Abstract
Kss1 protein kinase, and the homologous Fus3 kinase, are required for pheromone signal transduction in Saccharomyces cerevisiae. In MATa haploids exposed to alpha-factor, Kss1 was rapidly phosphorylated on both Thr183 and Tyr185, and both sites were required for Kss1 function in vivo. De novo protein synthesis was required for sustained pheromone-induced phosphorylation of Kss1. Catalytically inactive Kss1 mutants displayed alpha-factor-induced phosphorylation on both residues, even in kss1 delta cells; hence, autophosphorylation is not obligatory for these modifications. In kss1 delta fus3 delta double mutants, Kss1 phosphorylation was elevated even in the absence of pheromone; thus, cross-phosphorylation by Fus3 is not responsible for Kss1 activation. In contrast, pheromone-induced Kss1 phosphorylation was eliminated in mutants deficient in two other protein kinases, Ste11 and Ste7. A dominant hyperactive allele of STE11 caused a dramatic increase in the phosphorylation of Kss1, even in the absence of pheromone stimulation, but required Ste7 for this effect, suggesting an order of function: Ste11-->Ste7-->Kss1. When overproduced, Kss1 stimulated recovery from pheromone-imposed G1 arrest. Catalytic activity was essential for Kss1 function in signal transmission, but not for its recovery-promoting