Abstract
The effect of positive and negative stress on myosin cross-bridge orientation in glycerinated muscle fibers was investigated by using fluorescence polarization spectroscopy of the emission from the covalent label tetramethyl-rhodamine-5-(and -6)-iodoacetamide (IATR) specifically modifying sulfhydryl one (SH1) on the myosin heavy chain. Positive tension was applied by stretching the fiber in rigor. Negative tension was applied in two steps by using a protocol introduced by Goldman et al. [Goldman, Y. E., McCray, J. A. & Vallette, D. P. (1988) J. Physiol. (London) 398, 75P]: relaxing a fiber at resting length and stretching it until the relaxed tension is appreciable and then placing the fiber in rigor and releasing the tension onto the rigor cross-bridges. We found, as have others, that positive tension has no effect on the fluorescence polarization spectrum from the SH1-bound probe, indicating that the cross-bridge does not rotate under these conditions. Negative tension, however, causes a change in the fluorescence polarization spectrum that indicates a probe rotation. The changes in the polarization spectrum from negative stress are partially reversed by the subsequent application of positive stress. It appears that negative tension strains the cross-bridge, or the cross-bridge domain containing SH1, and causes it to rotate.
Full text
PDF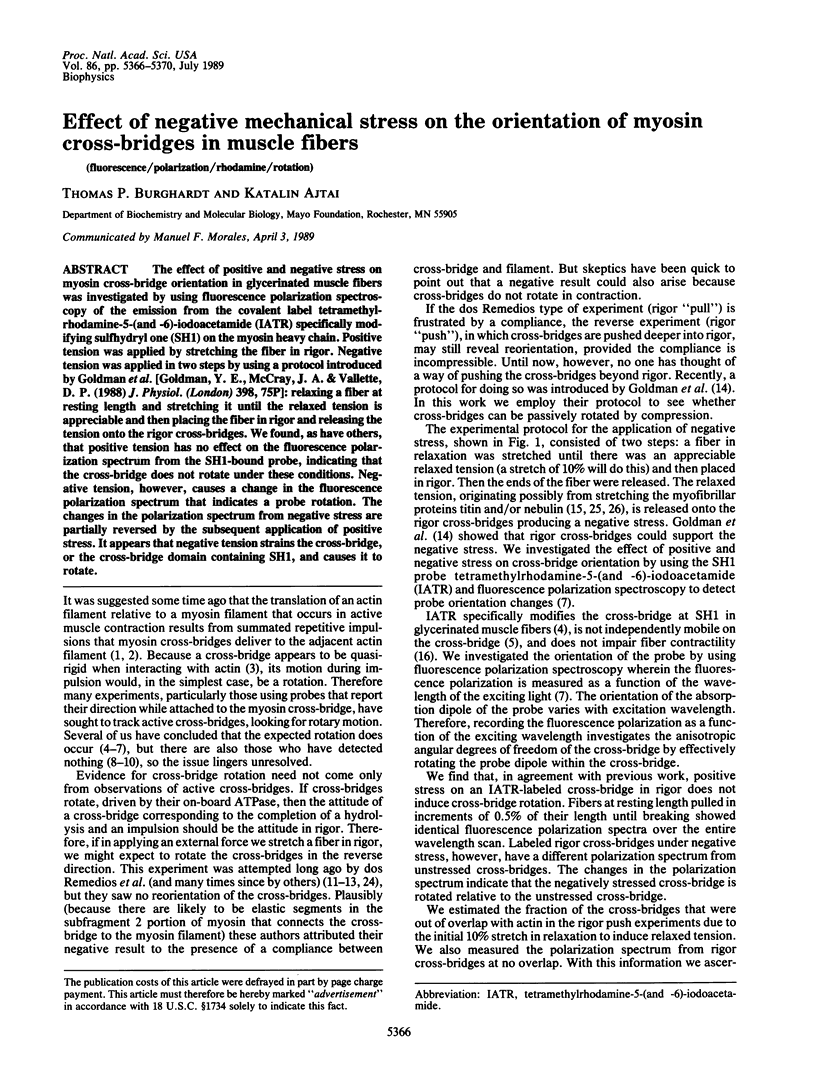
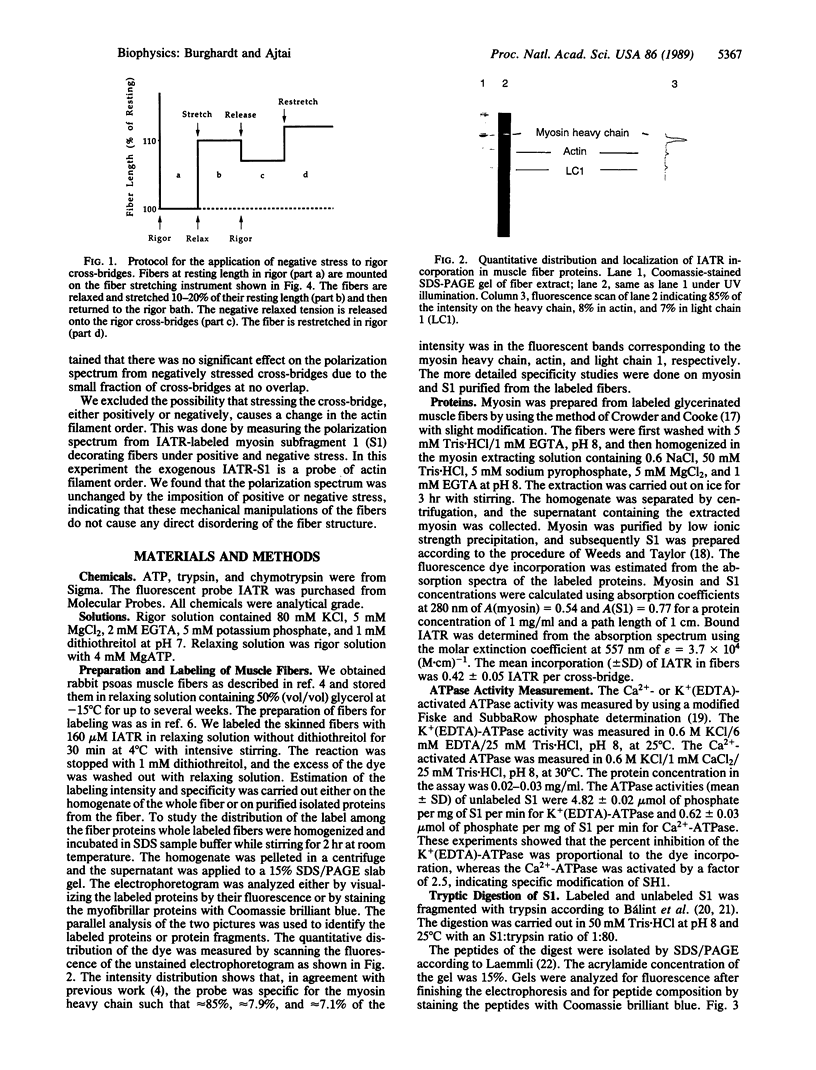
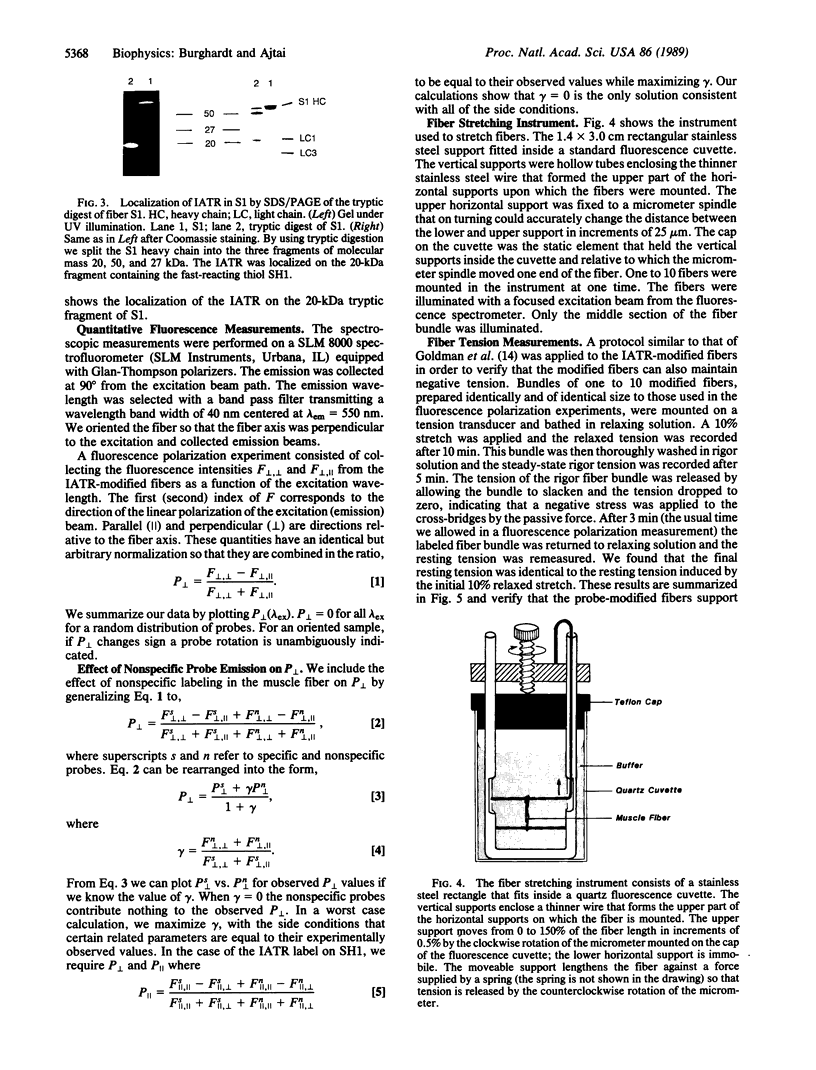
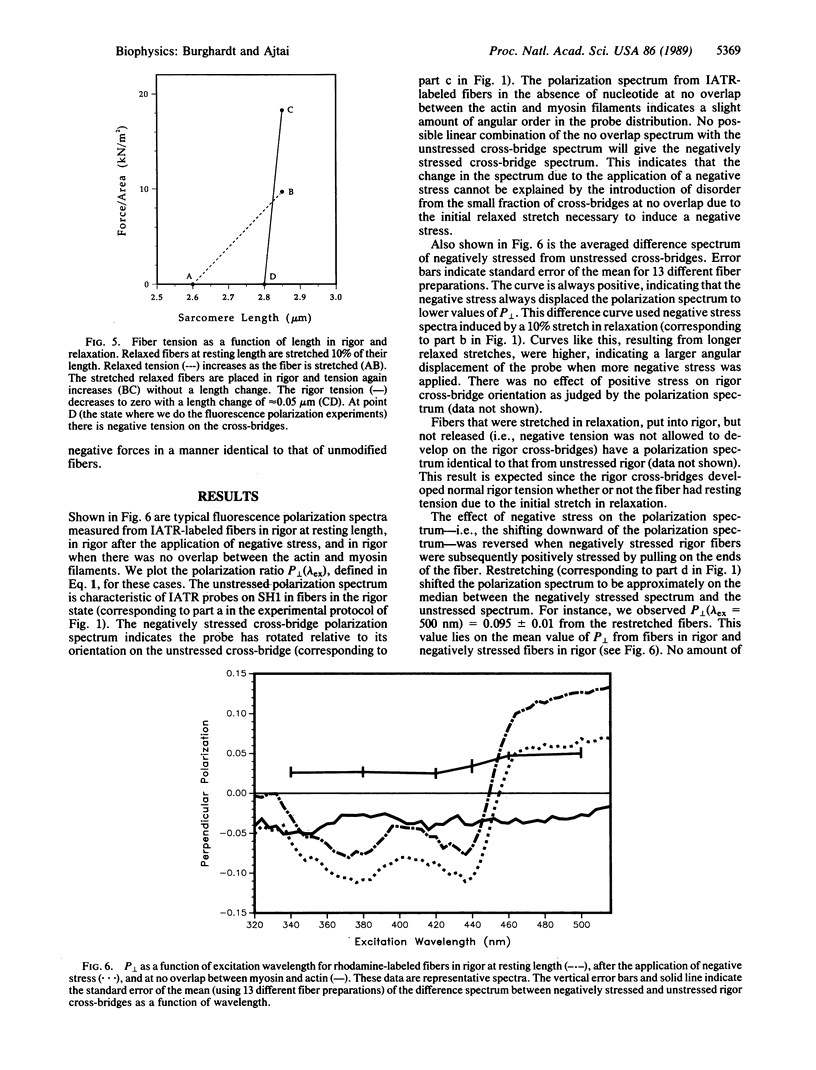
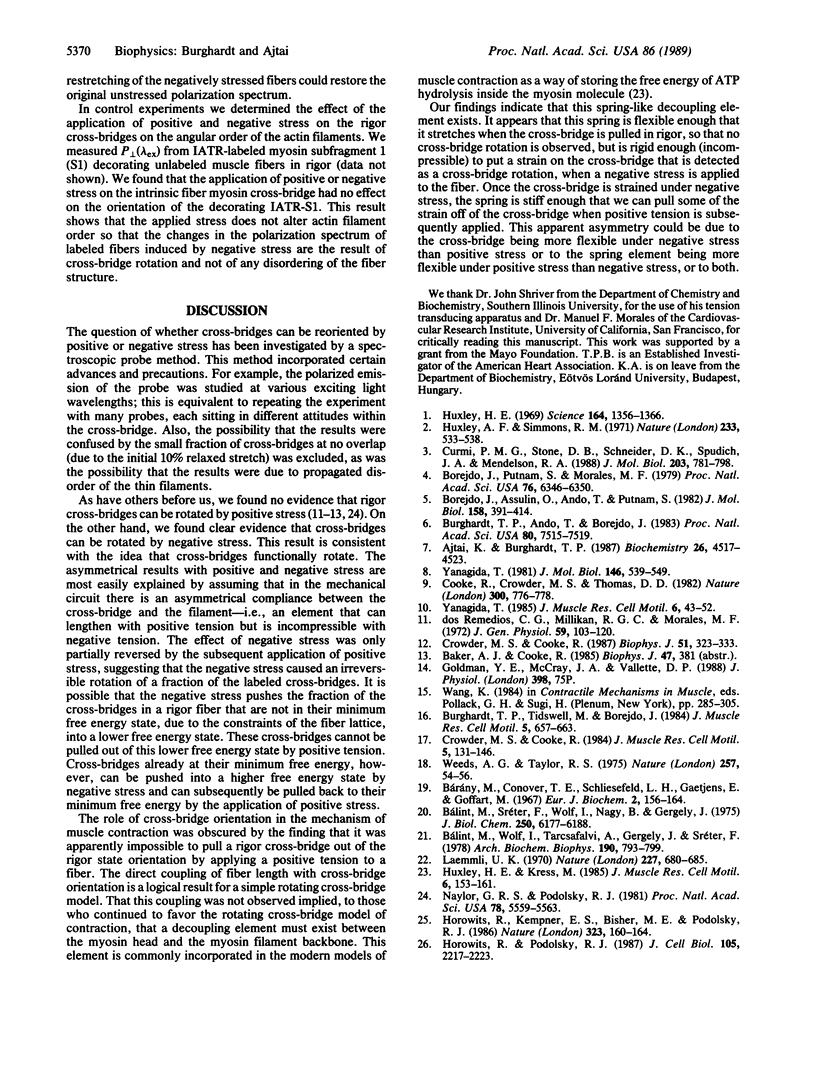
Images in this article
Selected References
These references are in PubMed. This may not be the complete list of references from this article.
