Abstract
Myosin was recently identified in erythrocytes and was shown to partition both with membrane and cytosolic fractions, suggesting that it may be loosely bound to membranes [Fowler, V. M., Davis, J. Q. & Bennett, V. (1985) J. Cell Biol. 100, 47-55, and Wong, A. J., Kiehart, D. P. & Pollard, T. D. (1985) J. Biol. Chem. 260, 46-49]; however, the molecular basis for this binding was unclear. The present studies employed immobilized monomeric myosin to examine the interaction of myosin with erythrocyte protein 4.1. In human erythrocytes, protein 4.1 binds to integral membrane proteins and mediates spectrin-actin assembly. Protein 4.1 binds to rabbit skeletal muscle myosin with a Kd = 140 nM and a stoichiometry consistent with 1:1 binding. Heavy meromyosin competes for protein 4.1 binding with Ki = 36-54 nM; however, the S1 fragment (the myosin head) competes less efficiently. Affinity chromatography of partial chymotryptic digests of protein 4.1 on immobilized myosin identified a 10-kDa domain of protein 4.1 as the myosin-binding site. In functional studies, protein 4.1 partially inhibited the actin-activated Mg2+-ATPase activity of rabbit skeletal muscle myosin with Ki = 51 nM. Liver cytosolic and erythrocyte myosins preactivated with myosin light-chain kinase were similarly inhibited by protein 4.1. These studies show that protein 4.1 binds, modulates, and thus may regulate myosin. This interaction might serve to generate the contractile forces involved in