activity. Kss1 was found almost exclusively in the particulate material and its subcellular fractionation was unaffected by pheromone treatment. Indirect immunofluorescence demonstrated that Kss1 is concentrated in the nucleus and that its distribution is not altered detectably during signaling.
Full text
PDF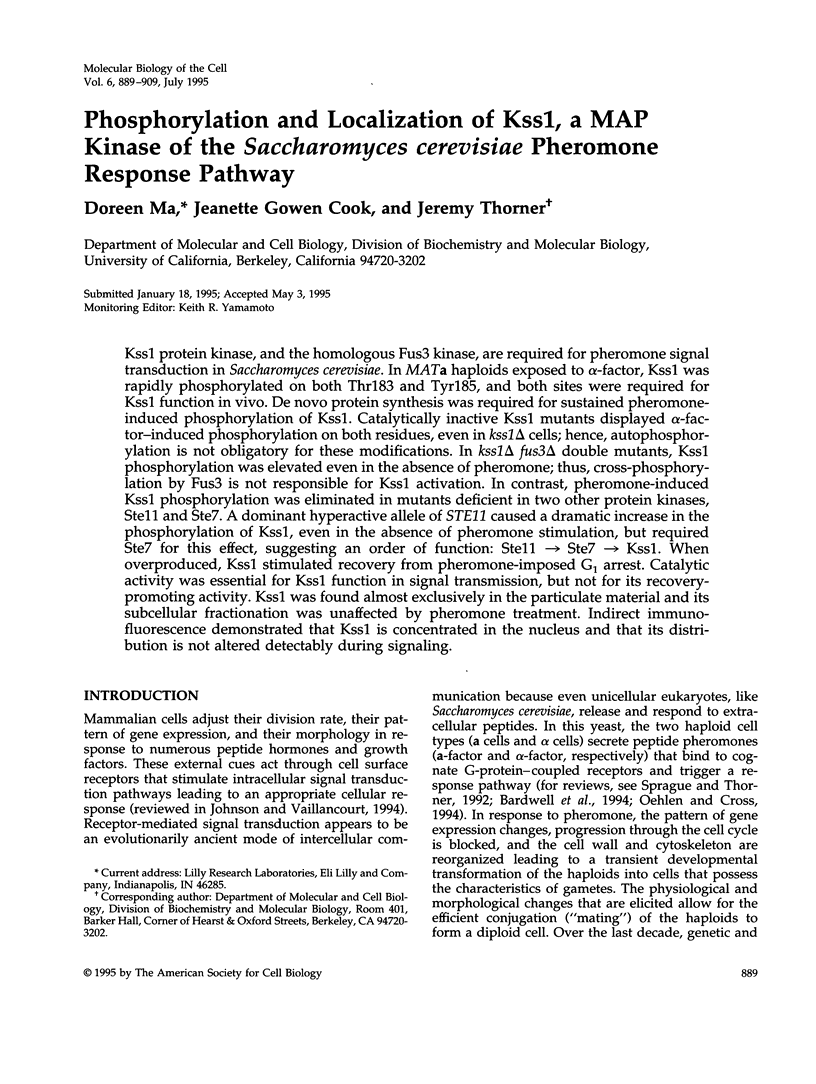
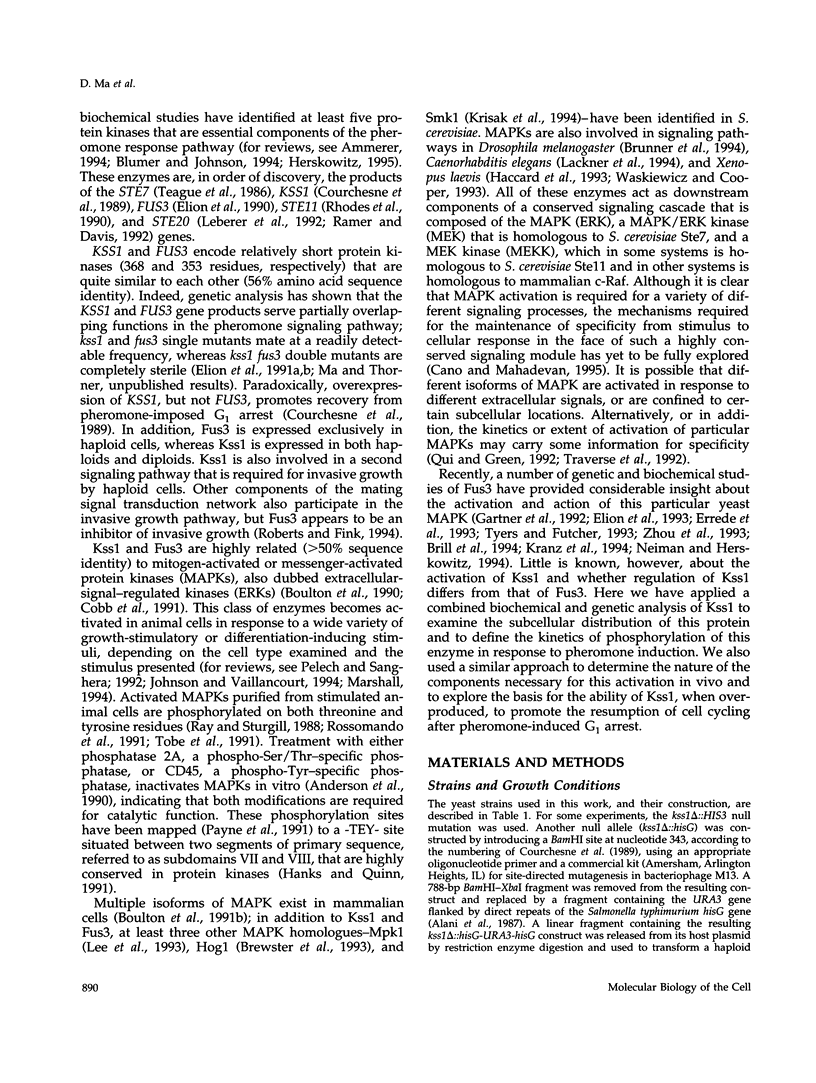
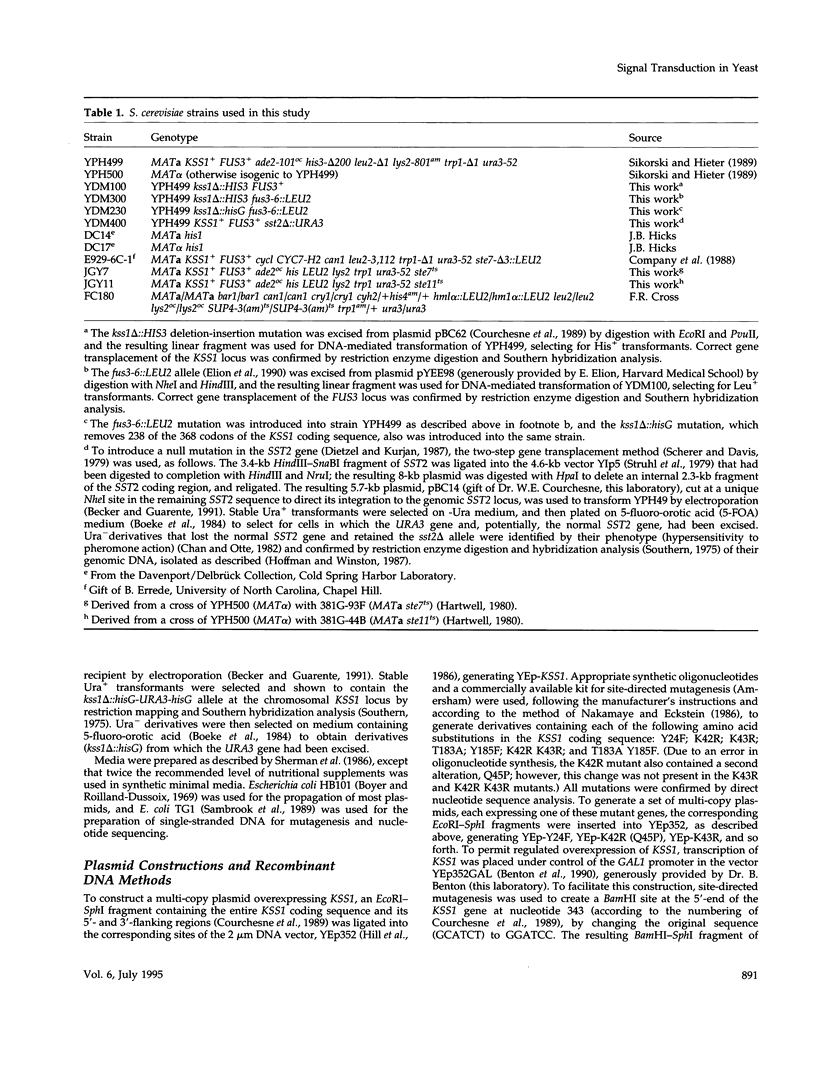