- Ajtai K., Burghardt T. P. Probe studies of the MgADP state of muscle cross-bridges: microscopic and wavelength-dependent fluorescence polarization from 1,5-IAEDANS-labeled myosin subfragment 1 decorating muscle fibers. Biochemistry. 1987 Jul 14;26(14):4517–4523. doi: 10.1021/bi00388a052. [DOI] [PubMed] [Google Scholar]
- Borejdo J., Assulin O., Ando T., Putnam S. Cross-bridge orientation in skeletal muscle measured by linear dichroism of an extrinsic chromophore. J Mol Biol. 1982 Jul 5;158(3):391–414. doi: 10.1016/0022-2836(82)90205-4. [DOI] [PubMed] [Google Scholar]
- Borejdo J., Putnam S., Morales M. F. Fluctuations in polarized fluorescence: evidence that muscle cross bridges rotate repetitively during contraction. Proc Natl Acad Sci U S A. 1979 Dec;76(12):6346–6350. doi: 10.1073/pnas.76.12.6346. [DOI] [PMC free article] [PubMed] [Google Scholar]
- Burghardt T. P., Ando T., Borejdo J. Evidence for cross-bridge order in contraction of glycerinated skeletal muscle. Proc Natl Acad Sci U S A. 1983 Dec;80(24):7515–7519. doi: 10.1073/pnas.80.24.7515. [DOI] [PMC free article] [PubMed] [Google Scholar]
- Burghardt T. P., Tidswell M., Borejdo J. Crossbridge order and orientation in resting single glycerinated muscle fibres studied by linear dichroism of bound rhodamine labels. J Muscle Res Cell Motil. 1984 Dec;5(6):657–663. doi: 10.1007/BF00713924. [DOI] [PubMed] [Google Scholar]
- Bálint M., Sréter F. A., Wolf I., Nagy B., Gergely J. The substructure of heavy meromyosin. The effect of Ca2+ and Mg2+ on the tryptic fragmentation of heavy meromyosin. J Biol Chem. 1975 Aug 10;250(15):6168–6177. [PubMed] [Google Scholar]
- Bálint M., Wolf I., Tarcsafalvi A., Gergely J., Sréter F. A. Location of SH-1 and SH-2 in the heavy chain segment of heavy meromyosin. Arch Biochem Biophys. 1978 Oct;190(2):793–799. doi: 10.1016/0003-9861(78)90339-9. [DOI] [PubMed] [Google Scholar]
- Bárány M., Conover T. E., Schliselfeld L. H., Gaetjens E., Goffart M. Relation of properties of isolated myosin to those of intact muscles of the cat and sloth. Eur J Biochem. 1967 Sep;2(2):156–164. doi: 10.1111/j.1432-1033.1967.tb00120.x. [DOI] [PubMed] [Google Scholar]
- Cooke R., Crowder M. S., Thomas D. D. Orientation of spin labels attached to cross-bridges in contracting muscle fibres. Nature. 1982 Dec 23;300(5894):776–778. doi: 10.1038/300776a0. [DOI] [PubMed] [Google Scholar]
- Crowder M. S., Cooke R. Orientation of spin-labeled nucleotides bound to myosin in glycerinated muscle fibers. Biophys J. 1987 Feb;51(2):323–333. doi: 10.1016/S0006-3495(87)83338-6. [DOI] [PMC free article] [PubMed] [Google Scholar]
- Crowder M. S., Cooke R. The effect of myosin sulphydryl modification on the mechanics of fibre contraction. J Muscle Res Cell Motil. 1984 Apr;5(2):131–146. doi: 10.1007/BF00712152. [DOI] [PubMed] [Google Scholar]
