Abstract
Cholinergic deficits in Alzheimer's disease are typically assessed by choline acetyltransferase, the enzyme that synthesizes acetylcholine. However, the determining step in acetylcholine formation is choline uptake via a high affinity transporter in nerve terminal membranes. Evaluating uptake is difficult because regulatory changes in transporter function decay rapidly postmortem. To overcome this problem, brain regions from patients with or without Alzheimer's disease were frozen within 4 h of death and examined for both choline acetyltransferase activity and for binding of [3H]-hemicholinium-3 to the choline transporter. Consistent with the loss of cholinergic projections, cerebral cortical areas exhibited marked decreases in enzyme activity whereas the putamen, a region not involved in Alzheimer's disease, was unaffected. However, [3H]hemicholinium-3 binding was significantly enhanced in the cortical regions. In the frontal cortex, the increase in [3H]hemicholinium-3 binding far exceeded the loss of choline acetyltransferase, indicating transporter overexpression beyond that necessary to offset loss of synaptic terminals. These results suggest that, in Alzheimer's disease, the loss of cholinergic function is not dictated simply by destruction of nerve terminals, but rather involves additional alterations in choline utilization; interventions aimed at increasing the activity of cholinergic neurons may thus accelerate neurodegeneration.
Full text
PDF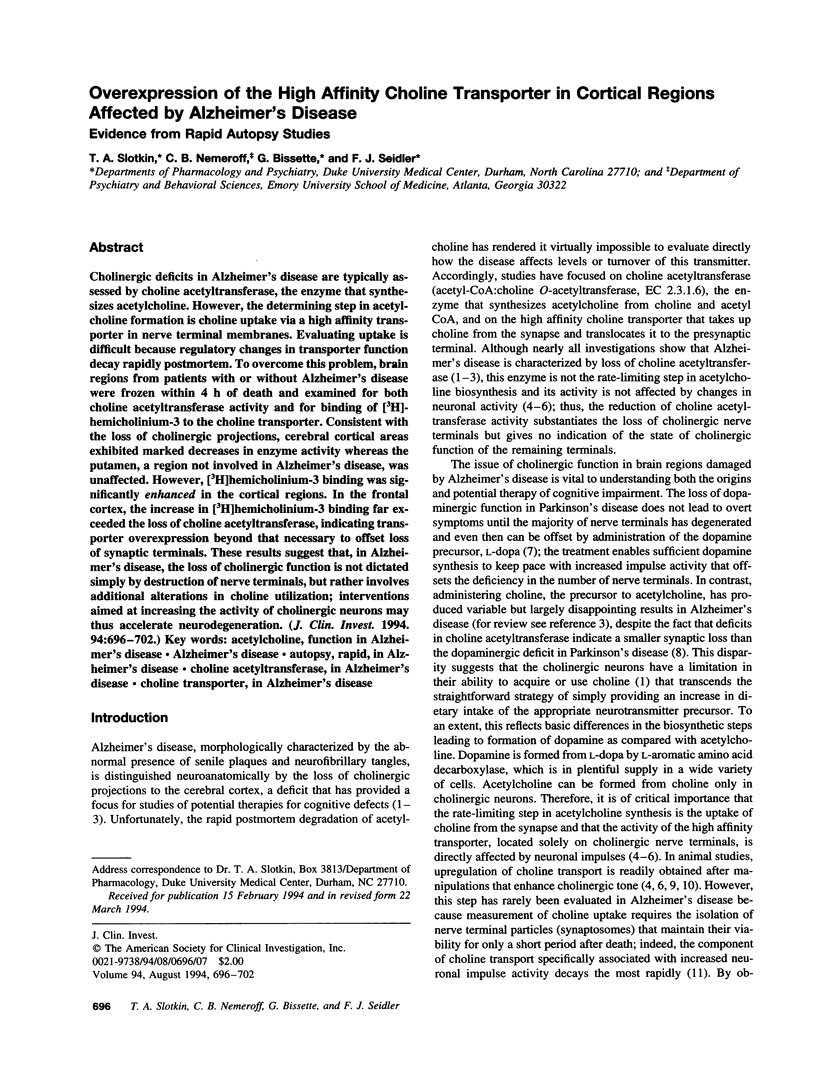
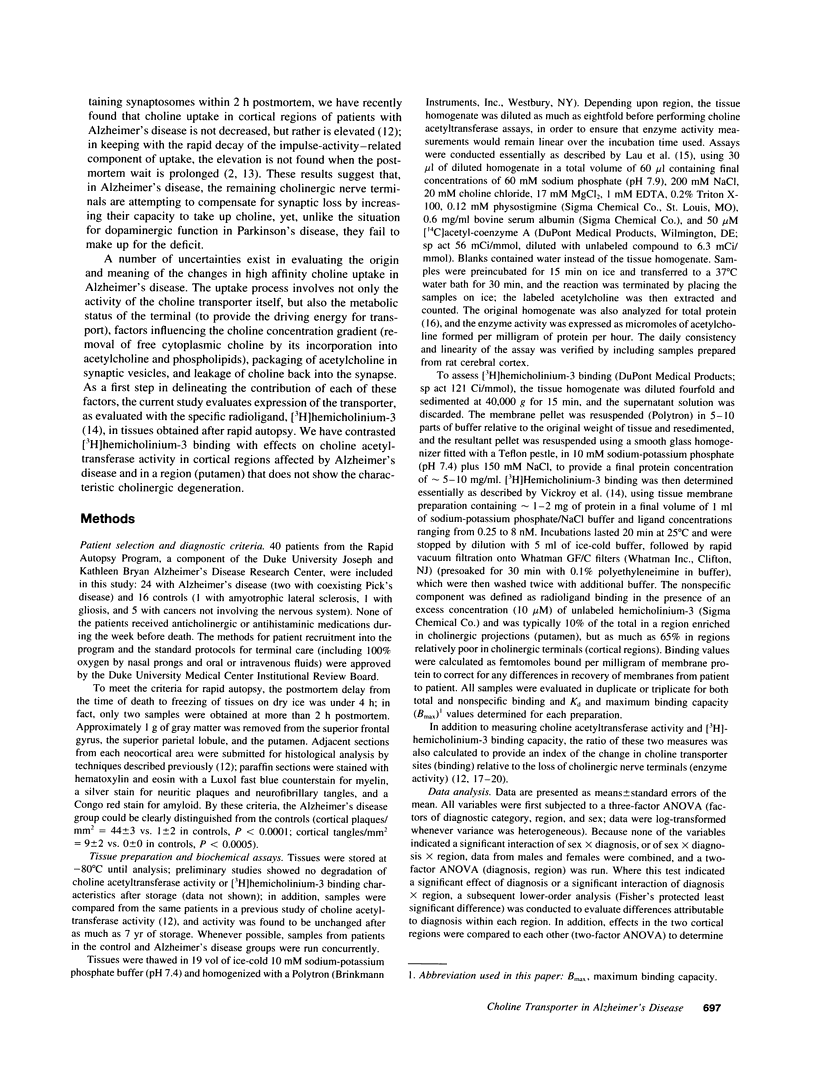
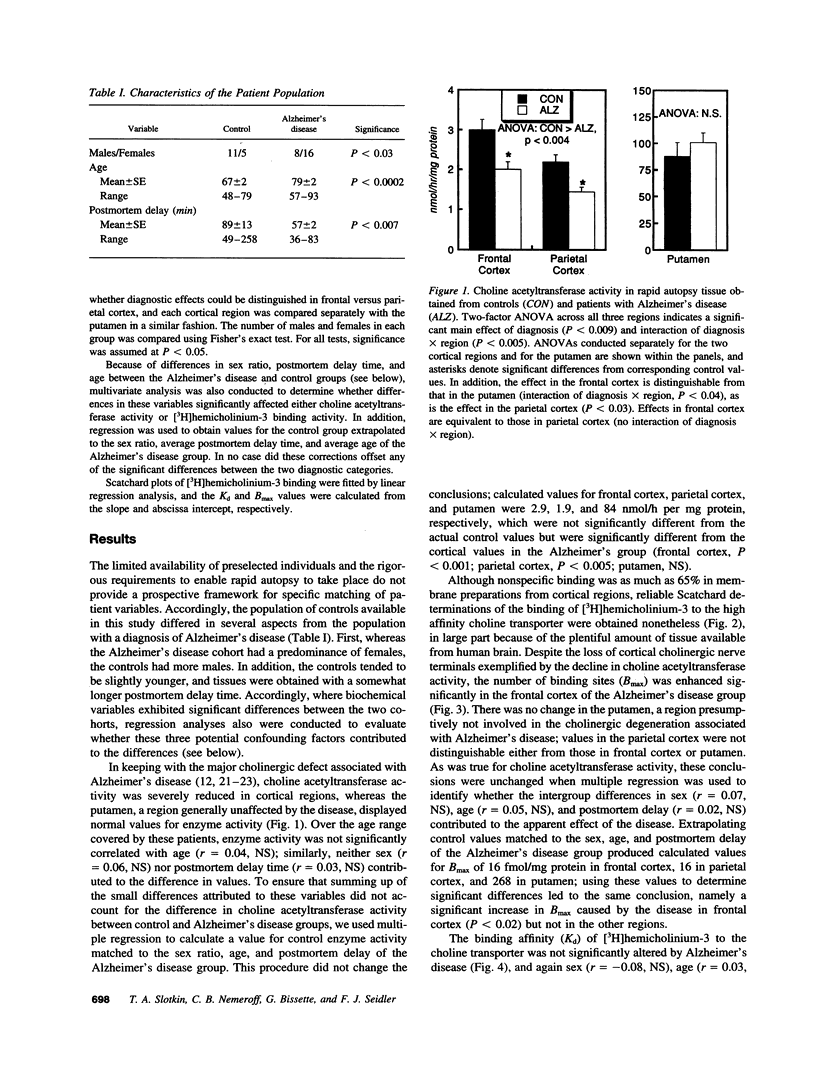
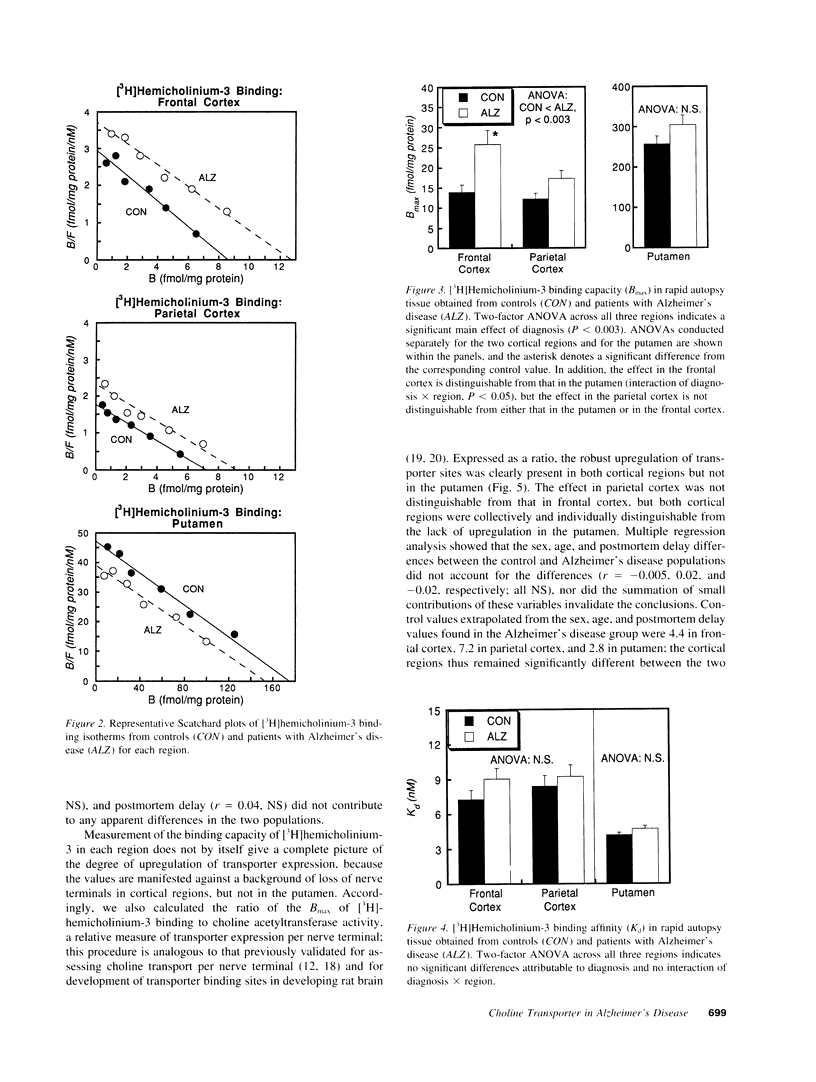
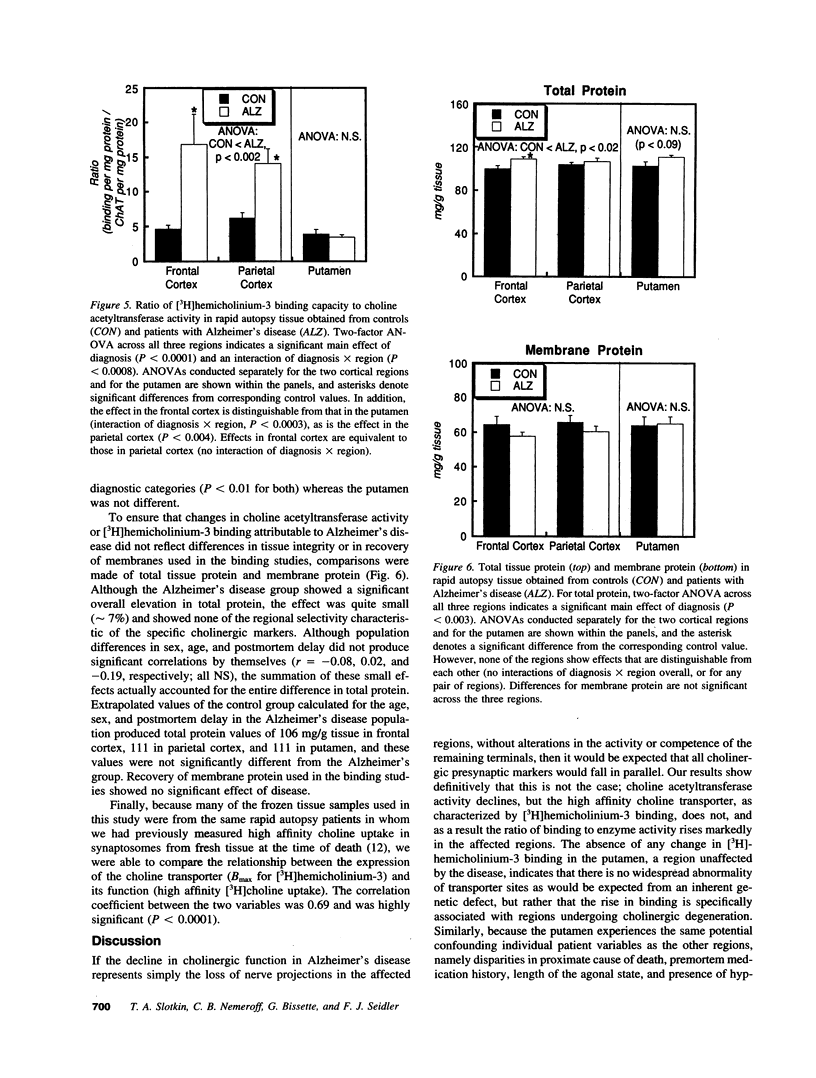
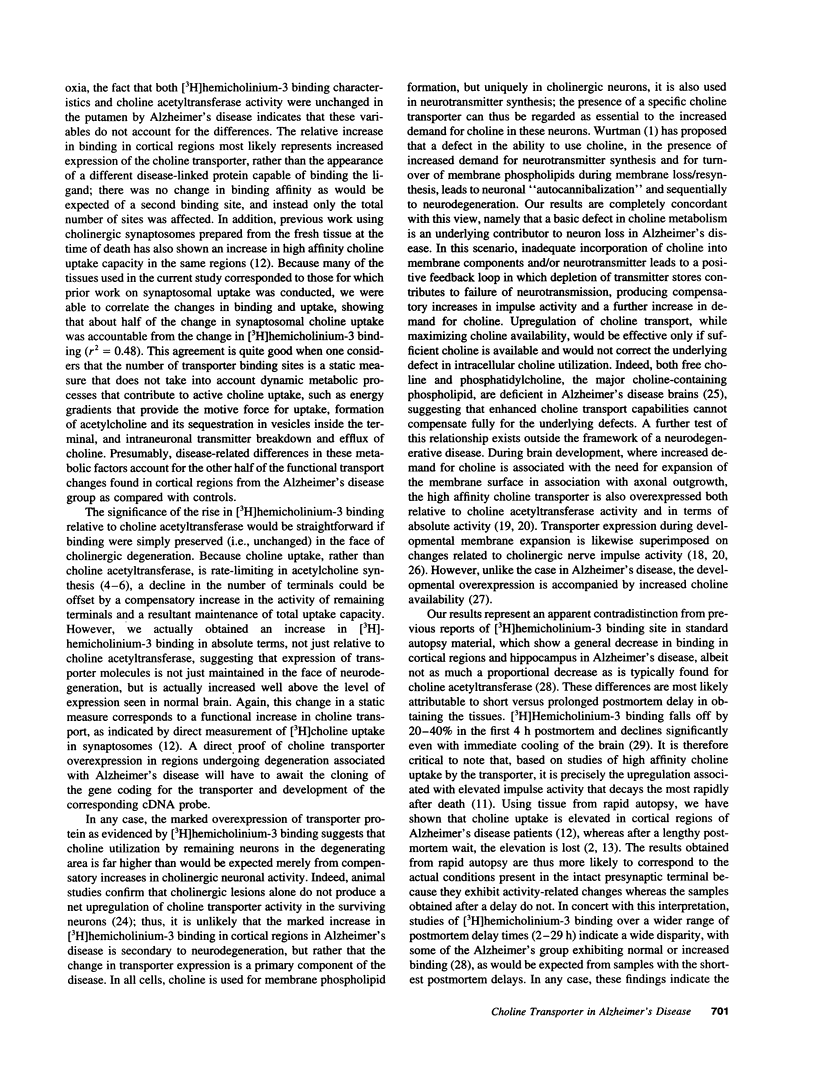
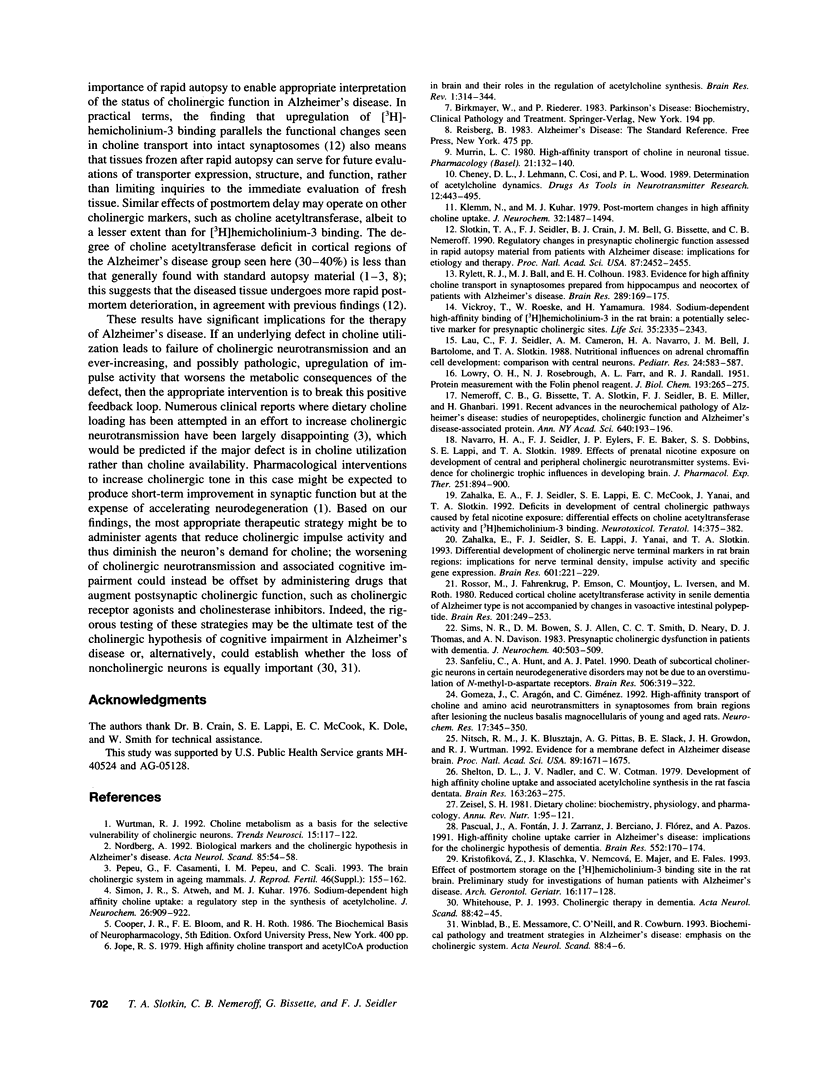
Selected References
These references are in PubMed. This may not be the complete list of references from this article.
- Gomeza J., Aragón C., Giménez C. High-affinity transport of choline and amino acid neurotransmitters in synaptosomes from brain regions after lesioning the nucleus basalis magnocellularis of young and aged rats. Neurochem Res. 1992 Apr;17(4):345–350. doi: 10.1007/BF00974576. [DOI] [PubMed] [Google Scholar]