Mg2+-ATP-dependent shape changes in erythrocytes and may additionally serve as a model for myosin organization and regulation in non-muscle cells.
Full text
PDF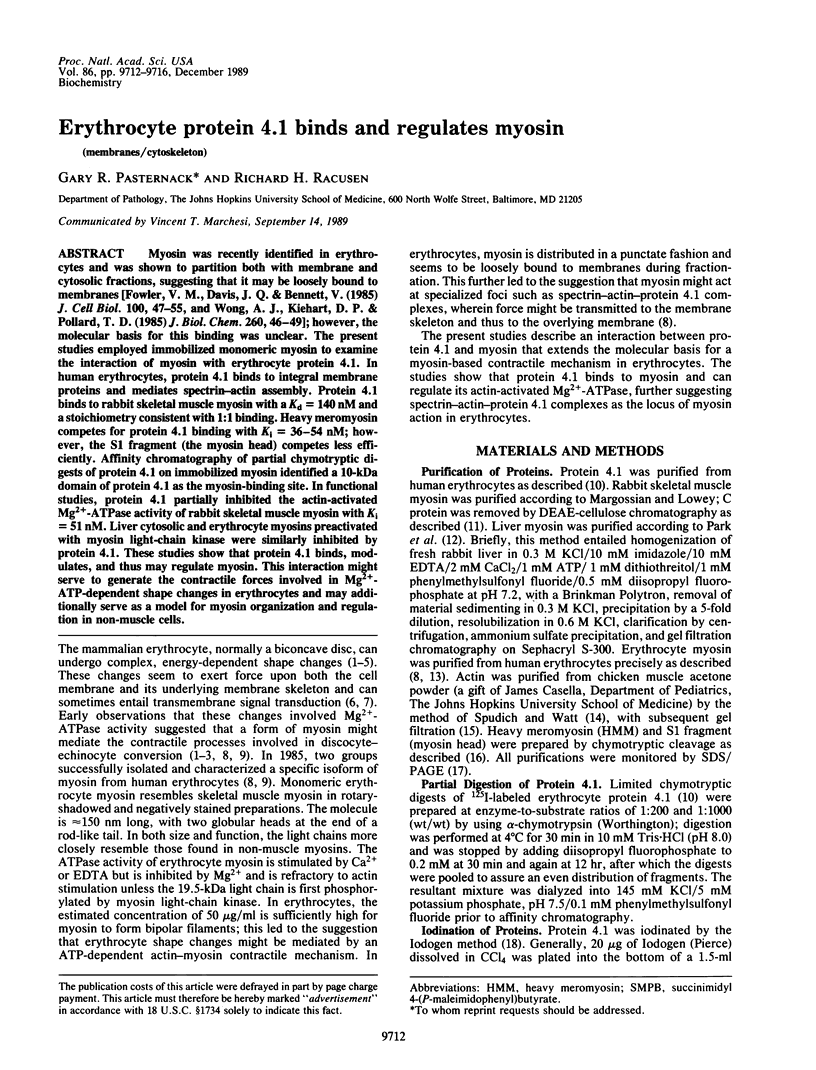
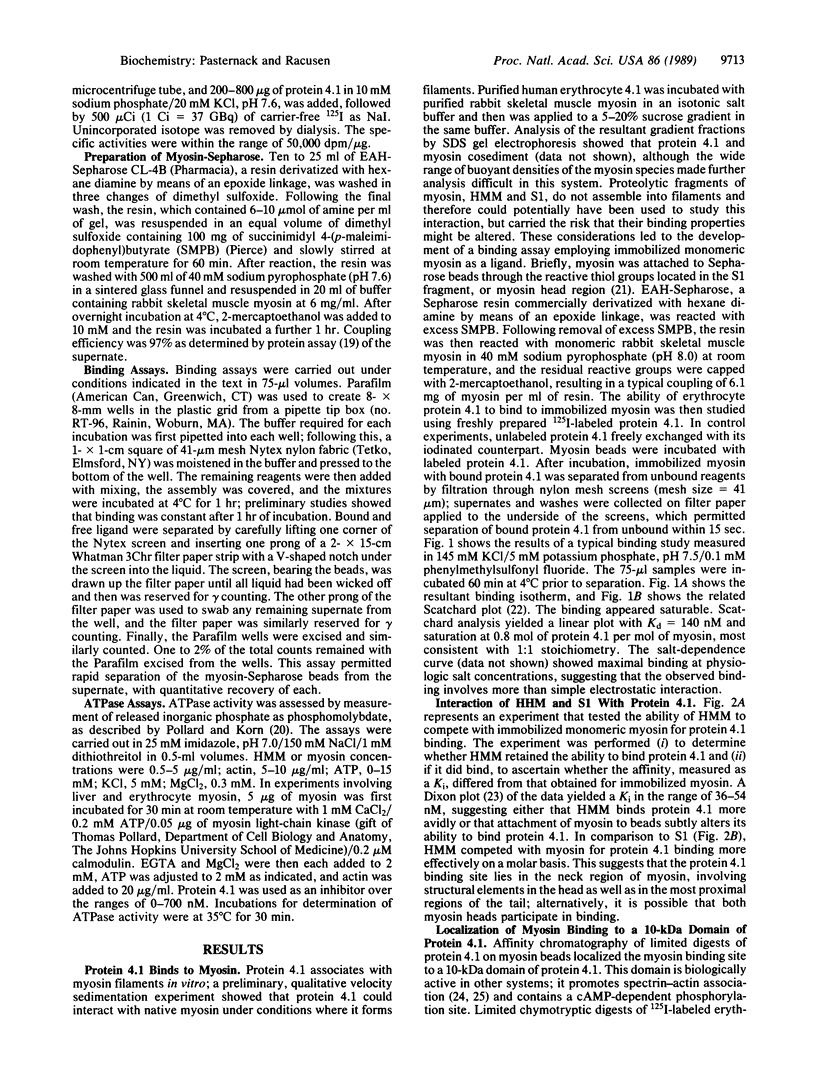
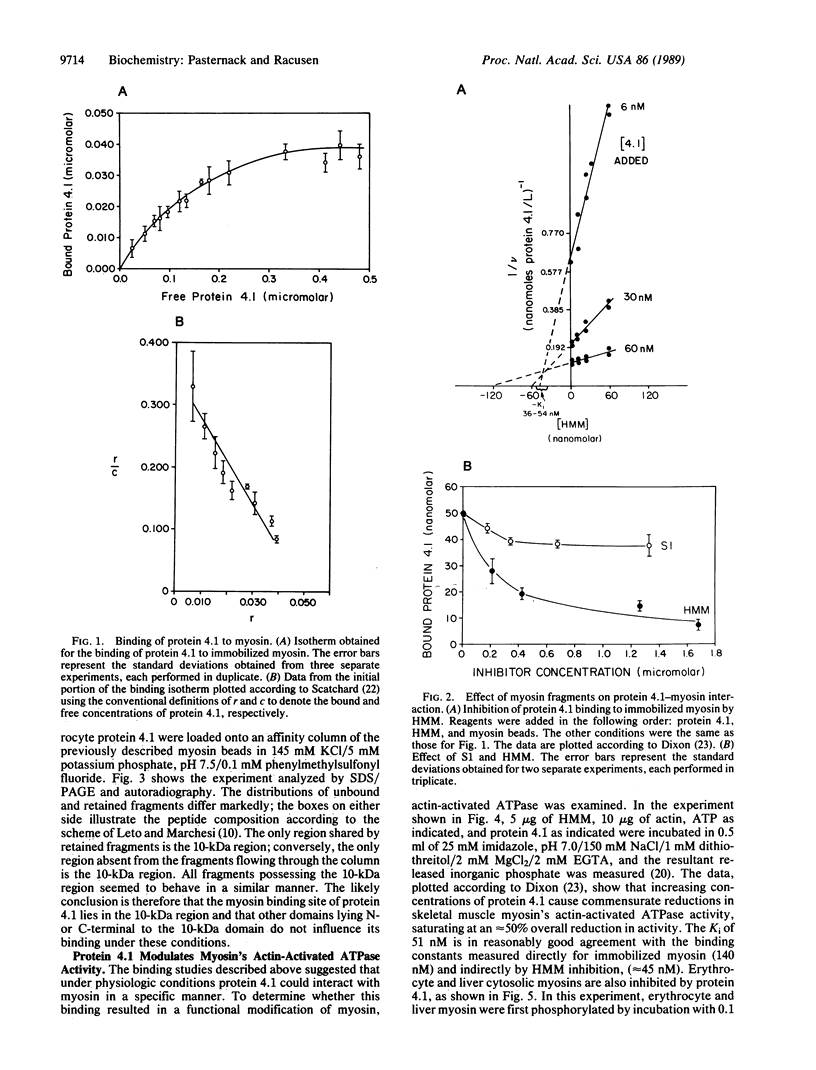
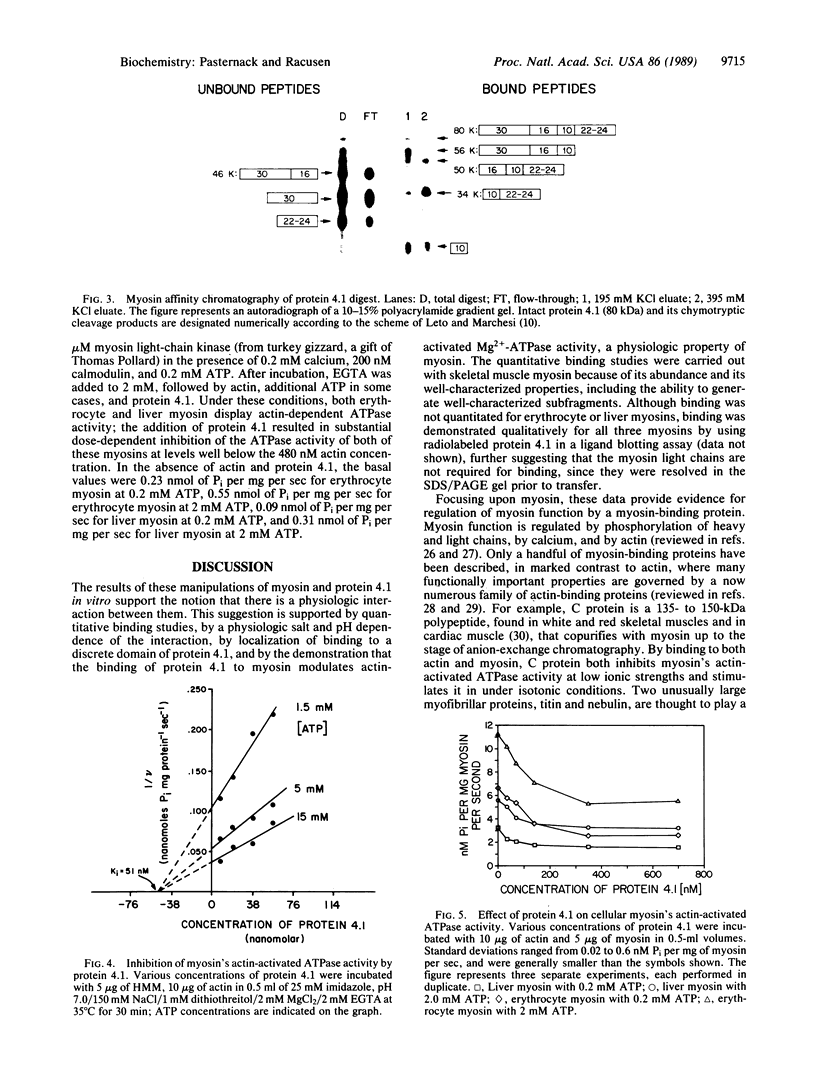
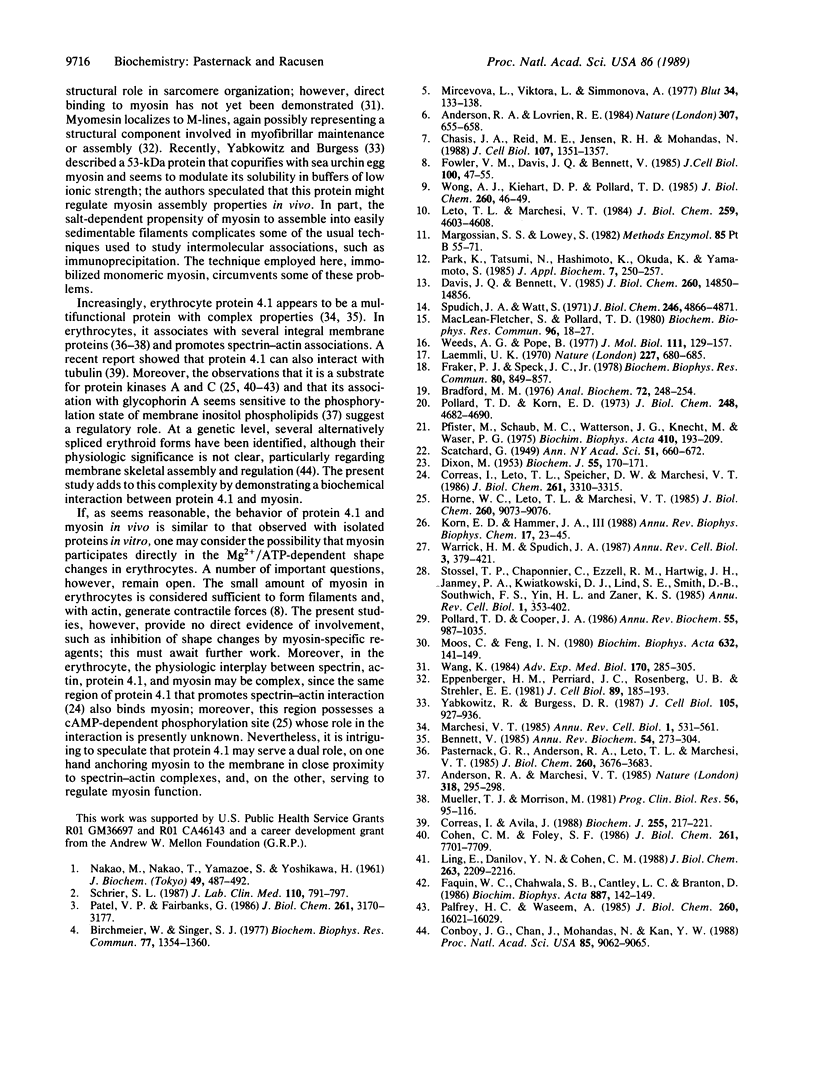
Images in this article
Selected References
These references are in PubMed. This may not be the complete list of references from this article.