- Curmi P. M., Stone D. B., Schneider D. K., Spudich J. A., Mendelson R. A. Comparison of the structure of myosin subfragment 1 bound to actin and free in solution. A neutron scattering study using actin made "invisible" by deuteration. J Mol Biol. 1988 Oct 5;203(3):781–798. doi: 10.1016/0022-2836(88)90209-4. [DOI] [PubMed] [Google Scholar]
- Dos Remedios C. G., Millikan R. G., Morales M. F. Polarization of tryptophan fluorescence from single striated muscle fibers. A molecular probe of contractile state. J Gen Physiol. 1972 Jan;59(1):103–120. doi: 10.1085/jgp.59.1.103. [DOI] [PMC free article] [PubMed] [Google Scholar]
- Horowits R., Kempner E. S., Bisher M. E., Podolsky R. J. A physiological role for titin and nebulin in skeletal muscle. Nature. 1986 Sep 11;323(6084):160–164. doi: 10.1038/323160a0. [DOI] [PubMed] [Google Scholar]
- Horowits R., Podolsky R. J. The positional stability of thick filaments in activated skeletal muscle depends on sarcomere length: evidence for the role of titin filaments. J Cell Biol. 1987 Nov;105(5):2217–2223. doi: 10.1083/jcb.105.5.2217. [DOI] [PMC free article] [PubMed] [Google Scholar]
- Huxley A. F., Simmons R. M. Proposed mechanism of force generation in striated muscle. Nature. 1971 Oct 22;233(5321):533–538. doi: 10.1038/233533a0. [DOI] [PubMed] [Google Scholar]
- Huxley H. E., Kress M. Crossbridge behaviour during muscle contraction. J Muscle Res Cell Motil. 1985 Apr;6(2):153–161. doi: 10.1007/BF00713057. [DOI] [PubMed] [Google Scholar]
- Huxley H. E. The mechanism of muscular contraction. Science. 1969 Jun 20;164(3886):1356–1365. doi: 10.1126/science.164.3886.1356. [DOI] [PubMed] [Google Scholar]
- Laemmli U. K. Cleavage of structural proteins during the assembly of the head of bacteriophage T4. Nature. 1970 Aug 15;227(5259):680–685. doi: 10.1038/227680a0. [DOI] [PubMed] [Google Scholar]
- Naylor G. R., Podolsky R. J. X-ray diffraction of strained muscle fibers in rigor. Proc Natl Acad Sci U S A. 1981 Sep;78(9):5559–5563. doi: 10.1073/pnas.78.9.5559. [DOI] [PMC free article] [PubMed] [Google Scholar]
- Weeds A. G., Taylor R. S. Separation of subfragment-1 isoenzymes from rabbit skeletal muscle myosin. Nature. 1975 Sep 4;257(5521):54–56. doi: 10.1038/257054a0. [DOI] [PubMed] [Google Scholar]
- Yanagida T. Angle of active site of myosin heads in contracting muscle during sudden length changes. J Muscle Res Cell Motil. 1985 Feb;6(1):43–52. doi: 10.1007/BF00712310. [DOI] [PubMed] [Google Scholar]
- Yanagida T. Angles of nucleotides bound to cross-bridges in glycerinated muscle fiber at various concentrations of epsilon-ATP, epsilon-ADP and epsilon-AMPPNP detected by polarized fluorescence. J Mol Biol. 1981 Mar 15;146(4):539–560. doi: 10.1016/0022-2836(81)90046-2. [DOI] [PubMed] [Google Scholar]