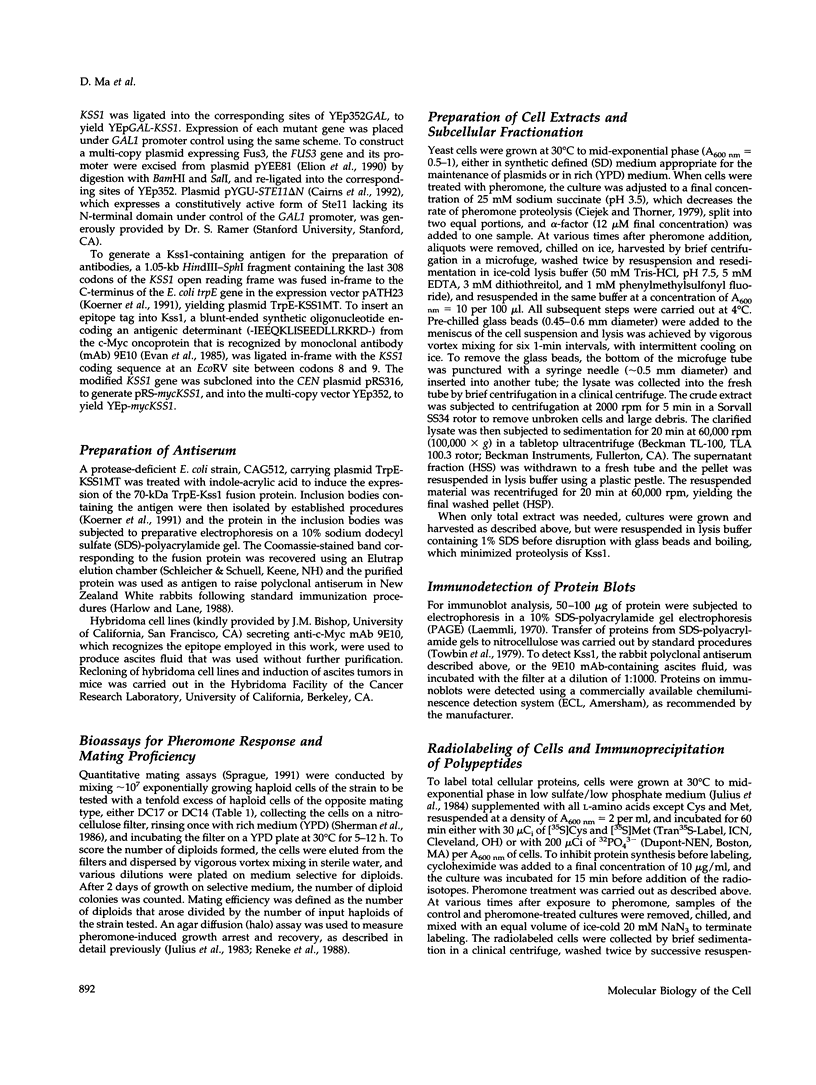
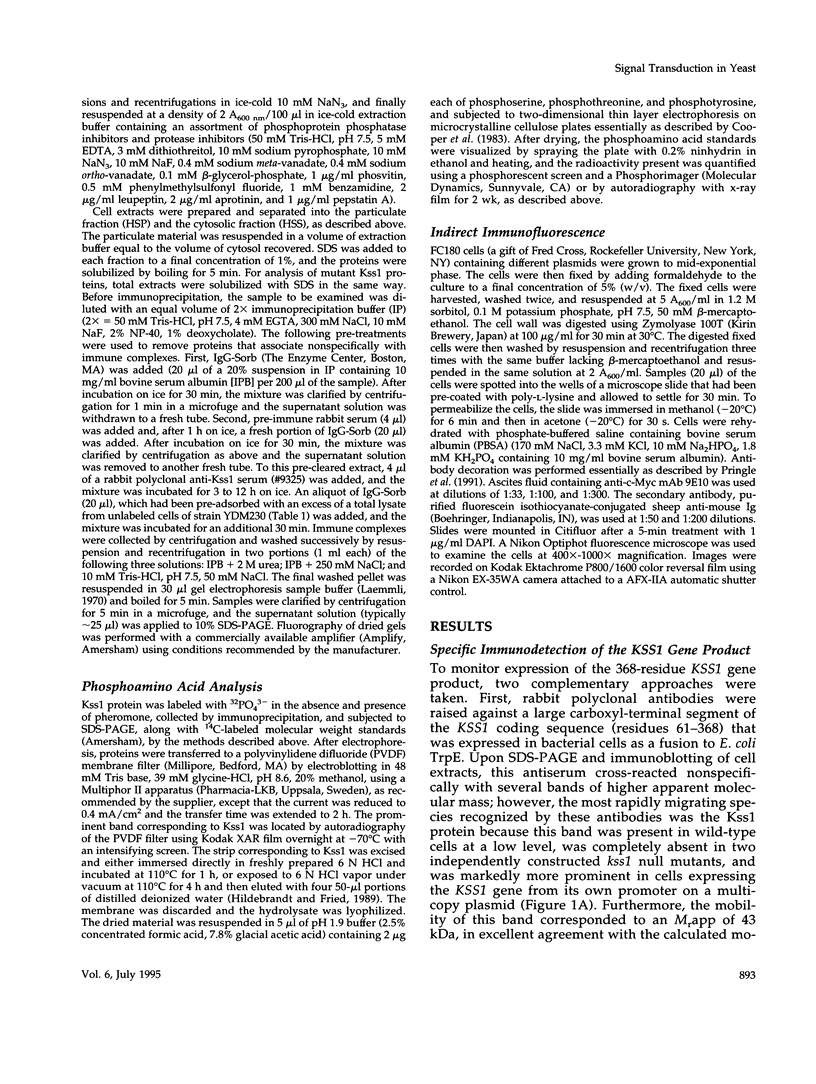
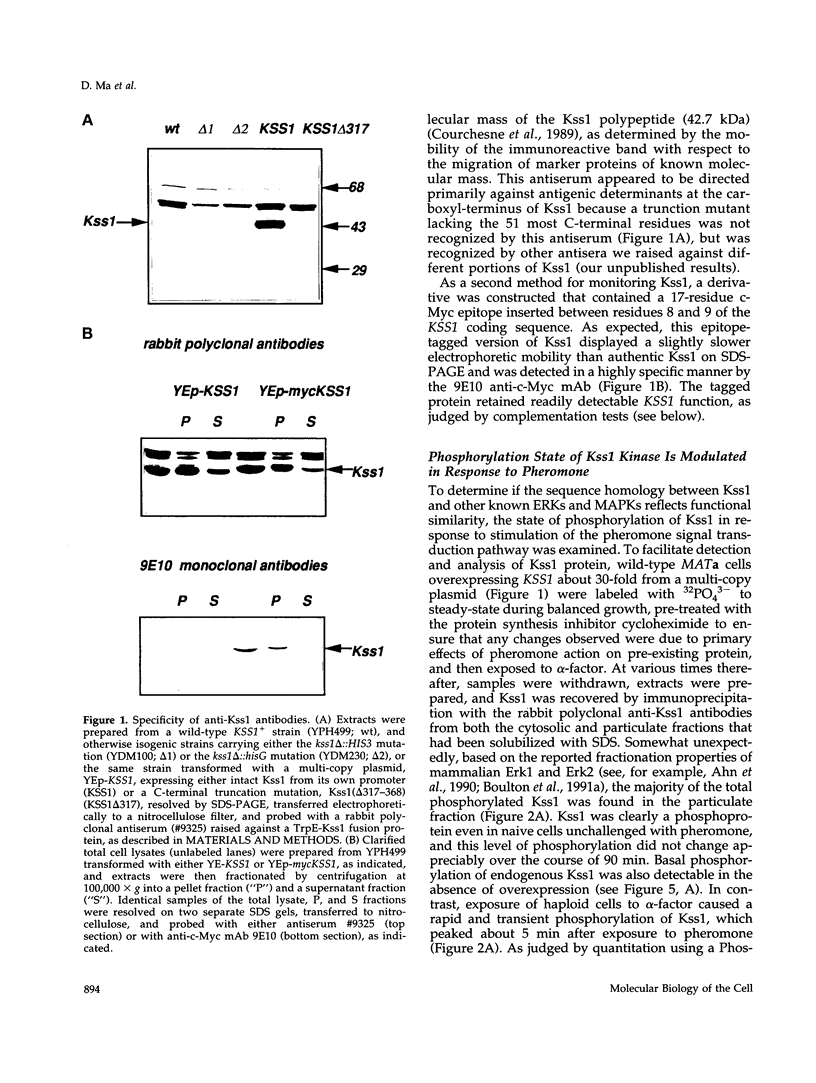
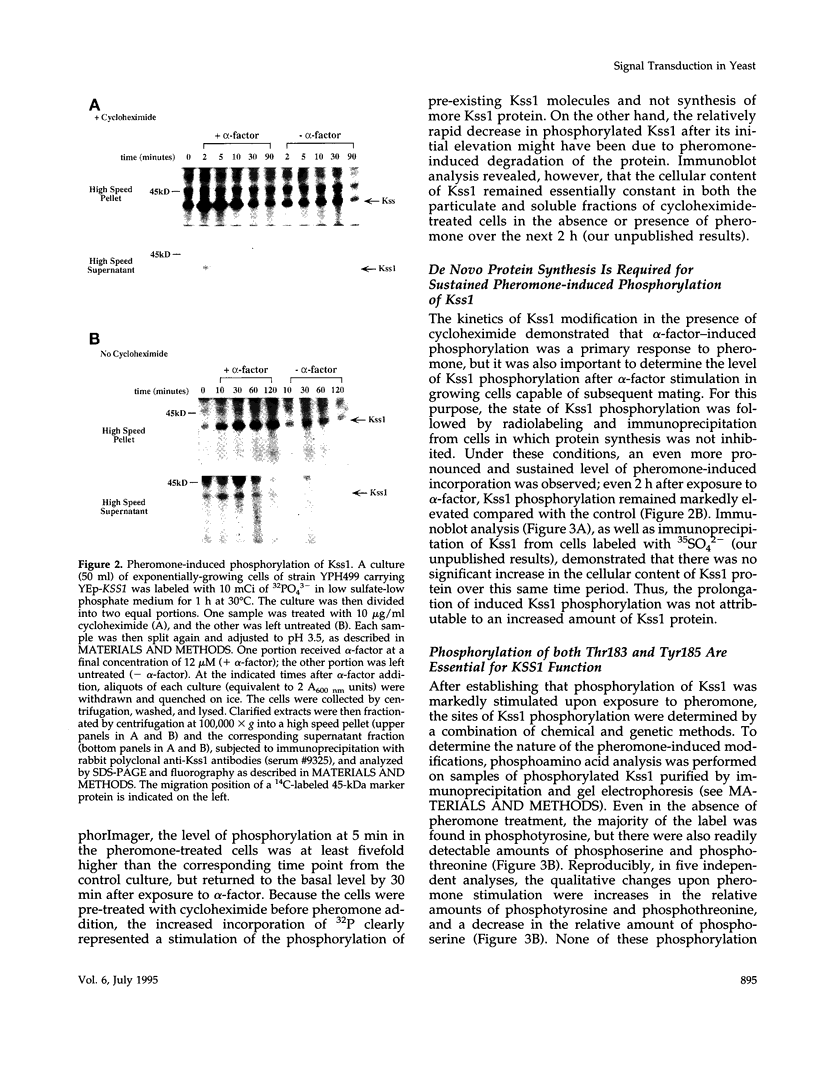
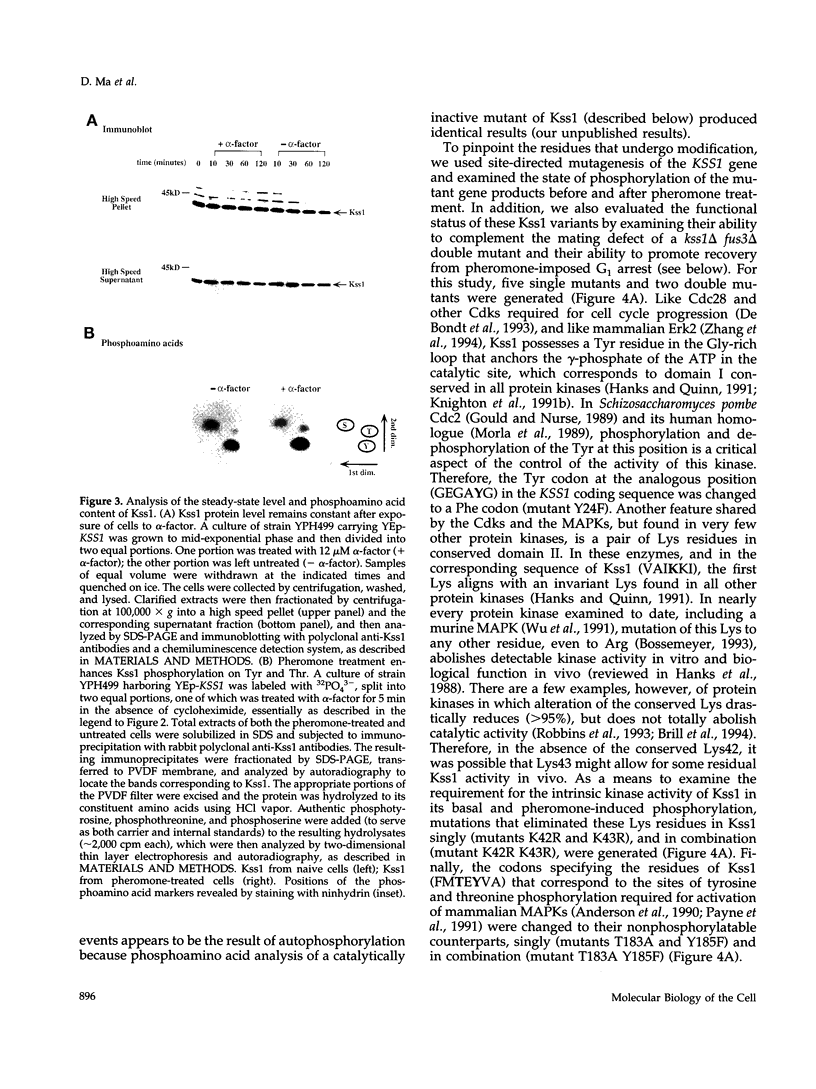
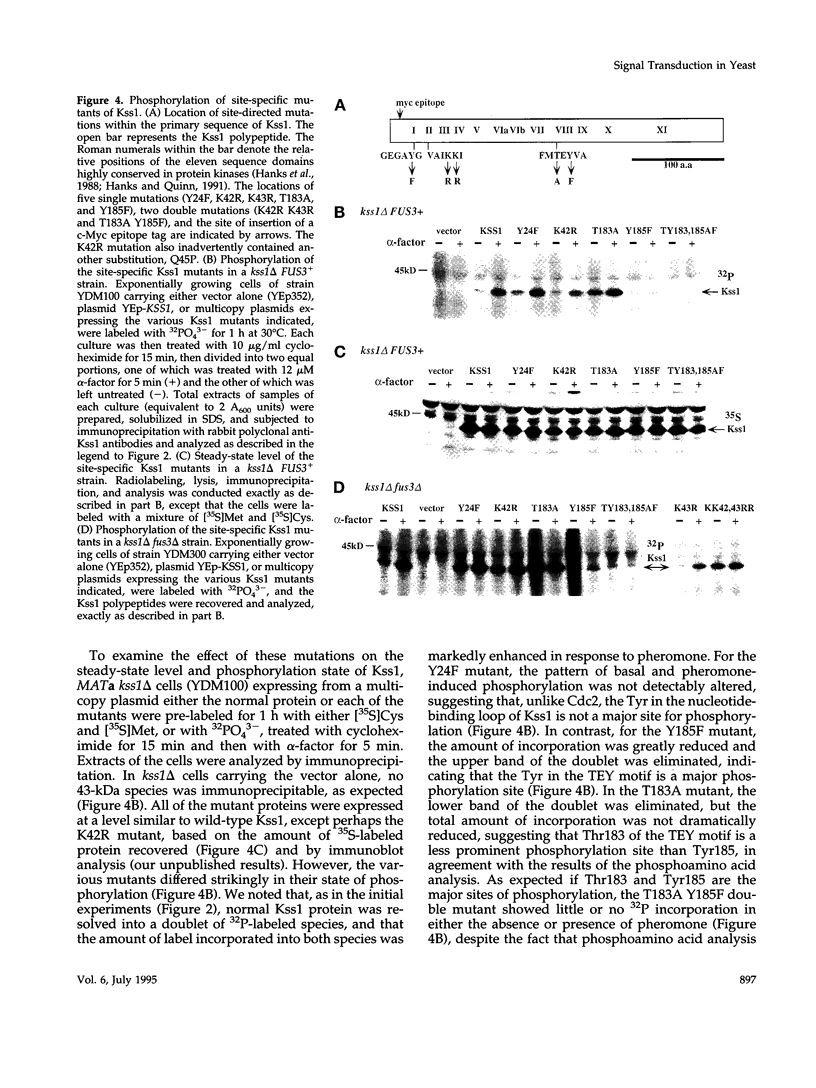
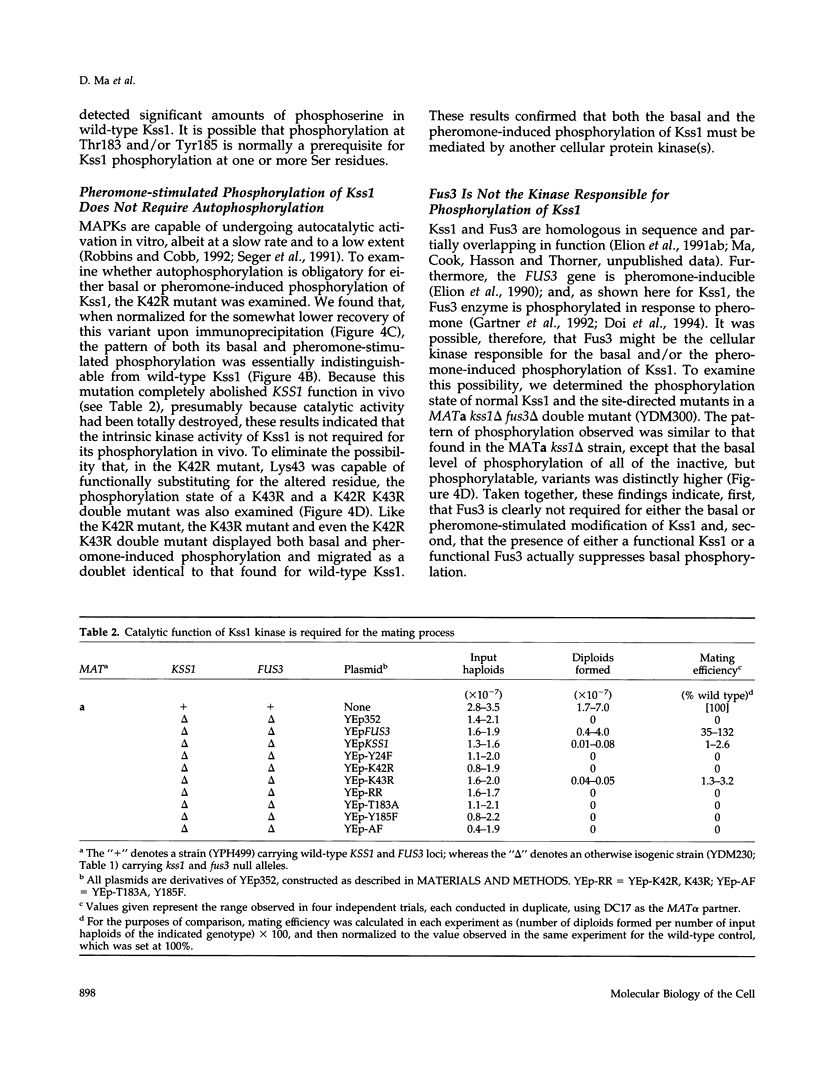
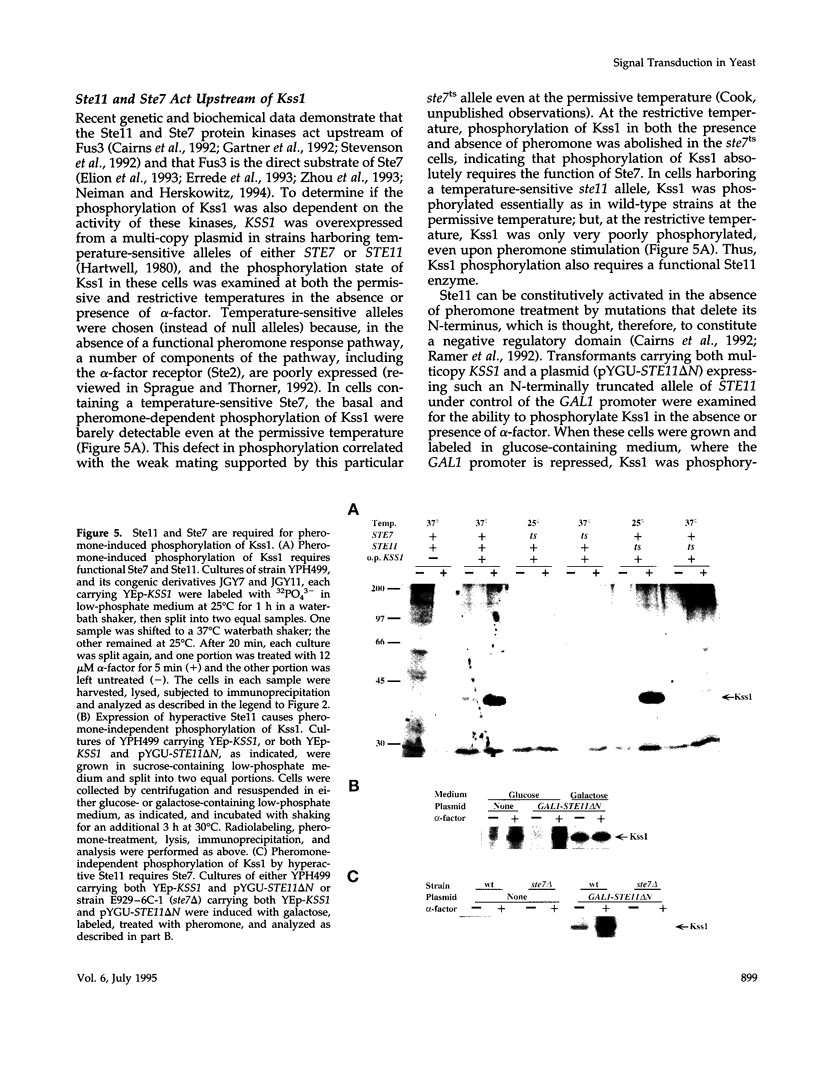
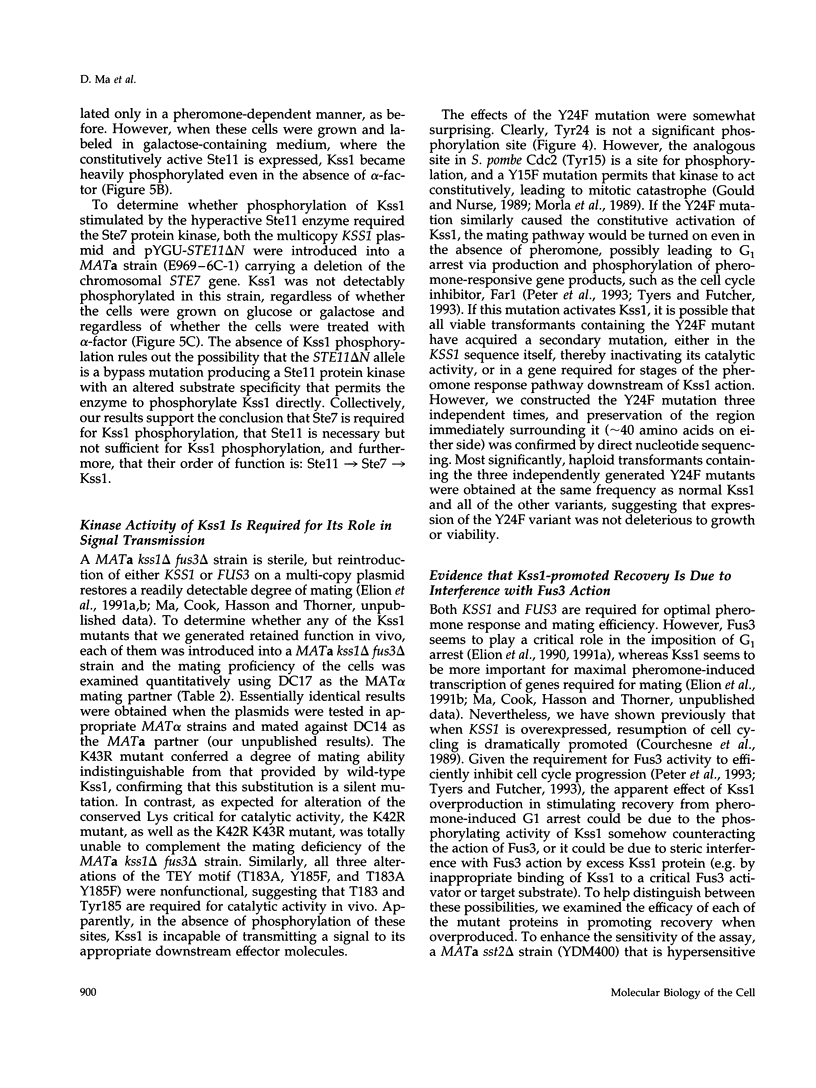
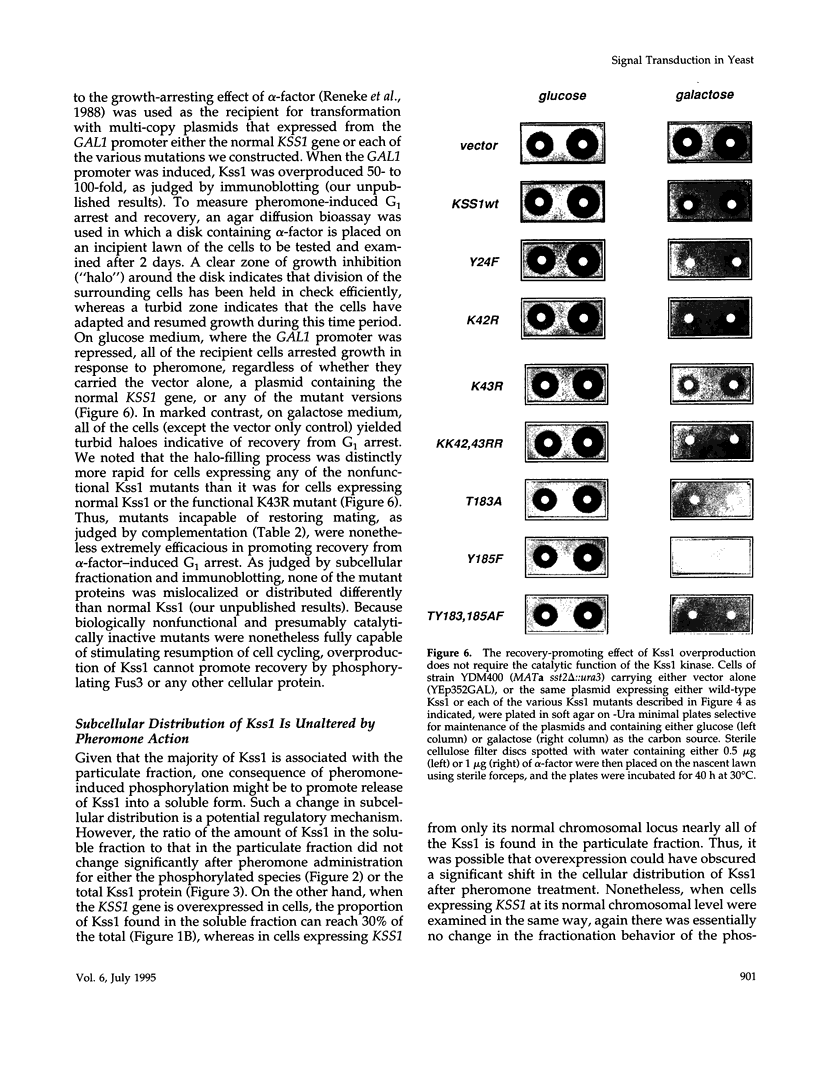
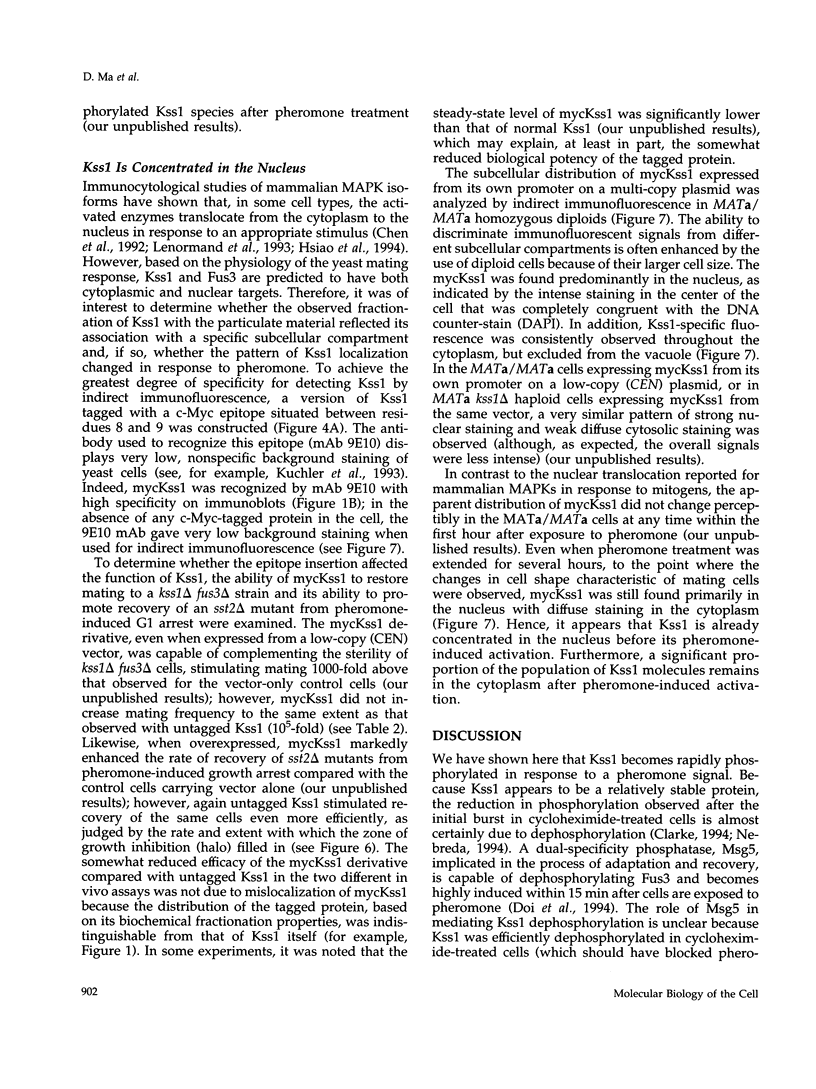