- Jope R. S. High affinity choline transport and acetylCoA production in brain and their roles in the regulation of acetylcholine synthesis. Brain Res. 1979 Dec;180(3):313–344. doi: 10.1016/0165-0173(79)90009-2. [DOI] [PubMed] [Google Scholar]
- Klemm N., Kuhar M. J. Post-mortem changes in high affinity choline uptake. J Neurochem. 1979 May;32(5):1487–1494. doi: 10.1111/j.1471-4159.1979.tb11089.x. [DOI] [PubMed] [Google Scholar]
- Kristofiková Z., Klaschka J., Nemcová V., Majer E., Fales E. Effect of postmortem storage on the [3H]hemicholinium-3 binding site in the rat brain. Preliminary study for investigations of human patients with Alzheimer's disease. Arch Gerontol Geriatr. 1993 Mar-Apr;16(2):117–128. doi: 10.1016/0167-4943(93)90003-z. [DOI] [PubMed] [Google Scholar]
- LOWRY O. H., ROSEBROUGH N. J., FARR A. L., RANDALL R. J. Protein measurement with the Folin phenol reagent. J Biol Chem. 1951 Nov;193(1):265–275. [PubMed] [Google Scholar]
- Lau C., Seidler F. J., Cameron A. M., Navarro H. A., Bell J. M., Bartolome J., Slotkin T. A. Nutritional influences on adrenal chromaffin cell development: comparison with central neurons. Pediatr Res. 1988 Nov;24(5):583–587. doi: 10.1203/00006450-198811000-00009. [DOI] [PubMed] [Google Scholar]
