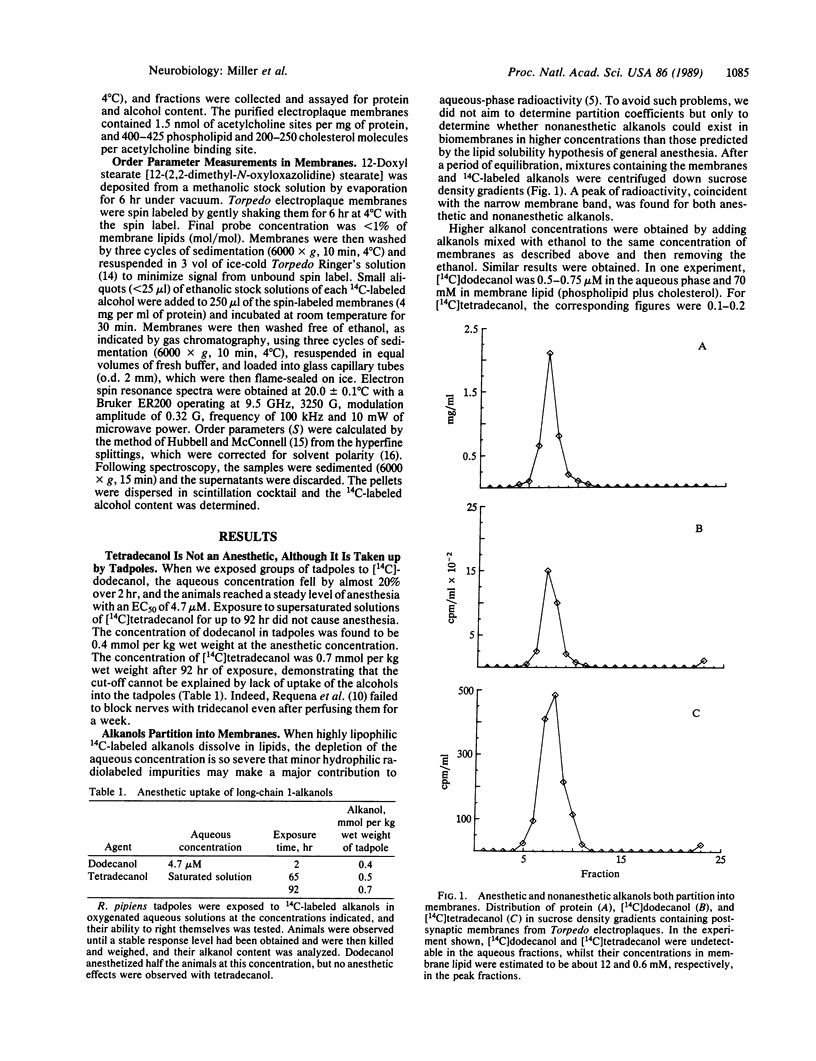
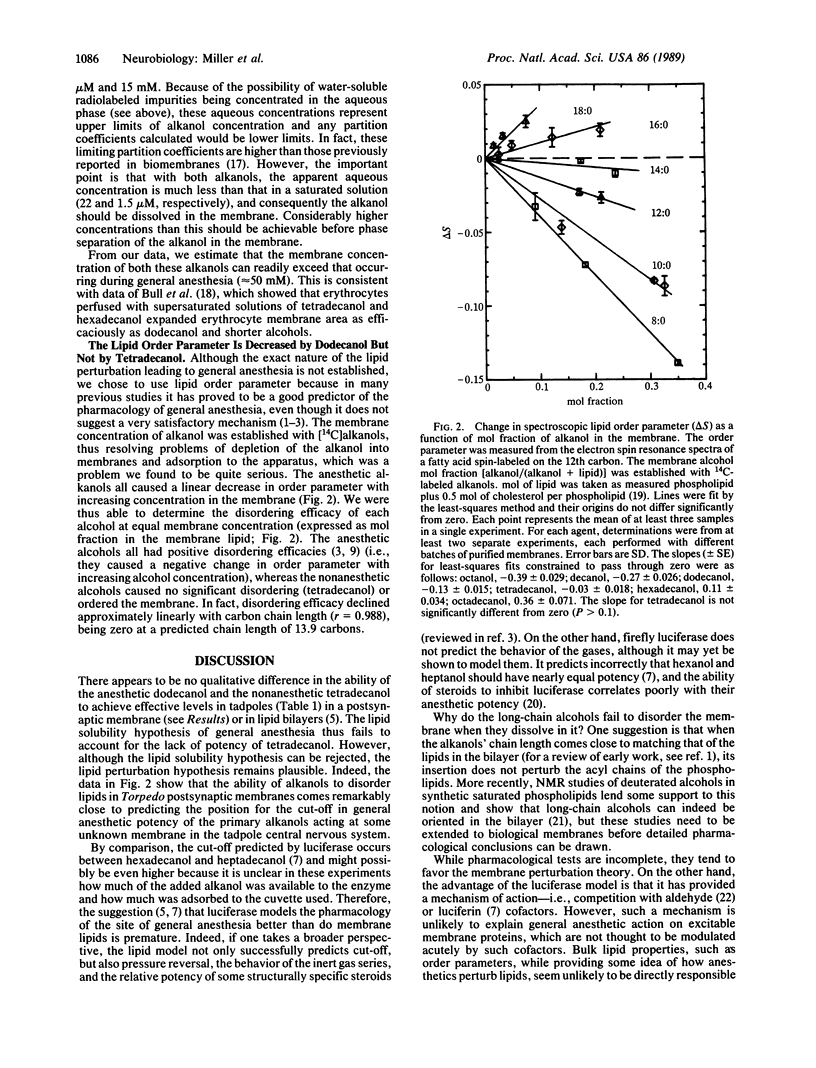
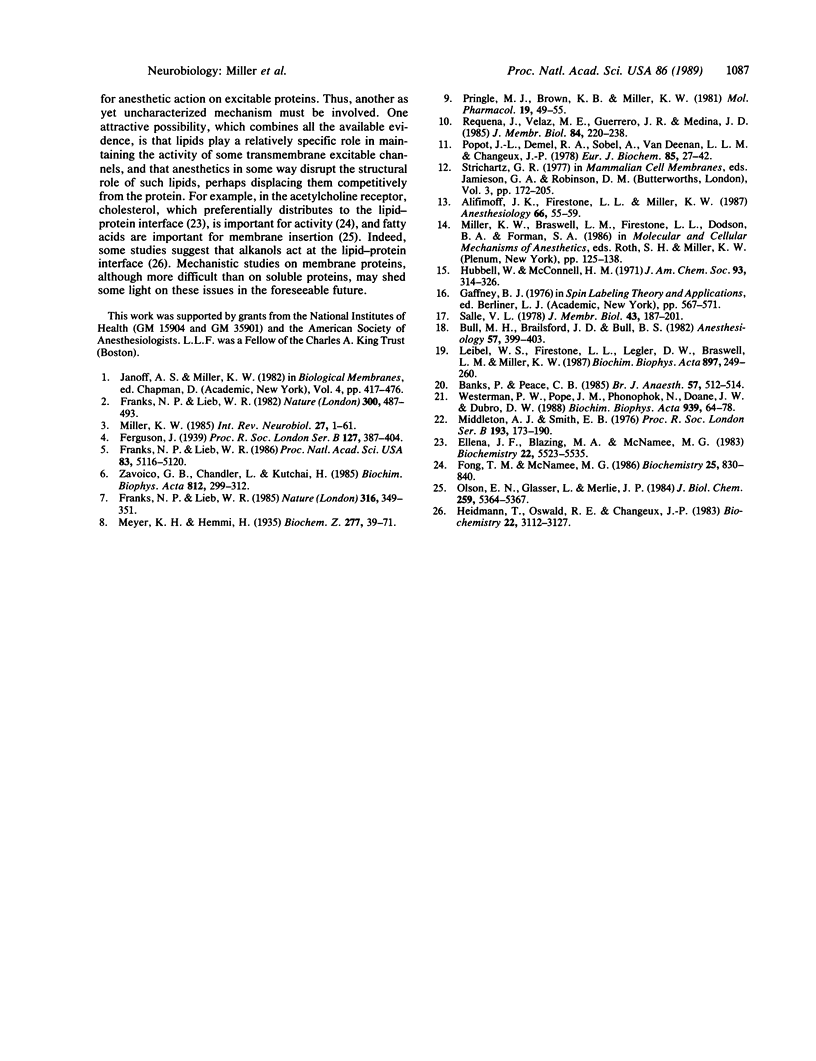
Selected References
These references are in PubMed. This may not be the complete list of references from this article.