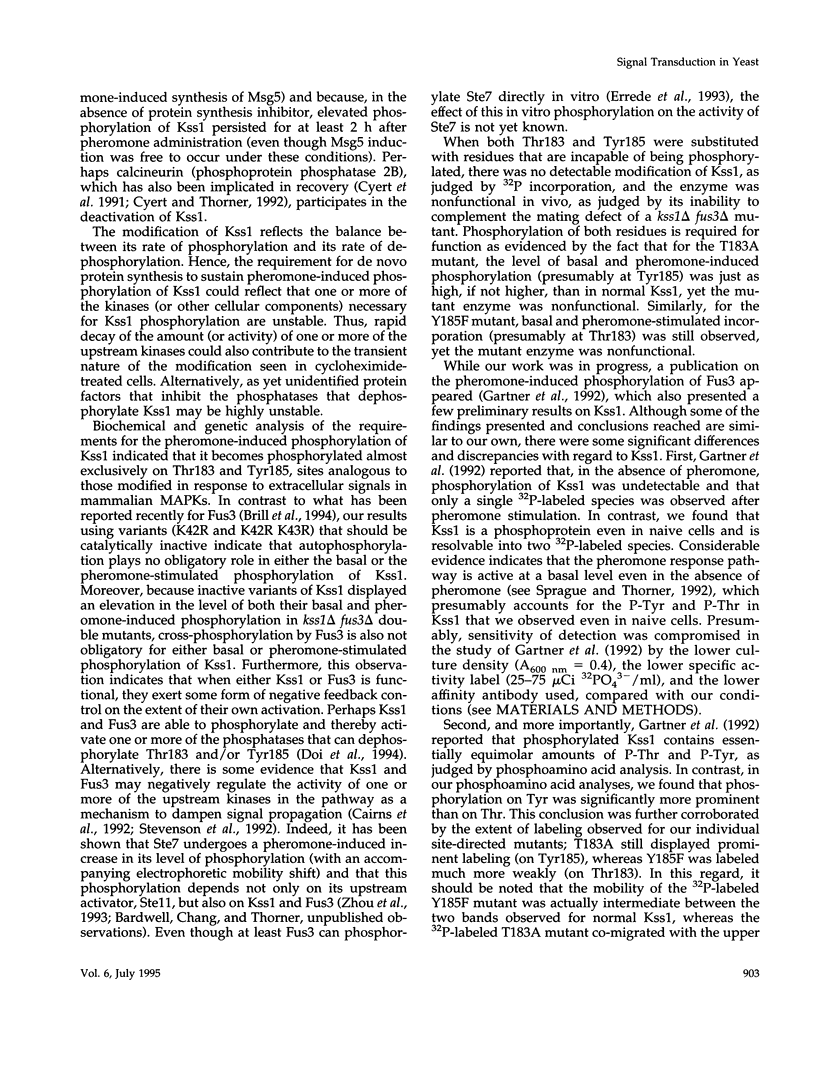
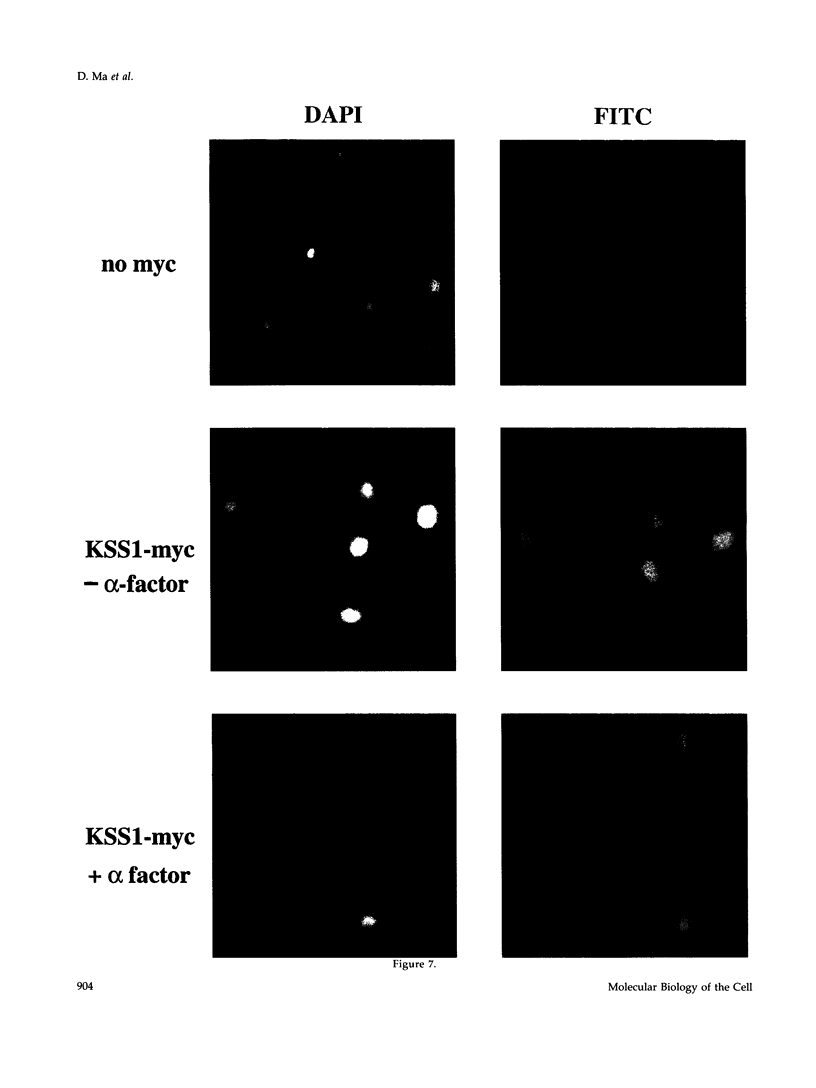
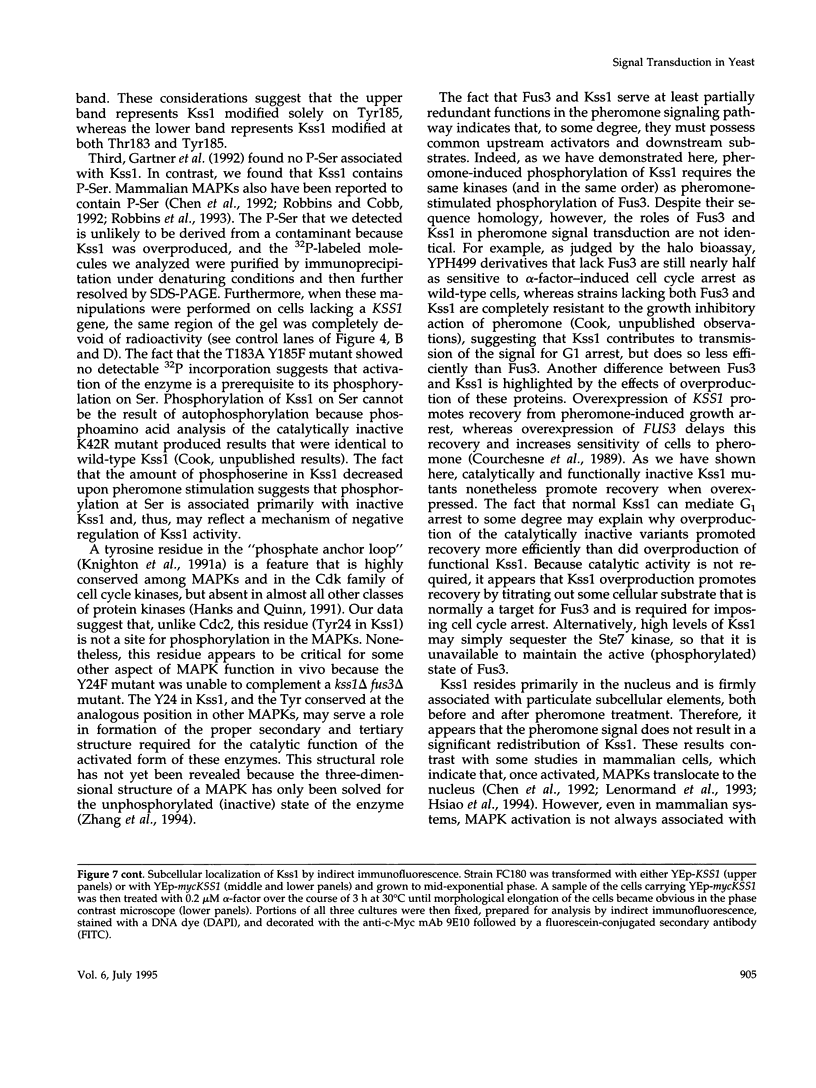
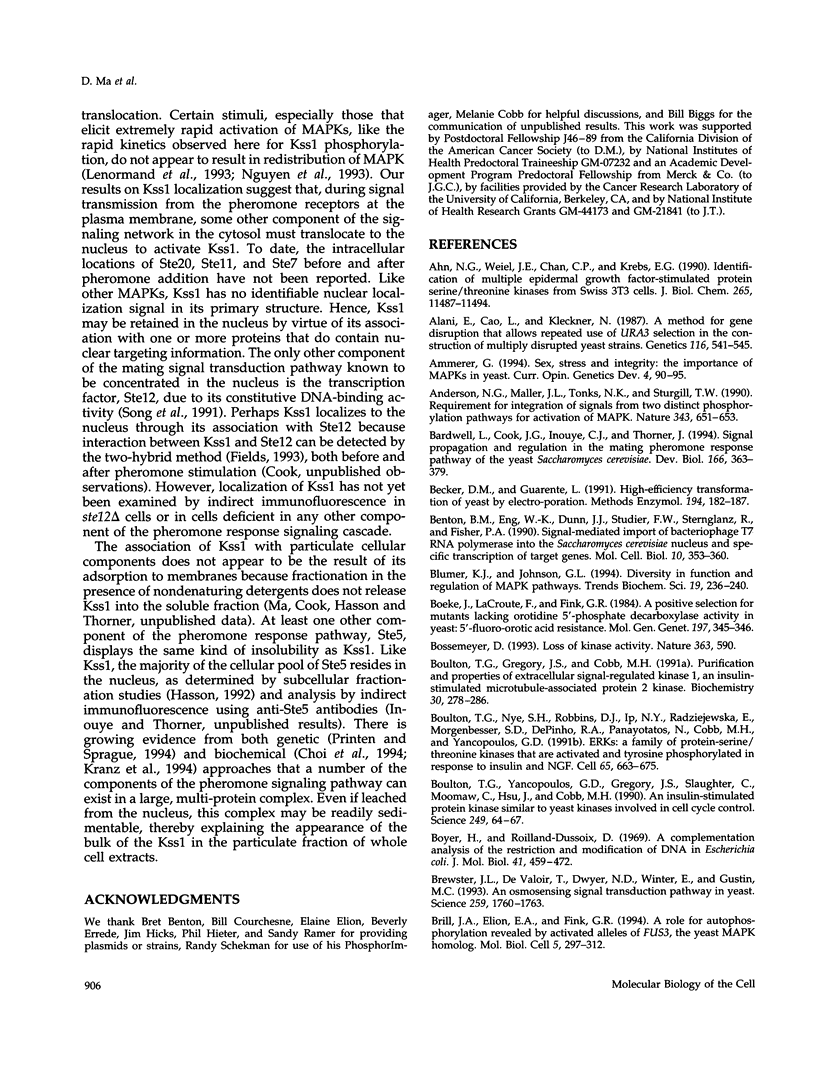
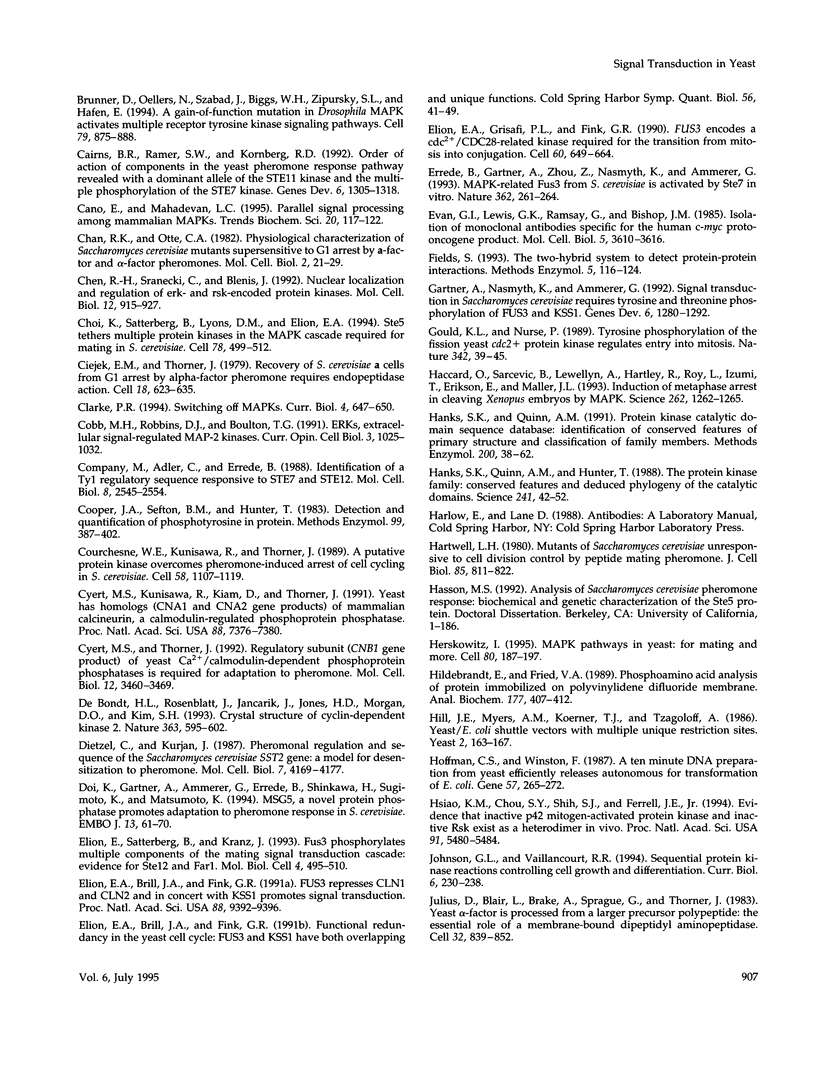
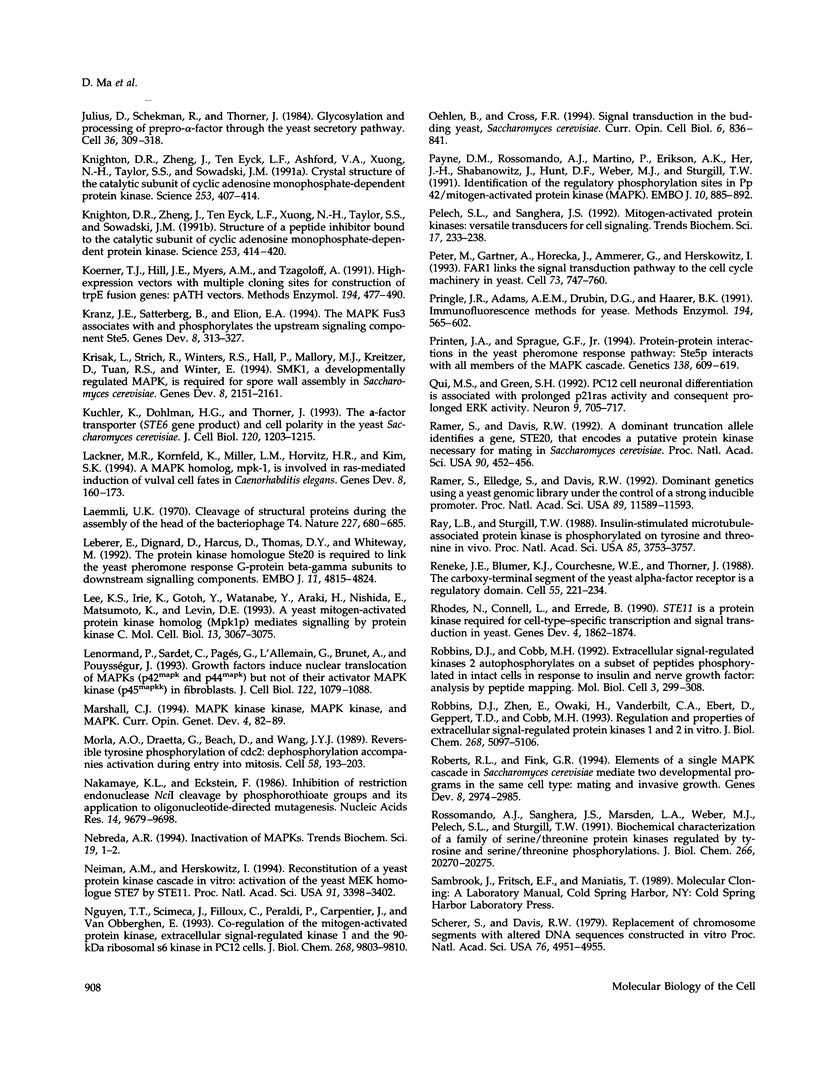
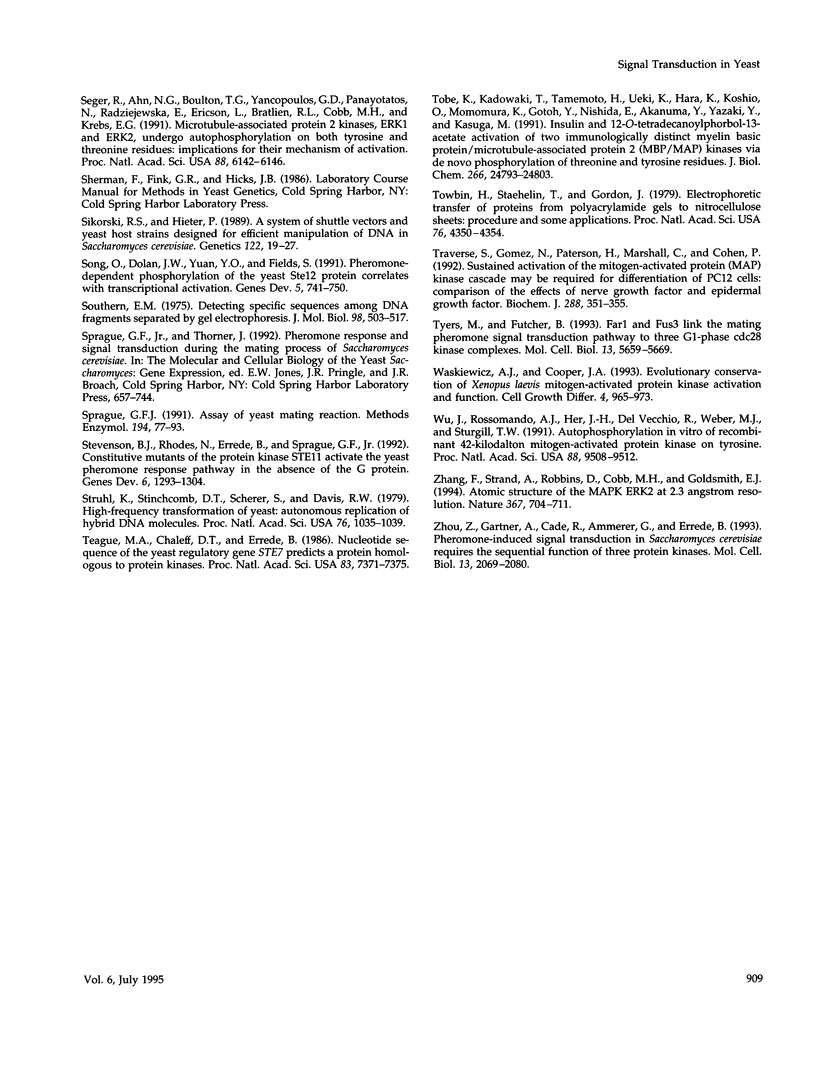
Images in this article
Selected References
These references are in PubMed. This may not be the complete list of references from this article.
