Abstract
After fertilization in Xenopus laevis, inositol 1,4,5-trisphosphate (IP3) mass increased from 53 to 261 fmol/cell and returned to near basal by 10 min after insemination. IP3 was also elevated over control egg levels during first mitosis and first cleavage. Because IP3 levels and the fertilization calcium wave decline at about the same time and because calcium ionophore or pricking the egg increased IP3, the fertilization calcium wave may be due to calcium-induced IP3 production. In addition, the onset of sperm motility was associated with an increase, whereas the acrosomal reaction was accompanied by a decrease in IP3 mass. Combining our published data with this report, the first chronology of the levels of IP3 from the induction of meiosis (maturation) through fertilization and cleavage in one cellular system is summarized. These data suggest an in vivo dose response for IP3 and calcium release. A small (17 fmol/cell) IP3 change during the induction of meiosis may not be associated with a calcium change. Larger IP3 changes at cleavage (40 fmol/cell) and mitosis (125 fmol/cell) are associated with localized small calcium increases, whereas the largest IP3 change (208 fmol/cell) is associated with the large calcium increase at fertilization.
Full text
PDF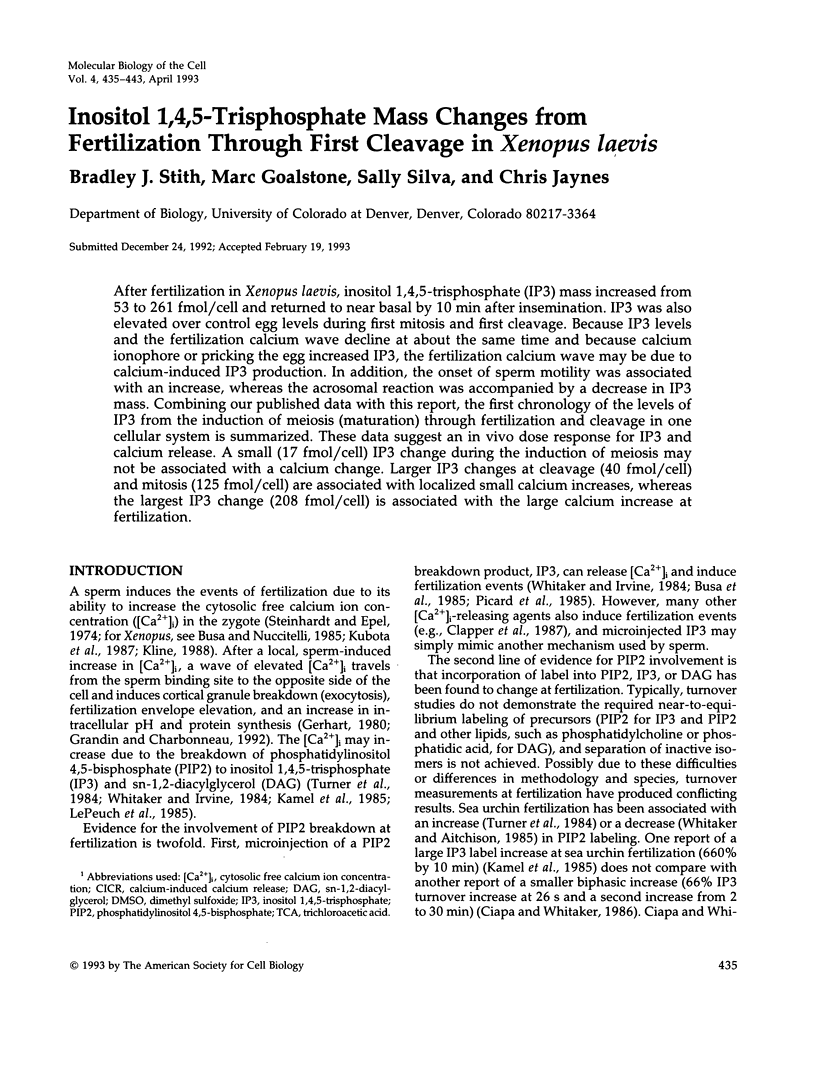
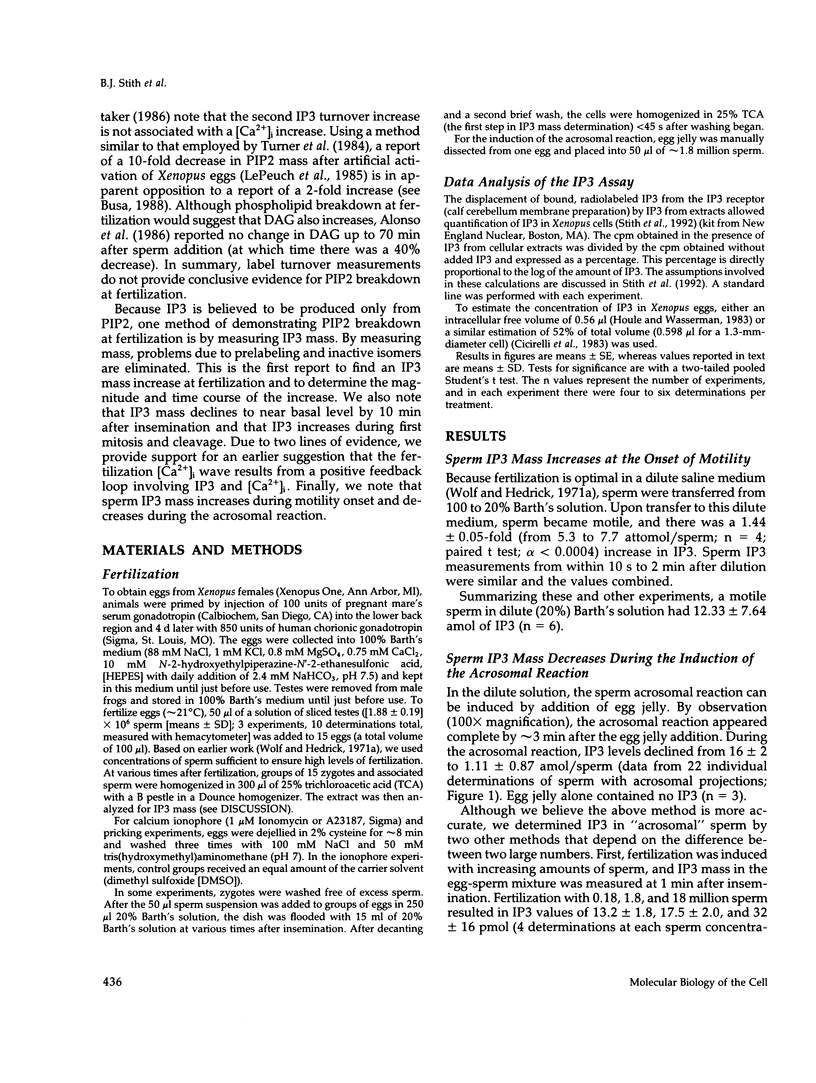
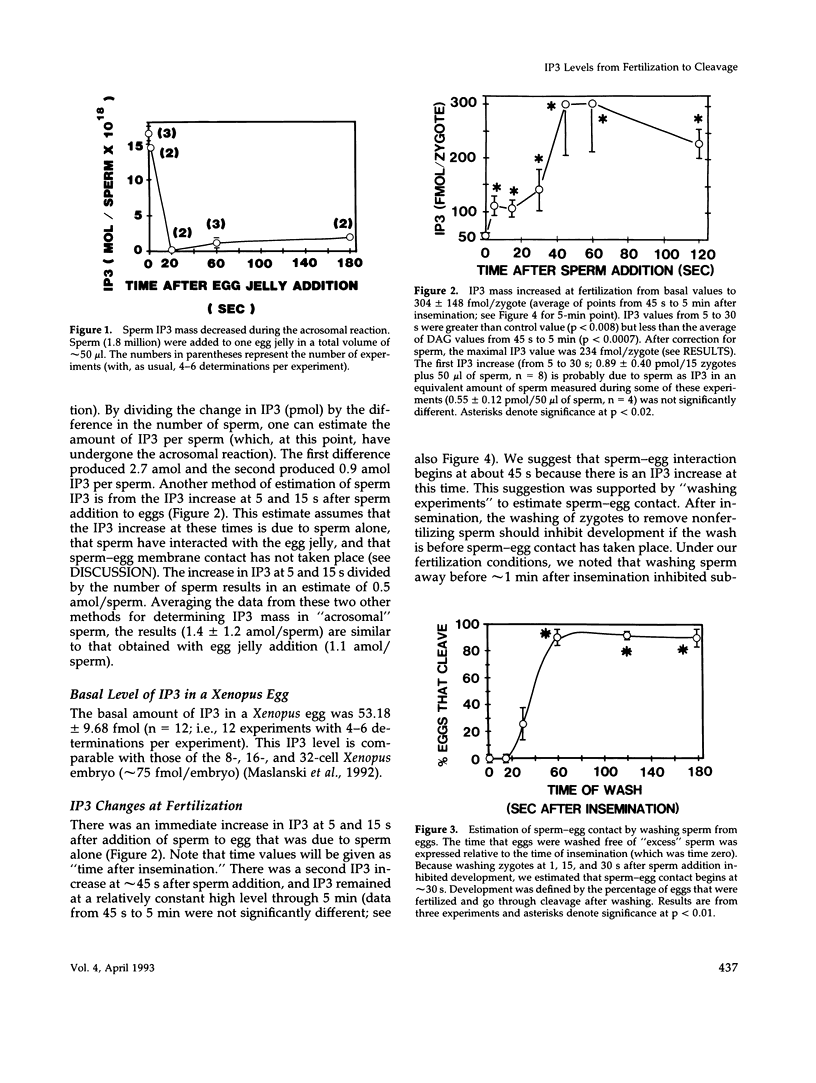