- Murrin L. C. High-affinity transport of choline in neuronal tissue. Pharmacology. 1980;21(2):132–140. doi: 10.1159/000137425. [DOI] [PubMed] [Google Scholar]
- Navarro H. A., Seidler F. J., Eylers J. P., Baker F. E., Dobbins S. S., Lappi S. E., Slotkin T. A. Effects of prenatal nicotine exposure on development of central and peripheral cholinergic neurotransmitter systems. Evidence for cholinergic trophic influences in developing brain. J Pharmacol Exp Ther. 1989 Dec;251(3):894–900. [PubMed] [Google Scholar]
- Nemeroff C. B., Bissette G., Slotkin T. A., Seidler F. J., Miller B. E., Ghanbari H. Recent advances in the neurochemical pathology of Alzheimer's disease. Studies of neuropeptides, cholinergic function and Alzheimer's disease-associated protein. Ann N Y Acad Sci. 1991;640:193–196. doi: 10.1111/j.1749-6632.1991.tb00216.x. [DOI] [PubMed] [Google Scholar]
- Nitsch R. M., Blusztajn J. K., Pittas A. G., Slack B. E., Growdon J. H., Wurtman R. J. Evidence for a membrane defect in Alzheimer disease brain. Proc Natl Acad Sci U S A. 1992 Mar 1;89(5):1671–1675. doi: 10.1073/pnas.89.5.1671. [DOI] [PMC free article] [PubMed] [Google Scholar]
