Abstract
By optically probing with a focused, low-power laser beam, we measured the spontaneous deflection fluctuations of the sensory hair bundles on frog saccular hair cells with a sensitivity of about 1 pm/square root of Hz. The preparation was illuminated by two orthogonally polarized laser beams separated by only about 0.2 microns at their foci in the structure under investigation. Slight movement of the object from one beam toward the other caused a change of the phase difference between the transmitted beams and an intensity modulation at the detector where the beams interfered. Maintenance of the health of the cells and function of the transduction mechanism were occasionally confirmed by measuring the intracellular resting potential and the sensitivity of transduction. The root-mean-square (rms) displacement of approximately 3.5 nm at a hair bundle's tip suggests a stiffness of about 350 microN/m, in agreement with measurements made with a probe attached to a bundle's tip. The spectra resemble those of overdamped harmonic oscillators with roll-off frequencies between 200 and 800 Hz. Because the roll-off frequencies depended strongly on the viscosity of the bathing medium, we conclude that hair-bundle motion is mainly damped by the surrounding fluid.
Full text
PDF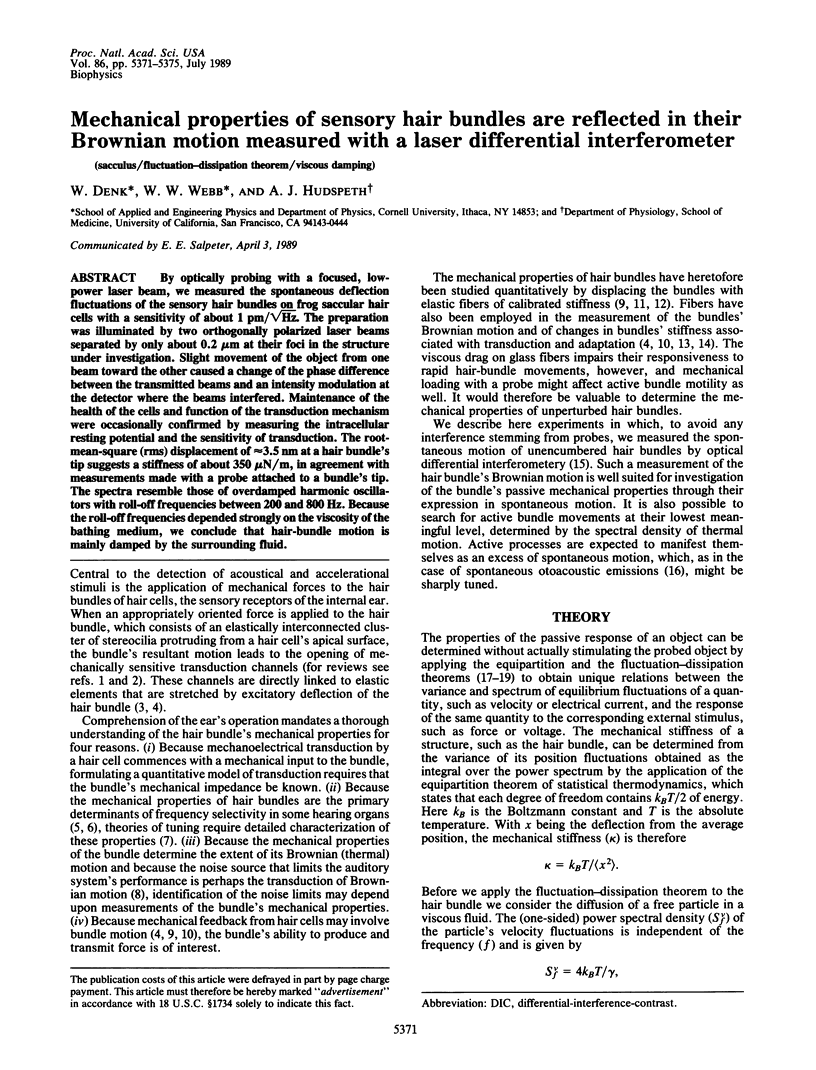
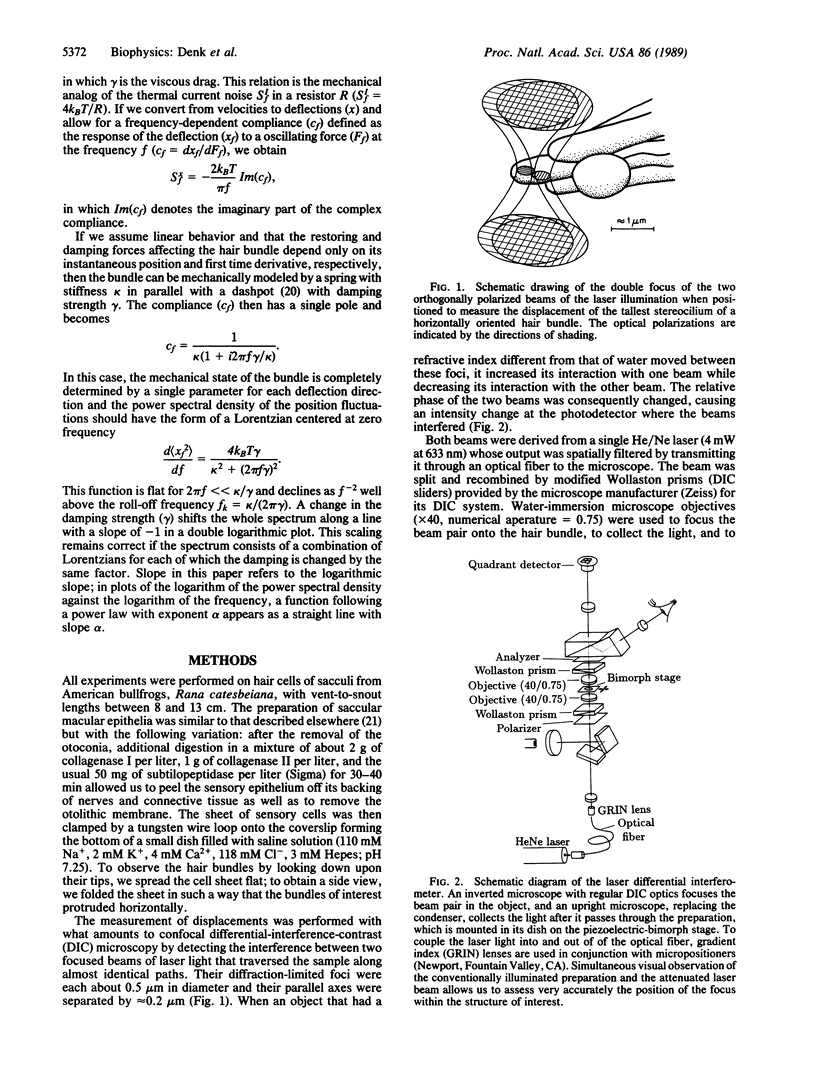
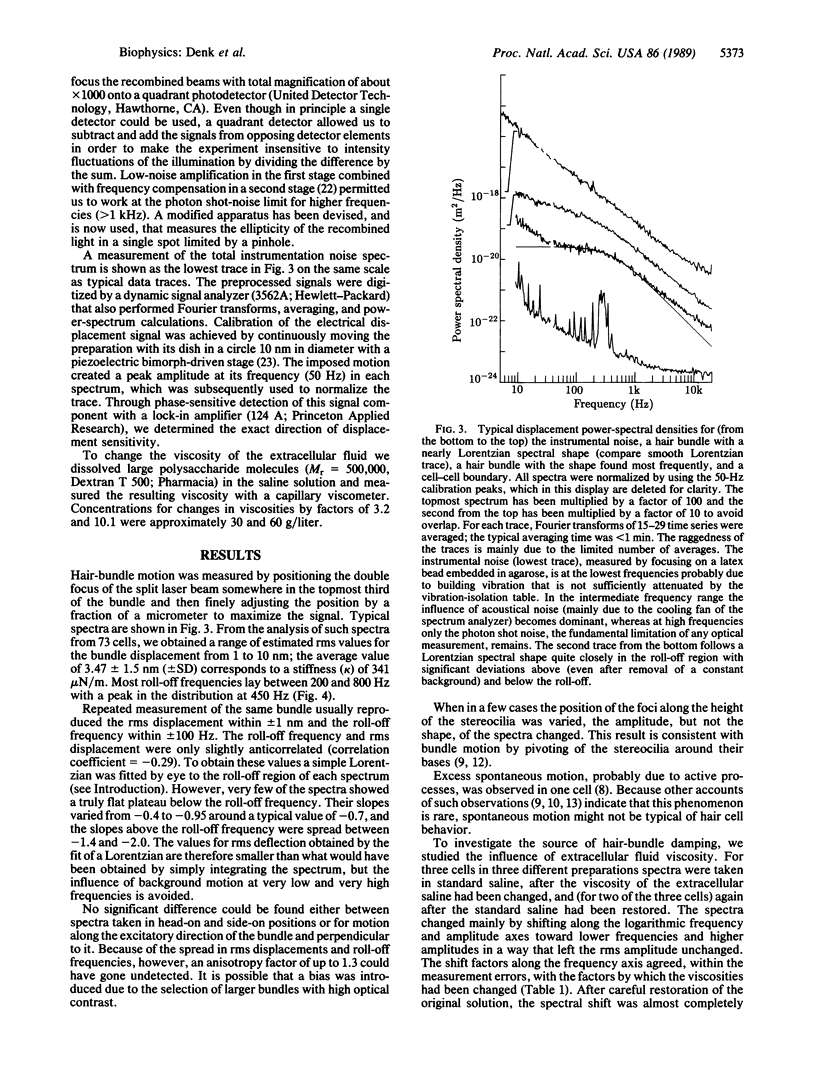
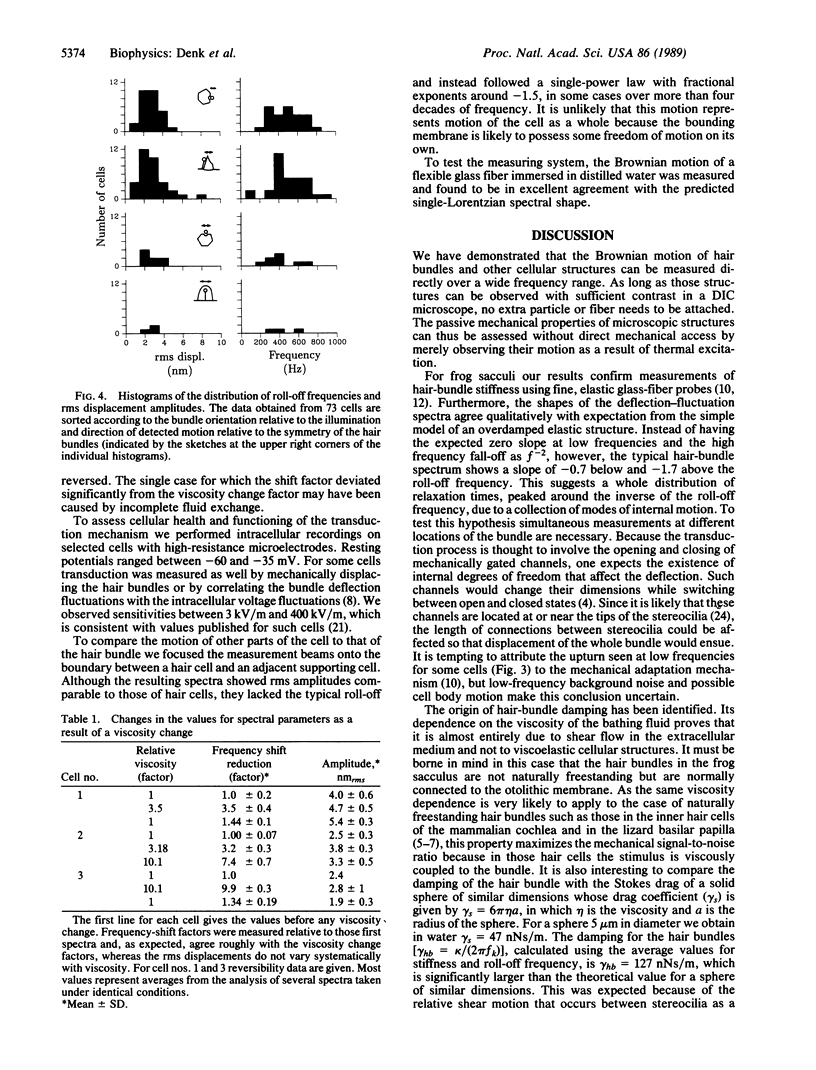
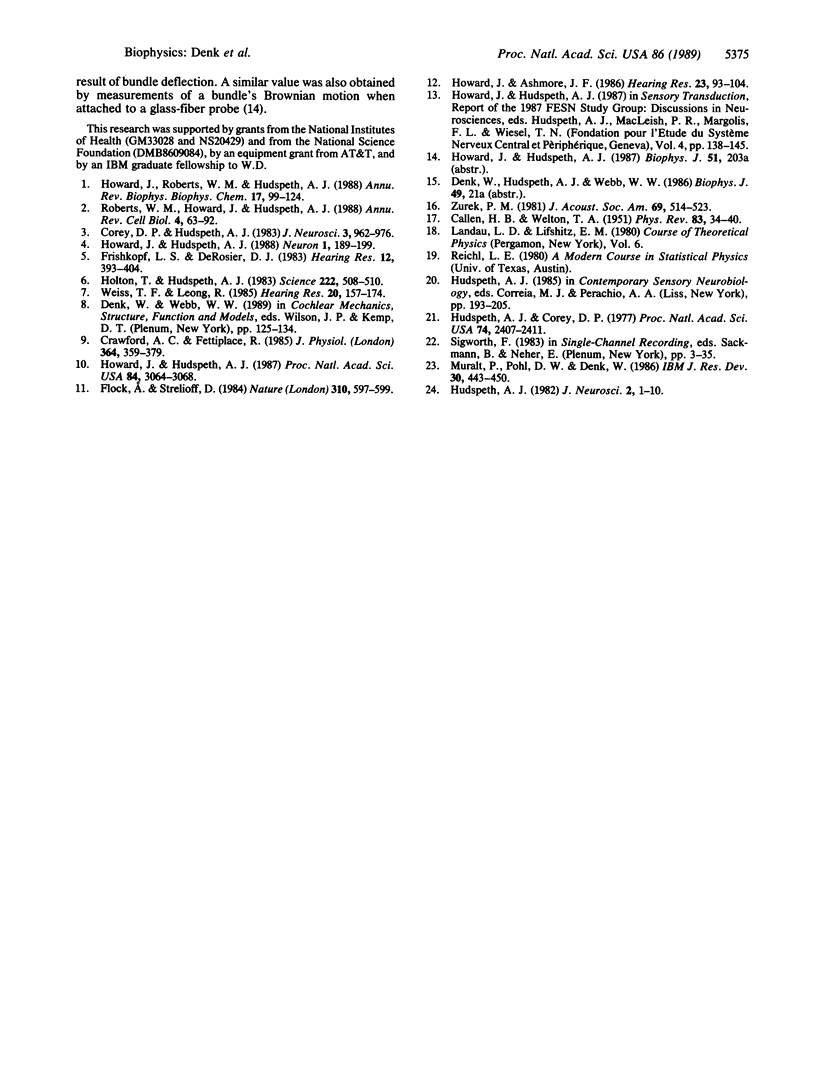
Selected References
These references are in PubMed. This may not be the complete list of references from this article.