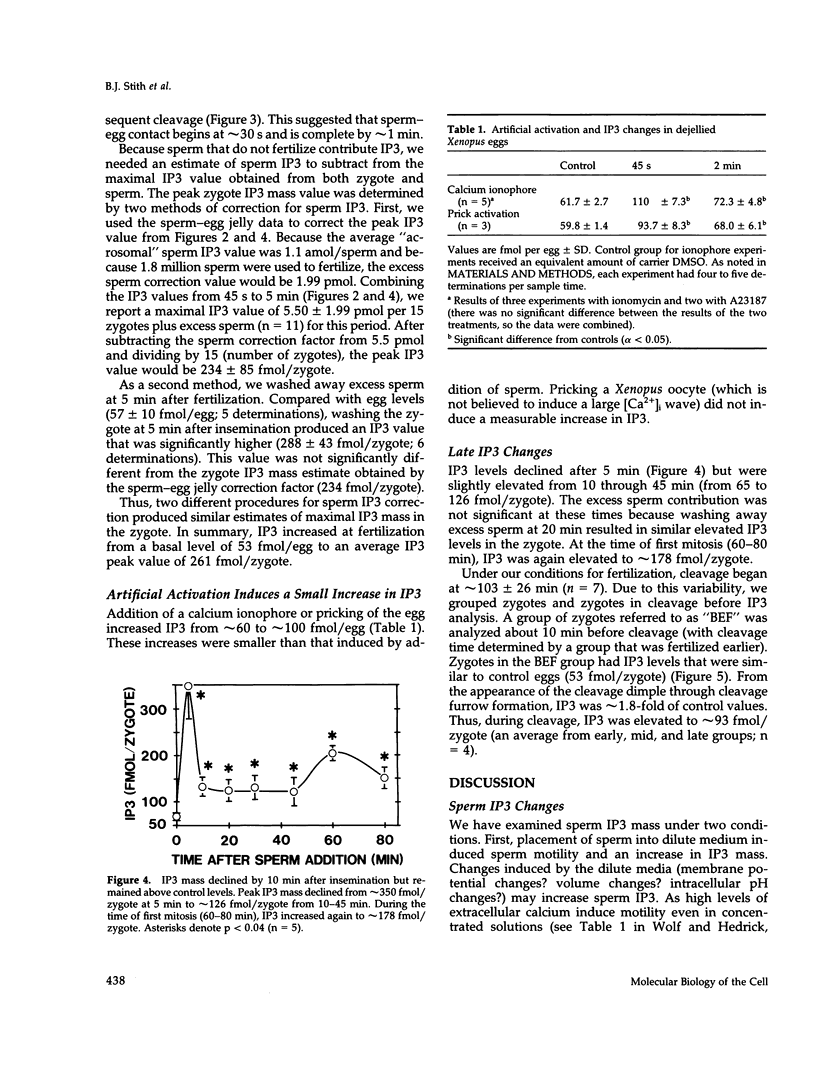
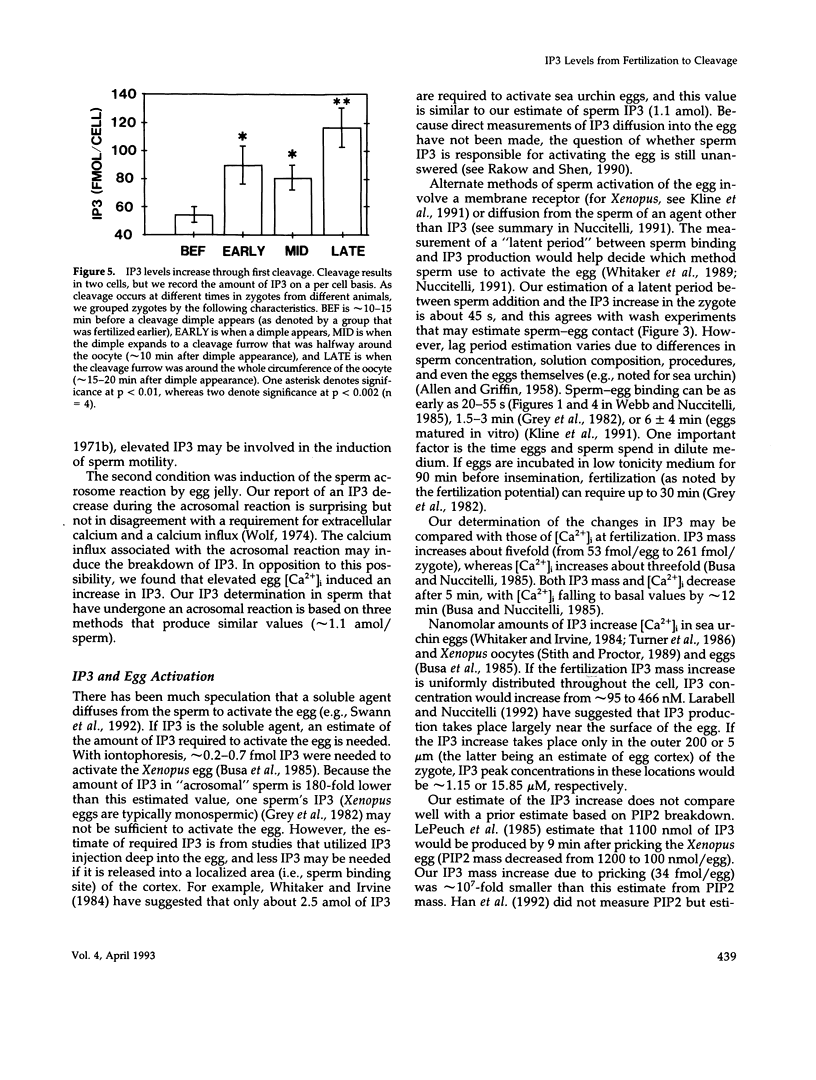
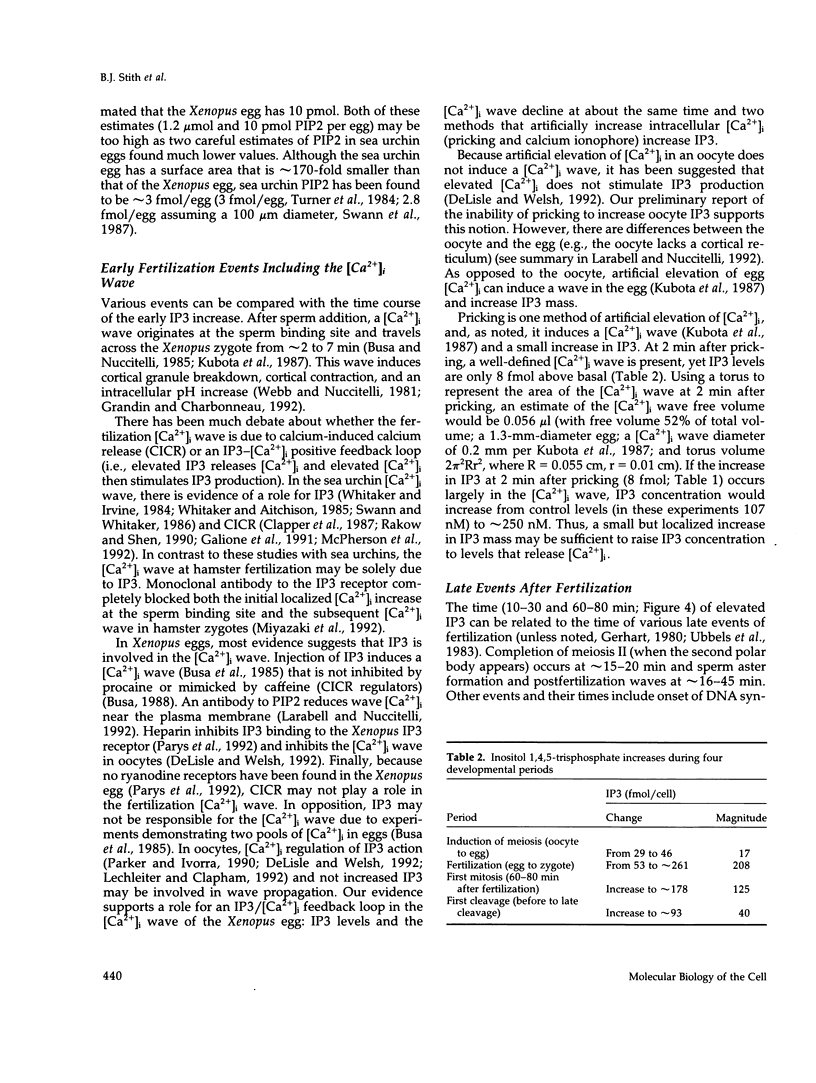
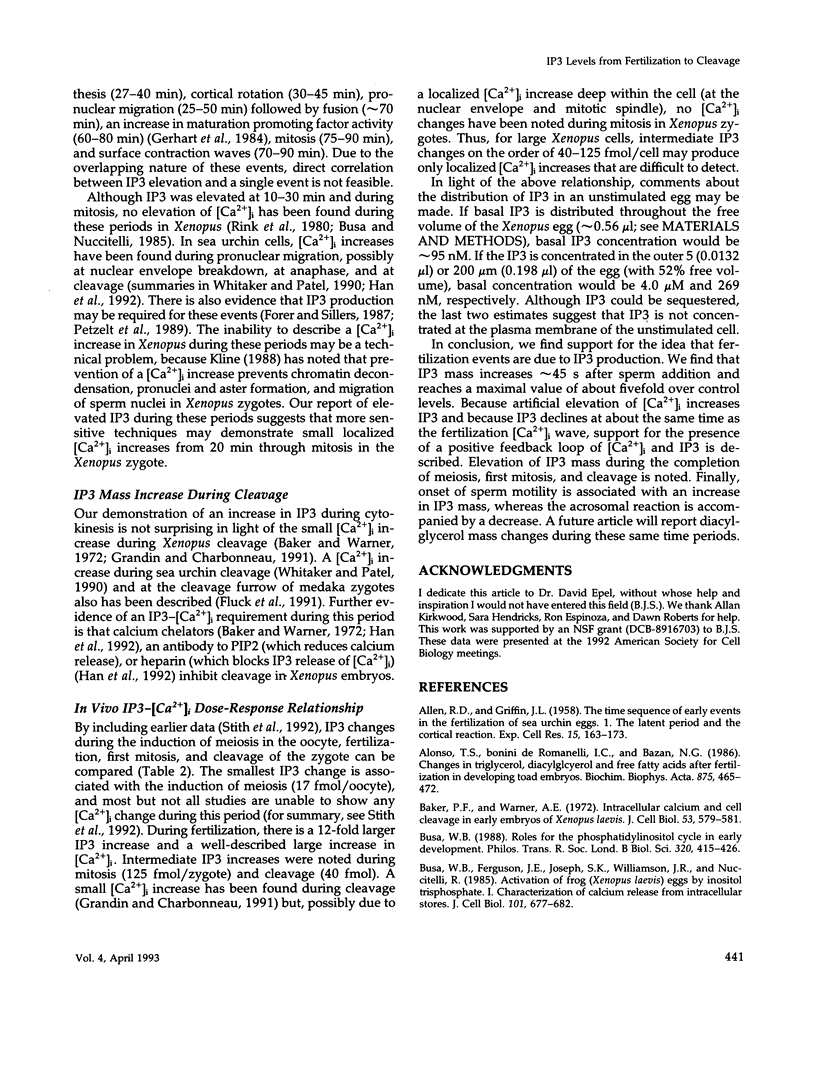
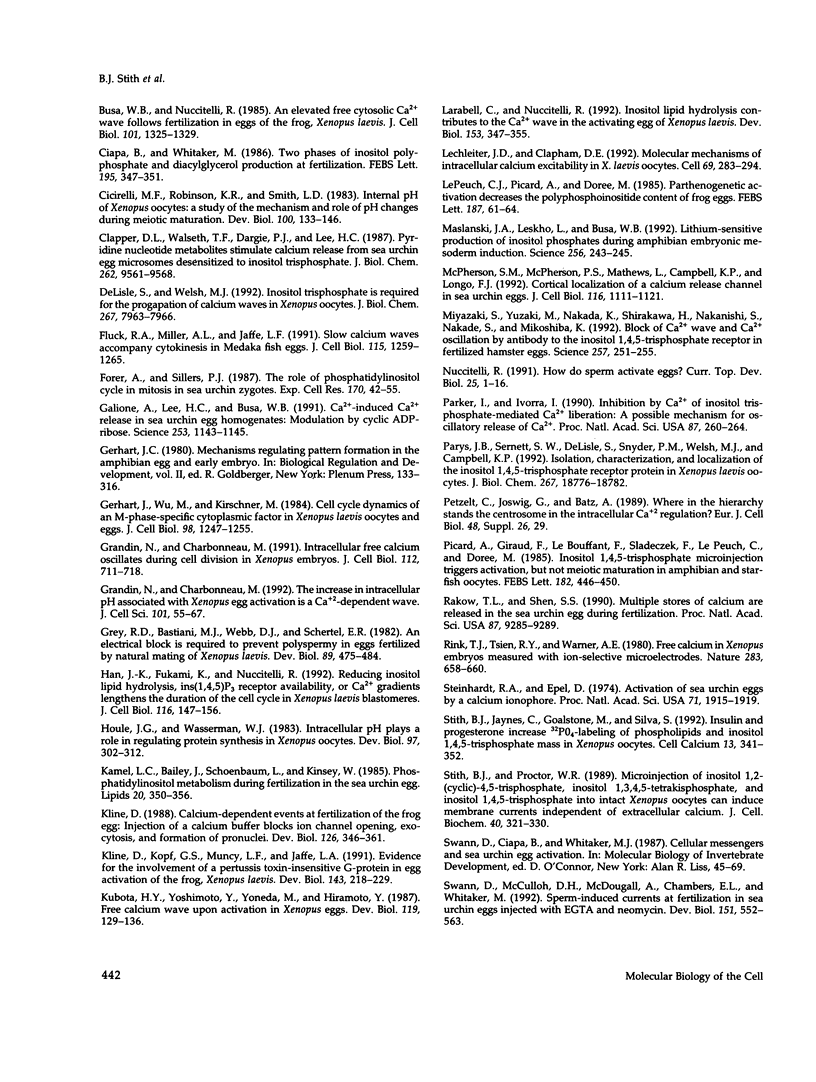
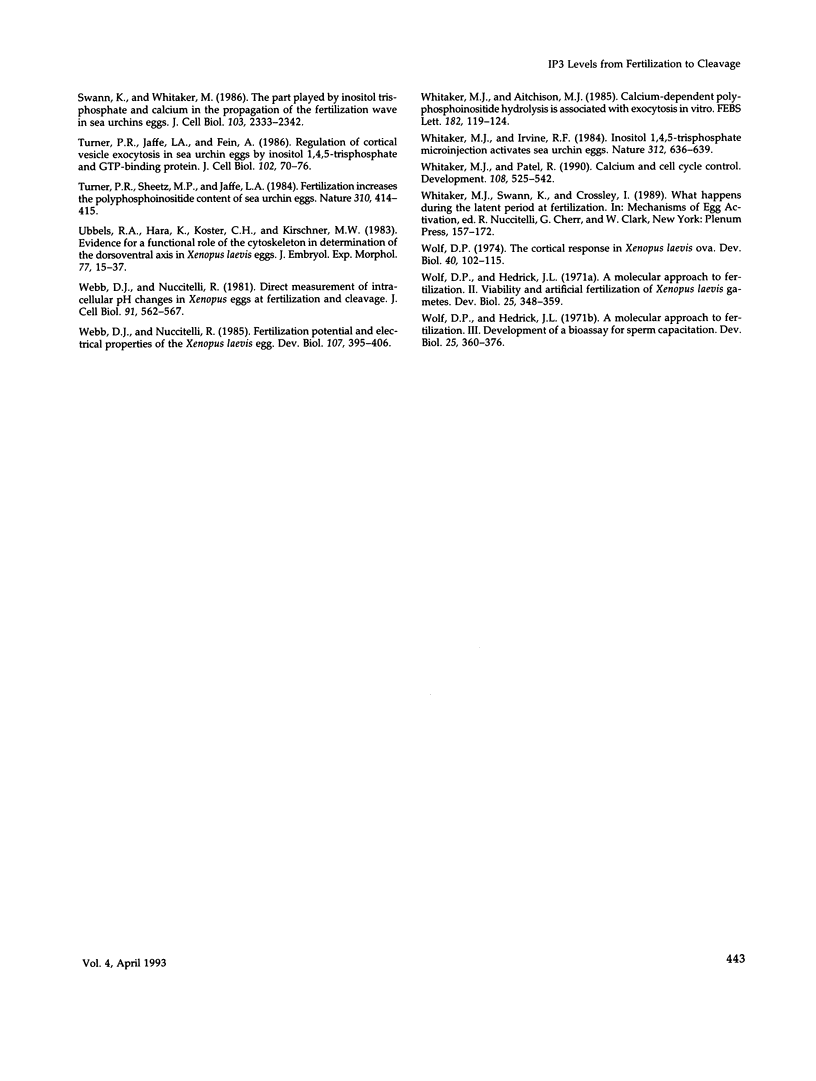
Selected References
These references are in PubMed. This may not be the complete list of references from this article.