- Nordberg A. Biological markers and the cholinergic hypothesis in Alzheimer's disease. Acta Neurol Scand Suppl. 1992;139:54–58. doi: 10.1111/j.1600-0404.1992.tb04455.x. [DOI] [PubMed] [Google Scholar]
- Pascual J., Fontán A., Zarranz J. J., Berciano J., Flórez J., Pazos A. High-affinity choline uptake carrier in Alzheimer's disease: implications for the cholinergic hypothesis of dementia. Brain Res. 1991 Jun 21;552(1):170–174. doi: 10.1016/0006-8993(91)90676-m. [DOI] [PubMed] [Google Scholar]
- Pepeu G., Casamenti F., Pepeu I. M., Scali C. The brain cholinergic system in ageing mammals. J Reprod Fertil Suppl. 1993;46:155–162. [PubMed] [Google Scholar]
- Rossor M., Fahrenkrug J., Emson P., Mountjoy C., Iversen L., Roth M. Reduced cortical choline acetyltransferase activity in senile dementia of Alzheimer type is not accompanied by changes in vasoactive intestinal polypeptide. Brain Res. 1980 Nov 10;201(1):249–253. doi: 10.1016/0006-8993(80)90795-7. [DOI] [PubMed] [Google Scholar]
- Rylett R. J., Ball M. J., Colhoun E. H. Evidence for high affinity choline transport in synaptosomes prepared from hippocampus and neocortex of patients with Alzheimer's disease. Brain Res. 1983 Dec 19;289(1-2):169–175. doi: 10.1016/0006-8993(83)90017-3. [DOI] [PubMed] [Google Scholar]
- Sanfeliu C., Hunt A., Patel A. J. Death of subcortical cholinergic neurons in certain neurodegenerative disorders may not be due to an overstimulation of N-methyl-D-aspartate receptors. Brain Res. 1990 Jan 8;506(2):319–322. doi: 10.1016/0006-8993(90)91271-h. [DOI] [PubMed] [Google Scholar]
- Shelton D. L., Nadler J. V., Cotman C. W. Development of high affinity choline uptake and associated acetylcholine synthesis in the rat fascia dentata. Brain Res. 1979 Mar 16;163(2):263–275. doi: 10.1016/0006-8993(79)90354-8. [DOI] [PubMed] [Google Scholar]
- Simon J. R., Atweh S., Kuhar M. J. Sodium-dependent high affinity choline uptake: a regulatory step in the synthesis of acetylcholine. J Neurochem. 1976 May;26(5):909–922. doi: 10.1111/j.1471-4159.1976.tb06472.x. [DOI] [PubMed] [Google Scholar]