- Anderson R. A., Lovrien R. E. Glycophorin is linked by band 4.1 protein to the human erythrocyte membrane skeleton. Nature. 1984 Feb 16;307(5952):655–658. doi: 10.1038/307655a0. [DOI] [PubMed] [Google Scholar]
- Anderson R. A., Marchesi V. T. Regulation of the association of membrane skeletal protein 4.1 with glycophorin by a polyphosphoinositide. Nature. 1985 Nov 21;318(6043):295–298. doi: 10.1038/318295a0. [DOI] [PubMed] [Google Scholar]
- Bennett V. The membrane skeleton of human erythrocytes and its implications for more complex cells. Annu Rev Biochem. 1985;54:273–304. doi: 10.1146/annurev.bi.54.070185.001421. [DOI] [PubMed] [Google Scholar]
- Birchmeier W., Singer S. J. Muscle G-actin is an inhibitor of ATP-induced erythrocyte ghost shape changes and endocytosis. Biochem Biophys Res Commun. 1977 Aug 22;77(4):1354–1360. doi: 10.1016/s0006-291x(77)80128-9. [DOI] [PubMed] [Google Scholar]
- Bradford M. M. A rapid and sensitive method for the quantitation of microgram quantities of protein utilizing the principle of protein-dye binding. Anal Biochem. 1976 May 7;72:248–254. doi: 10.1006/abio.1976.9999. [DOI] [PubMed] [Google Scholar]
- Chasis J. A., Reid M. E., Jensen R. H., Mohandas N. Signal transduction by glycophorin A: role of extracellular and cytoplasmic domains in a modulatable process. J Cell Biol. 1988 Oct;107(4):1351–1357. doi: 10.1083/jcb.107.4.1351. [DOI] [PMC free article] [PubMed] [Google Scholar]
- Cohen C. M., Foley S. F. Phorbol ester- and Ca2+-dependent phosphorylation of human red cell membrane skeletal proteins. J Biol Chem. 1986 Jun 15;261(17):7701–7709. [PubMed] [Google Scholar]
- Conboy J. G., Chan J., Mohandas N., Kan Y. W. Multiple protein 4.1 isoforms produced by alternative splicing in human erythroid cells. Proc Natl Acad Sci U S A. 1988 Dec;85(23):9062–9065. doi: 10.1073/pnas.85.23.9062. [DOI] [PMC free article] [PubMed] [Google Scholar]
- Correas I., Avila J. Erythrocyte protein 4.1 associates with tubulin. Biochem J. 1988 Oct 1;255(1):217–221. [PMC free article] [PubMed] [Google Scholar]
- Correas I., Leto T. L., Speicher D. W., Marchesi V. T. Identification of the functional site of erythrocyte protein 4.1 involved in spectrin-actin associations. J Biol Chem. 1986 Mar 5;261(7):3310–3315. [PubMed] [Google Scholar]
- DIXON M. The determination of enzyme inhibitor constants. Biochem J. 1953 Aug;55(1):170–171. doi: 10.1042/bj0550170. [DOI] [PMC free article] [PubMed] [Google Scholar]
- Davis J. Q., Bennett V. Human erythrocyte clathrin and clathrin-uncoating protein. J Biol Chem. 1985 Nov 25;260(27):14850–14856. [PubMed] [Google Scholar]
- Eppenberger H. M., Perriard J. C., Rosenberg U. B., Strehler E. E. The Mr 165,000 M-protein myomesin: a specific protein of cross-striated muscle cells. J Cell Biol. 1981 May;89(2):185–193. doi: 10.1083/jcb.89.2.185. [DOI] [PMC free article] [PubMed] [Google Scholar]
- Faquin W. C., Chahwala S. B., Cantley L. C., Branton D. Protein kinase C of human erythrocytes phosphorylates bands 4.1 and 4.9. Biochim Biophys Acta. 1986 Jul 11;887(2):142–149. doi: 10.1016/0167-4889(86)90048-0. [DOI] [PubMed] [Google Scholar]