- ALLEN R. D., GRIFFIN J. L. The time sequence of early events in the fertilization of sea urchin eggs. I. The latent period and the cortical reaction. Exp Cell Res. 1958 Aug;15(1):163–173. doi: 10.1016/0014-4827(58)90072-7. [DOI] [PubMed] [Google Scholar]
- Alonso T. S., Bonini de Romanelli I. C., Bazan N. G. Changes in triacylglycerol, diacylglycerol and free fatty acids after fertilization in developing toad embryos. Biochim Biophys Acta. 1986 Feb 28;875(3):465–472. doi: 10.1016/0005-2760(86)90066-4. [DOI] [PubMed] [Google Scholar]
- Baker P. F., Warner A. E. Intracellular calcium and cell cleavage in early embryos of Xenopus laevis. J Cell Biol. 1972 May;53(2):579–581. doi: 10.1083/jcb.53.2.579. [DOI] [PMC free article] [PubMed] [Google Scholar]
- Busa W. B., Ferguson J. E., Joseph S. K., Williamson J. R., Nuccitelli R. Activation of frog (Xenopus laevis) eggs by inositol trisphosphate. I. Characterization of Ca2+ release from intracellular stores. J Cell Biol. 1985 Aug;101(2):677–682. doi: 10.1083/jcb.101.2.677. [DOI] [PMC free article] [PubMed] [Google Scholar]
- Busa W. B., Nuccitelli R. An elevated free cytosolic Ca2+ wave follows fertilization in eggs of the frog, Xenopus laevis. J Cell Biol. 1985 Apr;100(4):1325–1329. doi: 10.1083/jcb.100.4.1325. [DOI] [PMC free article] [PubMed] [Google Scholar]
- Busa W. B. Roles for the phosphatidylinositol cycle in early development. Philos Trans R Soc Lond B Biol Sci. 1988 Jul 26;320(1199):415–426. doi: 10.1098/rstb.1988.0085. [DOI] [PubMed] [Google Scholar]
- Ciapa B., Whitaker M. Two phases of inositol polyphosphate and diacylglycerol production at fertilisation. FEBS Lett. 1986 Jan 20;195(1-2):347–351. doi: 10.1016/0014-5793(86)80191-0. [DOI] [PubMed] [Google Scholar]
- Cicirelli M. F., Robinson K. R., Smith L. D. Internal pH of Xenopus oocytes: a study of the mechanism and role of pH changes during meiotic maturation. Dev Biol. 1983 Nov;100(1):133–146. doi: 10.1016/0012-1606(83)90204-x. [DOI] [PubMed] [Google Scholar]
- Clapper D. L., Walseth T. F., Dargie P. J., Lee H. C. Pyridine nucleotide metabolites stimulate calcium release from sea urchin egg microsomes desensitized to inositol trisphosphate. J Biol Chem. 1987 Jul 15;262(20):9561–9568. [PubMed] [Google Scholar]
- DeLisle S., Welsh M. J. Inositol trisphosphate is required for the propagation of calcium waves in Xenopus oocytes. J Biol Chem. 1992 Apr 25;267(12):7963–7966. [PubMed] [Google Scholar]
- Fluck R. A., Miller A. L., Jaffe L. F. Slow calcium waves accompany cytokinesis in medaka fish eggs. J Cell Biol. 1991 Dec;115(5):1259–1265. doi: 10.1083/jcb.115.5.1259. [DOI] [PMC free article] [PubMed] [Google Scholar]
- Forer A., Sillers P. J. The role of the phosphatidylinositol cycle in mitosis in sea urchin zygotes. Lithium inhibition is overcome by myo-inositol but not by other cyclitols or sugars. Exp Cell Res. 1987 May;170(1):42–55. doi: 10.1016/0014-4827(87)90115-7. [DOI] [PubMed] [Google Scholar]
- Galione A., Lee H. C., Busa W. B. Ca(2+)-induced Ca2+ release in sea urchin egg homogenates: modulation by cyclic ADP-ribose. Science. 1991 Sep 6;253(5024):1143–1146. doi: 10.1126/science.1909457. [DOI] [PubMed] [Google Scholar]
- Gerhart J., Wu M., Kirschner M. Cell cycle dynamics of an M-phase-specific cytoplasmic factor in Xenopus laevis oocytes and eggs. J Cell Biol. 1984 Apr;98(4):1247–1255. doi: 10.1083/jcb.98.4.1247. [DOI] [PMC free article] [PubMed] [Google Scholar]
- Grandin N., Charbonneau M. Intracellular free calcium oscillates during cell division of Xenopus embryos. J Cell Biol. 1991 Feb;112(4):711–718. doi: 10.1083/jcb.112.4.711. [DOI] [PMC free article] [PubMed] [Google Scholar]
- Grandin N., Charbonneau M. The increase in intracellular pH associated with Xenopus egg activation is a Ca(2+)-dependent wave. J Cell Sci. 1992 Jan;101(Pt 1):55–67. doi: 10.1242/jcs.101.1.55. [DOI] [PubMed] [Google Scholar]
- Grey R. D., Bastiani M. J., Webb D. J., Schertel E. R. An electrical block is required to prevent polyspermy in eggs fertilized by natural mating of Xenopus laevis. Dev Biol. 1982 Feb;89(2):475–484. doi: 10.1016/0012-1606(82)90335-9. [DOI] [PubMed] [Google Scholar]