- Sims N. R., Bowen D. M., Allen S. J., Smith C. C., Neary D., Thomas D. J., Davison A. N. Presynaptic cholinergic dysfunction in patients with dementia. J Neurochem. 1983 Feb;40(2):503–509. doi: 10.1111/j.1471-4159.1983.tb11311.x. [DOI] [PubMed] [Google Scholar]
- Slotkin T. A., Seidler F. J., Crain B. J., Bell J. M., Bissette G., Nemeroff C. B. Regulatory changes in presynaptic cholinergic function assessed in rapid autopsy material from patients with Alzheimer disease: implications for etiology and therapy. Proc Natl Acad Sci U S A. 1990 Apr;87(7):2452–2455. doi: 10.1073/pnas.87.7.2452. [DOI] [PMC free article] [PubMed] [Google Scholar]
- Vickroy T. W., Roeske W. R., Yamamura H. I. Sodium-dependent high-affinity binding of [3H]hemicholinium-3 in the rat brain: a potentially selective marker for presynaptic cholinergic sites. Life Sci. 1984 Dec 3;35(23):2335–2343. doi: 10.1016/0024-3205(84)90525-3. [DOI] [PubMed] [Google Scholar]
- Winblad B., Messamore E., O'Neill C., Cowburn R. Biochemical pathology and treatment strategies in Alzheimer's disease: emphasis on the cholinergic system. Acta Neurol Scand Suppl. 1993;149:4–6. doi: 10.1111/j.1600-0404.1993.tb04244.x. [DOI] [PubMed] [Google Scholar]