- Ahn N. G., Weiel J. E., Chan C. P., Krebs E. G. Identification of multiple epidermal growth factor-stimulated protein serine/threonine kinases from Swiss 3T3 cells. J Biol Chem. 1990 Jul 15;265(20):11487–11494. [PubMed] [Google Scholar]
- Alani E., Cao L., Kleckner N. A method for gene disruption that allows repeated use of URA3 selection in the construction of multiply disrupted yeast strains. Genetics. 1987 Aug;116(4):541–545. doi: 10.1534/genetics.112.541.test. [DOI] [PMC free article] [PubMed] [Google Scholar]
- Ammerer G. Sex, stress and integrity: the importance of MAP kinases in yeast. Curr Opin Genet Dev. 1994 Feb;4(1):90–95. doi: 10.1016/0959-437x(94)90096-5. [DOI] [PubMed] [Google Scholar]
- Anderson N. G., Maller J. L., Tonks N. K., Sturgill T. W. Requirement for integration of signals from two distinct phosphorylation pathways for activation of MAP kinase. Nature. 1990 Feb 15;343(6259):651–653. doi: 10.1038/343651a0. [DOI] [PubMed] [Google Scholar]
- Bardwell L., Cook J. G., Inouye C. J., Thorner J. Signal propagation and regulation in the mating pheromone response pathway of the yeast Saccharomyces cerevisiae. Dev Biol. 1994 Dec;166(2):363–379. doi: 10.1006/dbio.1994.1323. [DOI] [PubMed] [Google Scholar]
- Becker D. M., Guarente L. High-efficiency transformation of yeast by electroporation. Methods Enzymol. 1991;194:182–187. doi: 10.1016/0076-6879(91)94015-5. [DOI] [PubMed] [Google Scholar]
- Benton B. M., Eng W. K., Dunn J. J., Studier F. W., Sternglanz R., Fisher P. A. Signal-mediated import of bacteriophage T7 RNA polymerase into the Saccharomyces cerevisiae nucleus and specific transcription of target genes. Mol Cell Biol. 1990 Jan;10(1):353–360. doi: 10.1128/mcb.10.1.353. [DOI] [PMC free article] [PubMed] [Google Scholar]
- Blumer K. J., Johnson G. L. Diversity in function and regulation of MAP kinase pathways. Trends Biochem Sci. 1994 Jun;19(6):236–240. doi: 10.1016/0968-0004(94)90147-3. [DOI] [PubMed] [Google Scholar]
- Boeke J. D., LaCroute F., Fink G. R. A positive selection for mutants lacking orotidine-5'-phosphate decarboxylase activity in yeast: 5-fluoro-orotic acid resistance. Mol Gen Genet. 1984;197(2):345–346. doi: 10.1007/BF00330984. [DOI] [PubMed] [Google Scholar]
- Bossemeyer D. Loss of kinase activity. Nature. 1993 Jun 17;363(6430):590–590. doi: 10.1038/363590a0. [DOI] [PubMed] [Google Scholar]
- Boulton T. G., Gregory J. S., Cobb M. H. Purification and properties of extracellular signal-regulated kinase 1, an insulin-stimulated microtubule-associated protein 2 kinase. Biochemistry. 1991 Jan 8;30(1):278–286. doi: 10.1021/bi00215a038. [DOI] [PubMed] [Google Scholar]
- Boulton T. G., Nye S. H., Robbins D. J., Ip N. Y., Radziejewska E., Morgenbesser S. D., DePinho R. A., Panayotatos N., Cobb M. H., Yancopoulos G. D. ERKs: a family of protein-serine/threonine kinases that are activated and tyrosine phosphorylated in response to insulin and NGF. Cell. 1991 May 17;65(4):663–675. doi: 10.1016/0092-8674(91)90098-j. [DOI] [PubMed] [Google Scholar]
- Boulton T. G., Yancopoulos G. D., Gregory J. S., Slaughter C., Moomaw C., Hsu J., Cobb M. H. An insulin-stimulated protein kinase similar to yeast kinases involved in cell cycle control. Science. 1990 Jul 6;249(4964):64–67. doi: 10.1126/science.2164259. [DOI] [PubMed] [Google Scholar]
- Boyer H. W., Roulland-Dussoix D. A complementation analysis of the restriction and modification of DNA in Escherichia coli. J Mol Biol. 1969 May 14;41(3):459–472. doi: 10.1016/0022-2836(69)90288-5. [DOI] [PubMed] [Google Scholar]
- Brewster J. L., de Valoir T., Dwyer N. D., Winter E., Gustin M. C. An osmosensing signal transduction pathway in yeast. Science. 1993 Mar 19;259(5102):1760–1763. doi: 10.1126/science.7681220. [DOI] [PubMed] [Google Scholar]
- Brill J. A., Elion E. A., Fink G. R. A role for autophosphorylation revealed by activated alleles of FUS3, the yeast MAP kinase homolog. Mol Biol Cell. 1994 Mar;5(3):297–312. doi: 10.1091/mbc.5.3.297. [DOI] [PMC free article] [PubMed] [Google Scholar]
- Brunner D., Oellers N., Szabad J., Biggs W. H., 3rd, Zipursky S. L., Hafen E. A gain-of-function mutation in Drosophila MAP kinase activates multiple receptor tyrosine kinase signaling pathways. Cell. 1994 Mar 11;76(5):875–888. doi: 10.1016/0092-8674(94)90362-x. [DOI] [PubMed] [Google Scholar]
- Cairns B. R., Ramer S. W., Kornberg R. D. Order of action of components in the yeast pheromone response pathway revealed with a dominant allele of the STE11 kinase and the multiple phosphorylation of the STE7 kinase. Genes Dev. 1992 Jul;6(7):1305–1318. doi: 10.1101/gad.6.7.1305. [DOI] [PubMed] [Google Scholar]
- Cano E., Mahadevan L. C. Parallel signal processing among mammalian MAPKs. Trends Biochem Sci. 1995 Mar;20(3):117–122. doi: 10.1016/s0968-0004(00)88978-1. [DOI] [PubMed] [Google Scholar]
- Chan R. K., Otte C. A. Physiological characterization of Saccharomyces cerevisiae mutants supersensitive to G1 arrest by a factor and alpha factor pheromones. Mol Cell Biol. 1982 Jan;2(1):21–29. doi: 10.1128/mcb.2.1.21. [DOI] [PMC free article] [PubMed] [Google Scholar]
- Chen R. H., Sarnecki C., Blenis J. Nuclear localization and regulation of erk- and rsk-encoded protein kinases. Mol Cell Biol. 1992 Mar;12(3):915–927. doi: 10.1128/mcb.12.3.915. [DOI] [PMC free article] [PubMed] [Google Scholar]
- Choi K. Y., Satterberg B., Lyons D. M., Elion E. A. Ste5 tethers multiple protein kinases in the MAP kinase cascade required for mating in S. cerevisiae. Cell. 1994 Aug 12;78(3):499–512. doi: 10.1016/0092-8674(94)90427-8. [DOI] [PubMed] [Google Scholar]
- Ciejek E., Thorner J. Recovery of S. cerevisiae a cells from G1 arrest by alpha factor pheromone requires endopeptidase action. Cell. 1979 Nov;18(3):623–635. doi: 10.1016/0092-8674(79)90117-x. [DOI] [PubMed] [Google Scholar]
- Clarke P. R. Signal transduction. Switching off MAP kinases. Curr Biol. 1994 Jul 1;4(7):647–650. doi: 10.1016/s0960-9822(00)00144-5. [DOI] [PubMed] [Google Scholar]
- Cobb M. H., Robbins D. J., Boulton T. G. ERKs, extracellular signal-regulated MAP-2 kinases. Curr Opin Cell Biol. 1991 Dec;3(6):1025–1032. doi: 10.1016/0955-0674(91)90124-h. [DOI] [PubMed] [Google Scholar]
- Company M., Adler C., Errede B. Identification of a Ty1 regulatory sequence responsive to STE7 and STE12. Mol Cell Biol. 1988 Jun;8(6):2545–2554. doi: 10.1128/mcb.8.6.2545. [DOI] [PMC free article] [PubMed] [Google Scholar]
- Cooper J. A., Sefton B. M., Hunter T. Detection and quantification of phosphotyrosine in proteins. Methods Enzymol. 1983;99:387–402. doi: 10.1016/0076-6879(83)99075-4. [DOI] [PubMed] [Google Scholar]
- Courchesne W. E., Kunisawa R., Thorner J. A putative protein kinase overcomes pheromone-induced arrest of cell cycling in S. cerevisiae. Cell. 1989 Sep 22;58(6):1107–1119. doi: 10.1016/0092-8674(89)90509-6. [DOI] [PubMed] [Google Scholar]
- Cyert M. S., Kunisawa R., Kaim D., Thorner J. Yeast has homologs (CNA1 and CNA2 gene products) of mammalian calcineurin, a calmodulin-regulated phosphoprotein phosphatase. Proc Natl Acad Sci U S A. 1991 Aug 15;88(16):7376–7380. doi: 10.1073/pnas.88.16.7376. [DOI] [PMC free article] [PubMed] [Google Scholar]
- Cyert M. S., Thorner J. Regulatory subunit (CNB1 gene product) of yeast Ca2+/calmodulin-dependent phosphoprotein phosphatases is required for adaptation to pheromone. Mol Cell Biol. 1992 Aug;12(8):3460–3469. doi: 10.1128/mcb.12.8.3460. [DOI] [PMC free article] [PubMed] [Google Scholar]
- De Bondt H. L., Rosenblatt J., Jancarik J., Jones H. D., Morgan D. O., Kim S. H. Crystal structure of cyclin-dependent kinase 2. Nature. 1993 Jun 17;363(6430):595–602. doi: 10.1038/363595a0. [DOI] [PubMed] [Google Scholar]
- Dietzel C., Kurjan J. Pheromonal regulation and sequence of the Saccharomyces cerevisiae SST2 gene: a model for desensitization to pheromone. Mol Cell Biol. 1987 Dec;7(12):4169–4177. doi: 10.1128/mcb.7.12.4169. [DOI] [PMC free article] [PubMed] [Google Scholar]
- Doi K., Gartner A., Ammerer G., Errede B., Shinkawa H., Sugimoto K., Matsumoto K. MSG5, a novel protein phosphatase promotes adaptation to pheromone response in S. cerevisiae. EMBO J. 1994 Jan 1;13(1):61–70. doi: 10.1002/j.1460-2075.1994.tb06235.x. [DOI] [PMC free article] [PubMed] [Google Scholar]
- Elion E. A., Brill J. A., Fink G. R. FUS3 represses CLN1 and CLN2 and in concert with KSS1 promotes signal transduction. Proc Natl Acad Sci U S A. 1991 Nov 1;88(21):9392–9396. doi: 10.1073/pnas.88.21.9392. [DOI] [PMC free article] [PubMed] [Google Scholar]
- Elion E. A., Brill J. A., Fink G. R. Functional redundancy in the yeast cell cycle: FUS3 and KSS1 have both overlapping and unique functions. Cold Spring Harb Symp Quant Biol. 1991;56:41–49. doi: 10.1101/sqb.1991.056.01.007. [DOI] [PubMed] [Google Scholar]
- Elion E. A., Grisafi P. L., Fink G. R. FUS3 encodes a cdc2+/CDC28-related kinase required for the transition from mitosis into conjugation. Cell. 1990 Feb 23;60(4):649–664. doi: 10.1016/0092-8674(90)90668-5. [DOI] [PubMed] [Google Scholar]
- Elion E. A., Satterberg B., Kranz J. E. FUS3 phosphorylates multiple components of the mating signal transduction cascade: evidence for STE12 and FAR1. Mol Biol Cell. 1993 May;4(5):495–510. doi: 10.1091/mbc.4.5.495. [DOI] [PMC free article] [PubMed] [Google Scholar]
- Errede B., Gartner A., Zhou Z., Nasmyth K., Ammerer G. MAP kinase-related FUS3 from S. cerevisiae is activated by STE7 in vitro. Nature. 1993 Mar 18;362(6417):261–264. doi: 10.1038/362261a0. [DOI] [PubMed] [Google Scholar]