- Fowler V. M., Davis J. Q., Bennett V. Human erythrocyte myosin: identification and purification. J Cell Biol. 1985 Jan;100(1):47–55. doi: 10.1083/jcb.100.1.47. [DOI] [PMC free article] [PubMed] [Google Scholar]
- Fraker P. J., Speck J. C., Jr Protein and cell membrane iodinations with a sparingly soluble chloroamide, 1,3,4,6-tetrachloro-3a,6a-diphrenylglycoluril. Biochem Biophys Res Commun. 1978 Feb 28;80(4):849–857. doi: 10.1016/0006-291x(78)91322-0. [DOI] [PubMed] [Google Scholar]
- Horne W. C., Leto T. L., Marchesi V. T. Differential phosphorylation of multiple sites in protein 4.1 and protein 4.9 by phorbol ester-activated and cyclic AMP-dependent protein kinases. J Biol Chem. 1985 Aug 5;260(16):9073–9076. [PubMed] [Google Scholar]
- Korn E. D., Hammer J. A., 3rd Myosins of nonmuscle cells. Annu Rev Biophys Biophys Chem. 1988;17:23–45. doi: 10.1146/annurev.bb.17.060188.000323. [DOI] [PubMed] [Google Scholar]
- Laemmli U. K. Cleavage of structural proteins during the assembly of the head of bacteriophage T4. Nature. 1970 Aug 15;227(5259):680–685. doi: 10.1038/227680a0. [DOI] [PubMed] [Google Scholar]
- Leto T. L., Marchesi V. T. A structural model of human erythrocyte protein 4.1. J Biol Chem. 1984 Apr 10;259(7):4603–4608. [PubMed] [Google Scholar]
- Ling E., Danilov Y. N., Cohen C. M. Modulation of red cell band 4.1 function by cAMP-dependent kinase and protein kinase C phosphorylation. J Biol Chem. 1988 Feb 15;263(5):2209–2216. [PubMed] [Google Scholar]
- MacLean-Fletcher S., Pollard T. D. Identification of a factor in conventional muscle actin preparations which inhibits actin filament self-association. Biochem Biophys Res Commun. 1980 Sep 16;96(1):18–27. doi: 10.1016/0006-291x(80)91175-4. [DOI] [PubMed] [Google Scholar]
- Marchesi V. T. Stabilizing infrastructure of cell membranes. Annu Rev Cell Biol. 1985;1:531–561. doi: 10.1146/annurev.cb.01.110185.002531. [DOI] [PubMed] [Google Scholar]
- Margossian S. S., Lowey S. Preparation of myosin and its subfragments from rabbit skeletal muscle. Methods Enzymol. 1982;85(Pt B):55–71. doi: 10.1016/0076-6879(82)85009-x. [DOI] [PubMed] [Google Scholar]
- Mircevová L., Viktora L., Simmonová A. The change of erythrocyte shape following action of different substances altering mg++-dependent ATPase activity (actomyosin-like protein). Blut. 1977 Feb;34(2):133–138. doi: 10.1007/BF00999859. [DOI] [PubMed] [Google Scholar]
- Moos C., Feng I. N. Effect of C-protein on actomyosin ATPase. Biochim Biophys Acta. 1980 Oct 1;632(2):141–149. doi: 10.1016/0304-4165(80)90071-9. [DOI] [PubMed] [Google Scholar]
- Mueller T. J., Morrison M. Glycoconnectin (PAS 2), a membrane attachment site for the human erythrocyte cytoskeleton. Prog Clin Biol Res. 1981;56:95–116. [PubMed] [Google Scholar]
- NAKAO M., NAKAO T., YAMAZOE S., YOSHIKAWA H. Adenosine triphosphate and shape of erythrocytes. J Biochem. 1961 Jun;49:487–492. doi: 10.1093/oxfordjournals.jbchem.a127333. [DOI] [PubMed] [Google Scholar]
- Palfrey H. C., Waseem A. Protein kinase C in the human erythrocyte. Translocation to the plasma membrane and phosphorylation of bands 4.1 and 4.9 and other membrane proteins. J Biol Chem. 1985 Dec 15;260(29):16021–16029. [PubMed] [Google Scholar]