- Corey D. P., Hudspeth A. J. Kinetics of the receptor current in bullfrog saccular hair cells. J Neurosci. 1983 May;3(5):962–976. doi: 10.1523/JNEUROSCI.03-05-00962.1983. [DOI] [PMC free article] [PubMed] [Google Scholar]
- Crawford A. C., Fettiplace R. The mechanical properties of ciliary bundles of turtle cochlear hair cells. J Physiol. 1985 Jul;364:359–379. doi: 10.1113/jphysiol.1985.sp015750. [DOI] [PMC free article] [PubMed] [Google Scholar]
- Flock A., Strelioff D. Graded and nonlinear mechanical properties of sensory hairs in the mammalian hearing organ. Nature. 1984 Aug 16;310(5978):597–599. doi: 10.1038/310597a0. [DOI] [PubMed] [Google Scholar]
- Frishkopf L. S., DeRosier D. J. Mechanical tuning of free-standing stereociliary bundles and frequency analysis in the alligator lizard cochlea. Hear Res. 1983 Dec;12(3):393–404. doi: 10.1016/0378-5955(83)90008-4. [DOI] [PubMed] [Google Scholar]
- Holton T., Hudspeth A. J. A micromechanical contribution to cochlear tuning and tonotopic organization. Science. 1983 Nov 4;222(4623):508–510. doi: 10.1126/science.6623089. [DOI] [PubMed] [Google Scholar]
- Howard J., Ashmore J. F. Stiffness of sensory hair bundles in the sacculus of the frog. Hear Res. 1986;23(1):93–104. doi: 10.1016/0378-5955(86)90178-4. [DOI] [PubMed] [Google Scholar]
- Howard J., Hudspeth A. J. Compliance of the hair bundle associated with gating of mechanoelectrical transduction channels in the bullfrog's saccular hair cell. Neuron. 1988 May;1(3):189–199. doi: 10.1016/0896-6273(88)90139-0. [DOI] [PubMed] [Google Scholar]
- Howard J., Hudspeth A. J. Mechanical relaxation of the hair bundle mediates adaptation in mechanoelectrical transduction by the bullfrog's saccular hair cell. Proc Natl Acad Sci U S A. 1987 May;84(9):3064–3068. doi: 10.1073/pnas.84.9.3064. [DOI] [PMC free article] [PubMed] [Google Scholar]
- Howard J., Roberts W. M., Hudspeth A. J. Mechanoelectrical transduction by hair cells. Annu Rev Biophys Biophys Chem. 1988;17:99–124. doi: 10.1146/annurev.bb.17.060188.000531. [DOI] [PubMed] [Google Scholar]
- Hudspeth A. J., Corey D. P. Sensitivity, polarity, and conductance change in the response of vertebrate hair cells to controlled mechanical stimuli. Proc Natl Acad Sci U S A. 1977 Jun;74(6):2407–2411. doi: 10.1073/pnas.74.6.2407. [DOI] [PMC free article] [PubMed] [Google Scholar]
- Hudspeth A. J. Extracellular current flow and the site of transduction by vertebrate hair cells. J Neurosci. 1982 Jan;2(1):1–10. doi: 10.1523/JNEUROSCI.02-01-00001.1982. [DOI] [PMC free article] [PubMed] [Google Scholar]
- Roberts W. M., Howard J., Hudspeth A. J. Hair cells: transduction, tuning, and transmission in the inner ear. Annu Rev Cell Biol. 1988;4:63–92. doi: 10.1146/annurev.cb.04.110188.000431. [DOI] [PubMed] [Google Scholar]
- Weiss T. F., Leong R. A model for signal transmission in an ear having hair cells with free-standing stereocilia. III. Micromechanical stage. Hear Res. 1985;20(2):157–174. doi: 10.1016/0378-5955(85)90166-2. [DOI] [PubMed] [Google Scholar]
- Zurek P. M. Spontaneous narrowband acoustic signals emitted by human ears. J Acoust Soc Am. 1981 Feb;69(2):514–523. doi: 10.1121/1.385481. [DOI] [PubMed] [Google Scholar]