- Alifimoff J. K., Firestone L. L., Miller K. W. Anesthetic potencies of secondary alcohol enantiomers. Anesthesiology. 1987 Jan;66(1):55–59. doi: 10.1097/00000542-198701000-00010. [DOI] [PubMed] [Google Scholar]
- Banks P., Peace C. B. Enzyme inhibition by steroid anaesthetic agents derived from progesterone. Br J Anaesth. 1985 May;57(5):512–514. doi: 10.1093/bja/57.5.512. [DOI] [PubMed] [Google Scholar]
- Bull M. H., Brailsford J. D., Bull B. S. Erythrocyte membrane expansion due to the volatile anesthetics, the 1-alkanols, and benzyl alcohol. Anesthesiology. 1982 Nov;57(5):399–403. doi: 10.1097/00000542-198211000-00008. [DOI] [PubMed] [Google Scholar]
- Ellena J. F., Blazing M. A., McNamee M. G. Lipid-protein interactions in reconstituted membranes containing acetylcholine receptor. Biochemistry. 1983 Nov 22;22(24):5523–5535. doi: 10.1021/bi00293a012. [DOI] [PubMed] [Google Scholar]
- Fong T. M., McNamee M. G. Correlation between acetylcholine receptor function and structural properties of membranes. Biochemistry. 1986 Feb 25;25(4):830–840. doi: 10.1021/bi00352a015. [DOI] [PubMed] [Google Scholar]
- Franks N. P., Lieb W. R. Mapping of general anaesthetic target sites provides a molecular basis for cutoff effects. Nature. 1985 Jul 25;316(6026):349–351. doi: 10.1038/316349a0. [DOI] [PubMed] [Google Scholar]
- Franks N. P., Lieb W. R. Molecular mechanisms of general anaesthesia. Nature. 1982 Dec 9;300(5892):487–493. doi: 10.1038/300487a0. [DOI] [PubMed] [Google Scholar]
- Franks N. P., Lieb W. R. Partitioning of long-chain alcohols into lipid bilayers: implications for mechanisms of general anesthesia. Proc Natl Acad Sci U S A. 1986 Jul;83(14):5116–5120. doi: 10.1073/pnas.83.14.5116. [DOI] [PMC free article] [PubMed] [Google Scholar]
- Heidmann T., Oswald R. E., Changeux J. P. Multiple sites of action for noncompetitive blockers on acetylcholine receptor rich membrane fragments from torpedo marmorata. Biochemistry. 1983 Jun 21;22(13):3112–3127. doi: 10.1021/bi00282a014. [DOI] [PubMed] [Google Scholar]
- Hubbell W. L., McConnell H. M. Molecular motion in spin-labeled phospholipids and membranes. J Am Chem Soc. 1971 Jan 27;93(2):314–326. doi: 10.1021/ja00731a005. [DOI] [PubMed] [Google Scholar]
- Leibel W. S., Firestone L. L., Legler D. C., Braswell L. M., Miller K. W. Two pools of cholesterol in acetylcholine receptor-rich membranes from Torpedo. Biochim Biophys Acta. 1987 Feb 26;897(2):249–260. doi: 10.1016/0005-2736(87)90421-4. [DOI] [PubMed] [Google Scholar]
- Middleton A. J., Smith E. B. General anaesthetics and bacterial luminescence. II. The effect of diethyl ether on the in vitro light emission of Vibrio fischeri. Proc R Soc Lond B Biol Sci. 1976 Apr 13;193(1111):173–190. doi: 10.1098/rspb.1976.0038. [DOI] [PubMed] [Google Scholar]
- Miller K. W. The nature of the site of general anesthesia. Int Rev Neurobiol. 1985;27:1–61. doi: 10.1016/s0074-7742(08)60555-3. [DOI] [PubMed] [Google Scholar]
- Olson E. N., Glaser L., Merlie J. P. Alpha and beta subunits of the nicotinic acetylcholine receptor contain covalently bound lipid. J Biol Chem. 1984 May 10;259(9):5364–5367. [PubMed] [Google Scholar]
- Popot J. L., Demel R. A., Sobel A., Van Deenen L. L., Changeux J. P. Interaction of the acetylcholine (nicotinic) receptor protein from Torpedo marmorata electric organ with monolayers of pure lipids. Eur J Biochem. 1978 Apr;85(1):27–42. doi: 10.1111/j.1432-1033.1978.tb12209.x. [DOI] [PubMed] [Google Scholar]
- Pringle M. J., Brown K. B., Miller K. W. Can the lipid theories of anesthesia account for the cutoff in anesthetic potency in homologous series of alcohols? Mol Pharmacol. 1981 Jan;19(1):49–55. [PubMed] [Google Scholar]
- Requena J., Velaz M. E., Guerrero J. R., Medina J. D. Isomers of long-chain alkane derivatives and nervous impulse blockage. J Membr Biol. 1985;84(3):229–238. doi: 10.1007/BF01871386. [DOI] [PubMed] [Google Scholar]
- Sallee V. L. Fatty acid and alcohol partitioning with intestinal brush border and erythrocyte membranes. J Membr Biol. 1978 Oct 19;43(2-3):187–201. doi: 10.1007/BF01933478. [DOI] [PubMed] [Google Scholar]
- Westerman P. W., Pope J. M., Phonphok N., Doane J. W., Dubro D. W. The interaction of n-alkanols with lipid bilayer membranes: a 2H-NMR study. Biochim Biophys Acta. 1988 Mar 22;939(1):64–78. doi: 10.1016/0005-2736(88)90048-x. [DOI] [PubMed] [Google Scholar]
- Zavoico G. B., Chandler L., Kutchai H. Perturbation of egg phosphatidylcholine and dipalmitoylphosphatidylcholine multilamellar vesicles by n-alkanols. A fluorescent probe study. Biochim Biophys Acta. 1985 Jan 25;812(2):299–312. doi: 10.1016/0005-2736(85)90304-9. [DOI] [PubMed] [Google Scholar]