- Park K., Tatsumi N., Hashimoto K., Okuda K., Yamamoto S. Simplified separation of myosin from rabbit liver. J Appl Biochem. 1985 Aug-Oct;7(4-5):250–257. [PubMed] [Google Scholar]
- Pasternack G. R., Anderson R. A., Leto T. L., Marchesi V. T. Interactions between protein 4.1 and band 3. An alternative binding site for an element of the membrane skeleton. J Biol Chem. 1985 Mar 25;260(6):3676–3683. [PubMed] [Google Scholar]
- Patel V. P., Fairbanks G. Relationship of major phosphorylation reactions and MgATPase activities to ATP-dependent shape change of human erythrocyte membranes. J Biol Chem. 1986 Mar 5;261(7):3170–3177. [PubMed] [Google Scholar]
- Pfister M., Schaub M. C., Watterson J. G., Knecht M., Waser P. G. Radioactive labeling and location of specific thiol groups in myosin from fast, slow and cardiac muscles. Biochim Biophys Acta. 1975 Nov 20;410(1):193–209. doi: 10.1016/0005-2744(75)90220-x. [DOI] [PubMed] [Google Scholar]
- Pollard T. D., Cooper J. A. Actin and actin-binding proteins. A critical evaluation of mechanisms and functions. Annu Rev Biochem. 1986;55:987–1035. doi: 10.1146/annurev.bi.55.070186.005011. [DOI] [PubMed] [Google Scholar]
- Pollard T. D., Korn E. D. Acanthamoeba myosin. I. Isolation from Acanthamoeba castellanii of an enzyme similar to muscle myosin. J Biol Chem. 1973 Jul 10;248(13):4682–4690. [PubMed] [Google Scholar]
- Schrier S. L. Shape changes and deformability in human erythrocyte membranes. J Lab Clin Med. 1987 Dec;110(6):791–797. [PubMed] [Google Scholar]
- Spudich J. A., Watt S. The regulation of rabbit skeletal muscle contraction. I. Biochemical studies of the interaction of the tropomyosin-troponin complex with actin and the proteolytic fragments of myosin. J Biol Chem. 1971 Aug 10;246(15):4866–4871. [PubMed] [Google Scholar]
- Stossel T. P., Chaponnier C., Ezzell R. M., Hartwig J. H., Janmey P. A., Kwiatkowski D. J., Lind S. E., Smith D. B., Southwick F. S., Yin H. L. Nonmuscle actin-binding proteins. Annu Rev Cell Biol. 1985;1:353–402. doi: 10.1146/annurev.cb.01.110185.002033. [DOI] [PubMed] [Google Scholar]
- Wang K. Cytoskeletal matrix in striated muscle: the role of titin, nebulin and intermediate filaments. Adv Exp Med Biol. 1984;170:285–305. doi: 10.1007/978-1-4684-4703-3_25. [DOI] [PubMed] [Google Scholar]
- Warrick H. M., Spudich J. A. Myosin structure and function in cell motility. Annu Rev Cell Biol. 1987;3:379–421. doi: 10.1146/annurev.cb.03.110187.002115. [DOI] [PubMed] [Google Scholar]
- Weeds A. G., Pope B. Studies on the chymotryptic digestion of myosin. Effects of divalent cations on proteolytic susceptibility. J Mol Biol. 1977 Apr;111(2):129–157. doi: 10.1016/s0022-2836(77)80119-8. [DOI] [PubMed] [Google Scholar]
- Wong A. J., Kiehart D. P., Pollard T. D. Myosin from human erythrocytes. J Biol Chem. 1985 Jan 10;260(1):46–49. [PubMed] [Google Scholar]
- Yabkowitz R., Burgess D. R. Low ionic strength solubility of myosin in sea urchin egg extracts is mediated by a myosin-binding protein. J Cell Biol. 1987 Aug;105(2):927–936. doi: 10.1083/jcb.105.2.927. [DOI] [PMC free article] [PubMed] [Google Scholar]