- Han J. K., Fukami K., Nuccitelli R. Reducing inositol lipid hydrolysis, Ins(1,4,5)P3 receptor availability, or Ca2+ gradients lengthens the duration of the cell cycle in Xenopus laevis blastomeres. J Cell Biol. 1992 Jan;116(1):147–156. doi: 10.1083/jcb.116.1.147. [DOI] [PMC free article] [PubMed] [Google Scholar]
- Houle J. G., Wasserman W. J. Intracellular pH plays a role in regulating protein synthesis in Xenopus oocytes. Dev Biol. 1983 Jun;97(2):302–312. doi: 10.1016/0012-1606(83)90088-x. [DOI] [PubMed] [Google Scholar]
- Kamel L. C., Bailey J., Schoenbaum L., Kinsey W. Phosphatidylinositol metabolism during fertilization in the sea urchin egg. Lipids. 1985 Jun;20(6):350–356. doi: 10.1007/BF02534201. [DOI] [PubMed] [Google Scholar]
- Kline D. Calcium-dependent events at fertilization of the frog egg: injection of a calcium buffer blocks ion channel opening, exocytosis, and formation of pronuclei. Dev Biol. 1988 Apr;126(2):346–361. doi: 10.1016/0012-1606(88)90145-5. [DOI] [PubMed] [Google Scholar]
- Kline D., Kopf G. S., Muncy L. F., Jaffe L. A. Evidence for the involvement of a pertussis toxin-insensitive G-protein in egg activation of the frog, Xenopus laevis. Dev Biol. 1991 Feb;143(2):218–229. doi: 10.1016/0012-1606(91)90072-b. [DOI] [PubMed] [Google Scholar]
- Kubota H. Y., Yoshimoto Y., Yoneda M., Hiramoto Y. Free calcium wave upon activation in Xenopus eggs. Dev Biol. 1987 Jan;119(1):129–136. doi: 10.1016/0012-1606(87)90214-4. [DOI] [PubMed] [Google Scholar]
- Larabell C., Nuccitelli R. Inositol lipid hydrolysis contributes to the Ca2+ wave in the activating egg of Xenopus laevis. Dev Biol. 1992 Oct;153(2):347–355. doi: 10.1016/0012-1606(92)90119-2. [DOI] [PubMed] [Google Scholar]
- Le Peuch C. j., Picard A., Dorée M. Parthenogenetic activation decreases the polyphosphoinositide content of frog eggs. FEBS Lett. 1985 Jul 22;187(1):61–64. doi: 10.1016/0014-5793(85)81214-x. [DOI] [PubMed] [Google Scholar]
- Lechleiter J. D., Clapham D. E. Molecular mechanisms of intracellular calcium excitability in X. laevis oocytes. Cell. 1992 Apr 17;69(2):283–294. doi: 10.1016/0092-8674(92)90409-6. [DOI] [PubMed] [Google Scholar]
- Maslanski J. A., Leshko L., Busa W. B. Lithium-sensitive production of inositol phosphates during amphibian embryonic mesoderm induction. Science. 1992 Apr 10;256(5054):243–245. doi: 10.1126/science.1314424. [DOI] [PubMed] [Google Scholar]
- McPherson S. M., McPherson P. S., Mathews L., Campbell K. P., Longo F. J. Cortical localization of a calcium release channel in sea urchin eggs. J Cell Biol. 1992 Mar;116(5):1111–1121. doi: 10.1083/jcb.116.5.1111. [DOI] [PMC free article] [PubMed] [Google Scholar]
- Miyazaki S., Yuzaki M., Nakada K., Shirakawa H., Nakanishi S., Nakade S., Mikoshiba K. Block of Ca2+ wave and Ca2+ oscillation by antibody to the inositol 1,4,5-trisphosphate receptor in fertilized hamster eggs. Science. 1992 Jul 10;257(5067):251–255. doi: 10.1126/science.1321497. [DOI] [PubMed] [Google Scholar]
- Nuccitelli R. How do sperm activate eggs? Curr Top Dev Biol. 1991;25:1–16. doi: 10.1016/s0070-2153(08)60409-3. [DOI] [PubMed] [Google Scholar]
- Parker I., Ivorra I. Inhibition by Ca2+ of inositol trisphosphate-mediated Ca2+ liberation: a possible mechanism for oscillatory release of Ca2+. Proc Natl Acad Sci U S A. 1990 Jan;87(1):260–264. doi: 10.1073/pnas.87.1.260. [DOI] [PMC free article] [PubMed] [Google Scholar]
- Parys J. B., Sernett S. W., DeLisle S., Snyder P. M., Welsh M. J., Campbell K. P. Isolation, characterization, and localization of the inositol 1,4,5-trisphosphate receptor protein in Xenopus laevis oocytes. J Biol Chem. 1992 Sep 15;267(26):18776–18782. [PubMed] [Google Scholar]
- Picard A., Giraud F., Le Bouffant F., Sladeczek F., Le Peuch C., Dorée M. Inositol 1,4,5-triphosphate microinjection triggers activation, but not meiotic maturation in amphibian and starfish oocytes. FEBS Lett. 1985 Mar 25;182(2):446–450. doi: 10.1016/0014-5793(85)80351-3. [DOI] [PubMed] [Google Scholar]