- Wurtman R. J. Choline metabolism as a basis for the selective vulnerability of cholinergic neurons. Trends Neurosci. 1992 Apr;15(4):117–122. doi: 10.1016/0166-2236(92)90351-8. [DOI] [PubMed] [Google Scholar]
- Zahalka E. A., Seidler F. J., Lappi S. E., McCook E. C., Yanai J., Slotkin T. A. Deficits in development of central cholinergic pathways caused by fetal nicotine exposure: differential effects on choline acetyltransferase activity and [3H]hemicholinium-3 binding. Neurotoxicol Teratol. 1992 Nov-Dec;14(6):375–382. doi: 10.1016/0892-0362(92)90047-e. [DOI] [PubMed] [Google Scholar]
- Zahalka E. A., Seidler F. J., Lappi S. E., Yanai J., Slotkin T. A. Differential development of cholinergic nerve terminal markers in rat brain regions: implications for nerve terminal density, impulse activity and specific gene expression. Brain Res. 1993 Jan 22;601(1-2):221–229. doi: 10.1016/0006-8993(93)91714-4. [DOI] [PubMed] [Google Scholar]
- Zeisel S. H. Dietary choline: biochemistry, physiology, and pharmacology. Annu Rev Nutr. 1981;1:95–121. doi: 10.1146/annurev.nu.01.070181.000523. [DOI] [PubMed] [Google Scholar]