- Rakow T. L., Shen S. S. Multiple stores of calcium are released in the sea urchin egg during fertilization. Proc Natl Acad Sci U S A. 1990 Dec;87(23):9285–9289. doi: 10.1073/pnas.87.23.9285. [DOI] [PMC free article] [PubMed] [Google Scholar]
- Rink T. J., Tsien R. Y., Warner A. E. Free calcium in Xenopus embryos measured with ion-selective microelectrodes. Nature. 1980 Feb 14;283(5748):658–660. doi: 10.1038/283658a0. [DOI] [PubMed] [Google Scholar]
- Steinhardt R. A., Epel D. Activation of sea-urchin eggs by a calcium ionophore. Proc Natl Acad Sci U S A. 1974 May;71(5):1915–1919. doi: 10.1073/pnas.71.5.1915. [DOI] [PMC free article] [PubMed] [Google Scholar]
- Stith B. J., Jaynes C., Goalstone M., Silva S. Insulin and progesterone increase 32PO4-labeling of phospholipids and inositol 1,4,5-trisphosphate mass in Xenopus oocytes. Cell Calcium. 1992 May;13(5):341–352. doi: 10.1016/0143-4160(92)90069-5. [DOI] [PubMed] [Google Scholar]
- Stith B. J., Proctor W. R. Microinjection of inositol 1,2-(cyclic)-4,5-trisphosphate, inositol 1,3,4,5-tetrakisphosphate, and inositol 1,4,5-trisphosphate into intact Xenopus oocytes can induce membrane currents independent of extracellular calcium. J Cell Biochem. 1989 Jul;40(3):321–330. doi: 10.1002/jcb.240400308. [DOI] [PubMed] [Google Scholar]
- Swann K., McCulloh D. H., McDougall A., Chambers E. L., Whitaker M. Sperm-induced currents at fertilization in sea urchin eggs injected with EGTA and neomycin. Dev Biol. 1992 Jun;151(2):552–563. doi: 10.1016/0012-1606(92)90193-k. [DOI] [PubMed] [Google Scholar]
- Swann K., Whitaker M. The part played by inositol trisphosphate and calcium in the propagation of the fertilization wave in sea urchin eggs. J Cell Biol. 1986 Dec;103(6 Pt 1):2333–2342. doi: 10.1083/jcb.103.6.2333. [DOI] [PMC free article] [PubMed] [Google Scholar]
- Turner P. R., Jaffe L. A., Fein A. Regulation of cortical vesicle exocytosis in sea urchin eggs by inositol 1,4,5-trisphosphate and GTP-binding protein. J Cell Biol. 1986 Jan;102(1):70–76. doi: 10.1083/jcb.102.1.70. [DOI] [PMC free article] [PubMed] [Google Scholar]
- Turner P. R., Sheetz M. P., Jaffe L. A. Fertilization increases the polyphosphoinositide content of sea urchin eggs. Nature. 1984 Aug 2;310(5976):414–415. doi: 10.1038/310414a0. [DOI] [PubMed] [Google Scholar]
- Ubbels G. A., Hara K., Koster C. H., Kirschner M. W. Evidence for a functional role of the cytoskeleton in determination of the dorsoventral axis in Xenopus laevis eggs. J Embryol Exp Morphol. 1983 Oct;77:15–37. [PubMed] [Google Scholar]
- Webb D. J., Nuccitelli R. Direct measurement of intracellular pH changes in Xenopus eggs at fertilization and cleavage. J Cell Biol. 1981 Nov;91(2 Pt 1):562–567. doi: 10.1083/jcb.91.2.562. [DOI] [PMC free article] [PubMed] [Google Scholar]
- Webb D. J., Nuccitelli R. Fertilization potential and electrical properties of the Xenopus laevis egg. Dev Biol. 1985 Feb;107(2):395–406. doi: 10.1016/0012-1606(85)90321-5. [DOI] [PubMed] [Google Scholar]
- Whitaker M., Aitchison M. Calcium-dependent polyphosphoinositide hydrolysis is associated with exocytosis in vitro. FEBS Lett. 1985 Mar 11;182(1):119–124. doi: 10.1016/0014-5793(85)81167-4. [DOI] [PubMed] [Google Scholar]
- Whitaker M., Patel R. Calcium and cell cycle control. Development. 1990 Apr;108(4):525–542. doi: 10.1242/dev.108.4.525. [DOI] [PubMed] [Google Scholar]
- Wolf D. P., Hedrick J. L. A molecular approach to fertilization. 3. Development of a bioassay for sperm capacitation. Dev Biol. 1971 Jul;25(3):360–376. doi: 10.1016/0012-1606(71)90037-6. [DOI] [PubMed] [Google Scholar]
- Wolf D. P., Hedrick J. L. A molecular approach to fertilization. II. Viability and artificial fertilization of Xenopus laevis gemetes. Dev Biol. 1971 Jul;25(3):348–359. doi: 10.1016/0012-1606(71)90036-4. [DOI] [PubMed] [Google Scholar]
- Wolf D. P. The cortical response in Xenopus laevis ova. Dev Biol. 1974 Sep;40(1):102–115. doi: 10.1016/0012-1606(74)90112-2. [DOI] [PubMed] [Google Scholar]