- Evan G. I., Lewis G. K., Ramsay G., Bishop J. M. Isolation of monoclonal antibodies specific for human c-myc proto-oncogene product. Mol Cell Biol. 1985 Dec;5(12):3610–3616. doi: 10.1128/mcb.5.12.3610. [DOI] [PMC free article] [PubMed] [Google Scholar]
- Gartner A., Nasmyth K., Ammerer G. Signal transduction in Saccharomyces cerevisiae requires tyrosine and threonine phosphorylation of FUS3 and KSS1. Genes Dev. 1992 Jul;6(7):1280–1292. doi: 10.1101/gad.6.7.1280. [DOI] [PubMed] [Google Scholar]
- Gould K. L., Nurse P. Tyrosine phosphorylation of the fission yeast cdc2+ protein kinase regulates entry into mitosis. Nature. 1989 Nov 2;342(6245):39–45. doi: 10.1038/342039a0. [DOI] [PubMed] [Google Scholar]
- Haccard O., Sarcevic B., Lewellyn A., Hartley R., Roy L., Izumi T., Erikson E., Maller J. L. Induction of metaphase arrest in cleaving Xenopus embryos by MAP kinase. Science. 1993 Nov 19;262(5137):1262–1265. doi: 10.1126/science.8235656. [DOI] [PubMed] [Google Scholar]
- Hanks S. K., Quinn A. M., Hunter T. The protein kinase family: conserved features and deduced phylogeny of the catalytic domains. Science. 1988 Jul 1;241(4861):42–52. doi: 10.1126/science.3291115. [DOI] [PubMed] [Google Scholar]
- Hanks S. K., Quinn A. M. Protein kinase catalytic domain sequence database: identification of conserved features of primary structure and classification of family members. Methods Enzymol. 1991;200:38–62. doi: 10.1016/0076-6879(91)00126-h. [DOI] [PubMed] [Google Scholar]
- Hartwell L. H. Mutants of Saccharomyces cerevisiae unresponsive to cell division control by polypeptide mating hormone. J Cell Biol. 1980 Jun;85(3):811–822. doi: 10.1083/jcb.85.3.811. [DOI] [PMC free article] [PubMed] [Google Scholar]
- Herskowitz I. MAP kinase pathways in yeast: for mating and more. Cell. 1995 Jan 27;80(2):187–197. doi: 10.1016/0092-8674(95)90402-6. [DOI] [PubMed] [Google Scholar]
- Hildebrandt E., Fried V. A. Phosphoamino acid analysis of protein immobilized on polyvinylidene difluoride membrane. Anal Biochem. 1989 Mar;177(2):407–412. doi: 10.1016/0003-2697(89)90075-4. [DOI] [PubMed] [Google Scholar]
- Hill J. E., Myers A. M., Koerner T. J., Tzagoloff A. Yeast/E. coli shuttle vectors with multiple unique restriction sites. Yeast. 1986 Sep;2(3):163–167. doi: 10.1002/yea.320020304. [DOI] [PubMed] [Google Scholar]
- Hoffman C. S., Winston F. A ten-minute DNA preparation from yeast efficiently releases autonomous plasmids for transformation of Escherichia coli. Gene. 1987;57(2-3):267–272. doi: 10.1016/0378-1119(87)90131-4. [DOI] [PubMed] [Google Scholar]
- Hsiao K. M., Chou S. Y., Shih S. J., Ferrell J. E., Jr Evidence that inactive p42 mitogen-activated protein kinase and inactive Rsk exist as a heterodimer in vivo. Proc Natl Acad Sci U S A. 1994 Jun 7;91(12):5480–5484. doi: 10.1073/pnas.91.12.5480. [DOI] [PMC free article] [PubMed] [Google Scholar]
- Johnson G. L., Vaillancourt R. R. Sequential protein kinase reactions controlling cell growth and differentiation. Curr Opin Cell Biol. 1994 Apr;6(2):230–238. doi: 10.1016/0955-0674(94)90141-4. [DOI] [PubMed] [Google Scholar]
- Julius D., Blair L., Brake A., Sprague G., Thorner J. Yeast alpha factor is processed from a larger precursor polypeptide: the essential role of a membrane-bound dipeptidyl aminopeptidase. Cell. 1983 Mar;32(3):839–852. doi: 10.1016/0092-8674(83)90070-3. [DOI] [PubMed] [Google Scholar]
- Julius D., Schekman R., Thorner J. Glycosylation and processing of prepro-alpha-factor through the yeast secretory pathway. Cell. 1984 Feb;36(2):309–318. doi: 10.1016/0092-8674(84)90224-1. [DOI] [PubMed] [Google Scholar]
- Knighton D. R., Zheng J. H., Ten Eyck L. F., Ashford V. A., Xuong N. H., Taylor S. S., Sowadski J. M. Crystal structure of the catalytic subunit of cyclic adenosine monophosphate-dependent protein kinase. Science. 1991 Jul 26;253(5018):407–414. doi: 10.1126/science.1862342. [DOI] [PubMed] [Google Scholar]
- Knighton D. R., Zheng J. H., Ten Eyck L. F., Xuong N. H., Taylor S. S., Sowadski J. M. Structure of a peptide inhibitor bound to the catalytic subunit of cyclic adenosine monophosphate-dependent protein kinase. Science. 1991 Jul 26;253(5018):414–420. doi: 10.1126/science.1862343. [DOI] [PubMed] [Google Scholar]
- Koerner T. J., Hill J. E., Myers A. M., Tzagoloff A. High-expression vectors with multiple cloning sites for construction of trpE fusion genes: pATH vectors. Methods Enzymol. 1991;194:477–490. doi: 10.1016/0076-6879(91)94036-c. [DOI] [PubMed] [Google Scholar]
- Kranz J. E., Satterberg B., Elion E. A. The MAP kinase Fus3 associates with and phosphorylates the upstream signaling component Ste5. Genes Dev. 1994 Feb 1;8(3):313–327. doi: 10.1101/gad.8.3.313. [DOI] [PubMed] [Google Scholar]
- Krisak L., Strich R., Winters R. S., Hall J. P., Mallory M. J., Kreitzer D., Tuan R. S., Winter E. SMK1, a developmentally regulated MAP kinase, is required for spore wall assembly in Saccharomyces cerevisiae. Genes Dev. 1994 Sep 15;8(18):2151–2161. doi: 10.1101/gad.8.18.2151. [DOI] [PubMed] [Google Scholar]
- Kuchler K., Dohlman H. G., Thorner J. The a-factor transporter (STE6 gene product) and cell polarity in the yeast Saccharomyces cerevisiae. J Cell Biol. 1993 Mar;120(5):1203–1215. doi: 10.1083/jcb.120.5.1203. [DOI] [PMC free article] [PubMed] [Google Scholar]
- Lackner M. R., Kornfeld K., Miller L. M., Horvitz H. R., Kim S. K. A MAP kinase homolog, mpk-1, is involved in ras-mediated induction of vulval cell fates in Caenorhabditis elegans. Genes Dev. 1994 Jan;8(2):160–173. doi: 10.1101/gad.8.2.160. [DOI] [PubMed] [Google Scholar]
- Laemmli U. K. Cleavage of structural proteins during the assembly of the head of bacteriophage T4. Nature. 1970 Aug 15;227(5259):680–685. doi: 10.1038/227680a0. [DOI] [PubMed] [Google Scholar]
- Leberer E., Dignard D., Harcus D., Thomas D. Y., Whiteway M. The protein kinase homologue Ste20p is required to link the yeast pheromone response G-protein beta gamma subunits to downstream signalling components. EMBO J. 1992 Dec;11(13):4815–4824. doi: 10.1002/j.1460-2075.1992.tb05587.x. [DOI] [PMC free article] [PubMed] [Google Scholar]
- Lee K. S., Irie K., Gotoh Y., Watanabe Y., Araki H., Nishida E., Matsumoto K., Levin D. E. A yeast mitogen-activated protein kinase homolog (Mpk1p) mediates signalling by protein kinase C. Mol Cell Biol. 1993 May;13(5):3067–3075. doi: 10.1128/mcb.13.5.3067. [DOI] [PMC free article] [PubMed] [Google Scholar]
- Lenormand P., Sardet C., Pagès G., L'Allemain G., Brunet A., Pouysségur J. Growth factors induce nuclear translocation of MAP kinases (p42mapk and p44mapk) but not of their activator MAP kinase kinase (p45mapkk) in fibroblasts. J Cell Biol. 1993 Sep;122(5):1079–1088. doi: 10.1083/jcb.122.5.1079. [DOI] [PMC free article] [PubMed] [Google Scholar]
- Marshall C. J. MAP kinase kinase kinase, MAP kinase kinase and MAP kinase. Curr Opin Genet Dev. 1994 Feb;4(1):82–89. doi: 10.1016/0959-437x(94)90095-7. [DOI] [PubMed] [Google Scholar]
- Morla A. O., Draetta G., Beach D., Wang J. Y. Reversible tyrosine phosphorylation of cdc2: dephosphorylation accompanies activation during entry into mitosis. Cell. 1989 Jul 14;58(1):193–203. doi: 10.1016/0092-8674(89)90415-7. [DOI] [PubMed] [Google Scholar]
- Nakamaye K. L., Eckstein F. Inhibition of restriction endonuclease Nci I cleavage by phosphorothioate groups and its application to oligonucleotide-directed mutagenesis. Nucleic Acids Res. 1986 Dec 22;14(24):9679–9698. doi: 10.1093/nar/14.24.9679. [DOI] [PMC free article] [PubMed] [Google Scholar]
- Nebreda A. R. Inactivation of MAP kinases. Trends Biochem Sci. 1994 Jan;19(1):1–2. doi: 10.1016/0968-0004(94)90163-5. [DOI] [PubMed] [Google Scholar]
- Neiman A. M., Herskowitz I. Reconstitution of a yeast protein kinase cascade in vitro: activation of the yeast MEK homologue STE7 by STE11. Proc Natl Acad Sci U S A. 1994 Apr 12;91(8):3398–3402. doi: 10.1073/pnas.91.8.3398. [DOI] [PMC free article] [PubMed] [Google Scholar]
- Nguyen T. T., Scimeca J. C., Filloux C., Peraldi P., Carpentier J. L., Van Obberghen E. Co-regulation of the mitogen-activated protein kinase, extracellular signal-regulated kinase 1, and the 90-kDa ribosomal S6 kinase in PC12 cells. Distinct effects of the neurotrophic factor, nerve growth factor, and the mitogenic factor, epidermal growth factor. J Biol Chem. 1993 May 5;268(13):9803–9810. [PubMed] [Google Scholar]
- Oehlen B., Cross F. R. Signal transduction in the budding yeast Saccharomyces cerevisiae. Curr Opin Cell Biol. 1994 Dec;6(6):836–841. doi: 10.1016/0955-0674(94)90053-1. [DOI] [PubMed] [Google Scholar]
- Payne D. M., Rossomando A. J., Martino P., Erickson A. K., Her J. H., Shabanowitz J., Hunt D. F., Weber M. J., Sturgill T. W. Identification of the regulatory phosphorylation sites in pp42/mitogen-activated protein kinase (MAP kinase). EMBO J. 1991 Apr;10(4):885–892. doi: 10.1002/j.1460-2075.1991.tb08021.x. [DOI] [PMC free article] [PubMed] [Google Scholar]
- Pelech S. L., Sanghera J. S. Mitogen-activated protein kinases: versatile transducers for cell signaling. Trends Biochem Sci. 1992 Jun;17(6):233–238. doi: 10.1016/s0968-0004(00)80005-5. [DOI] [PubMed] [Google Scholar]
- Peter M., Gartner A., Horecka J., Ammerer G., Herskowitz I. FAR1 links the signal transduction pathway to the cell cycle machinery in yeast. Cell. 1993 May 21;73(4):747–760. doi: 10.1016/0092-8674(93)90254-n. [DOI] [PubMed] [Google Scholar]
- Pringle J. R., Adams A. E., Drubin D. G., Haarer B. K. Immunofluorescence methods for yeast. Methods Enzymol. 1991;194:565–602. doi: 10.1016/0076-6879(91)94043-c. [DOI] [PubMed] [Google Scholar]
- Printen J. A., Sprague G. F., Jr Protein-protein interactions in the yeast pheromone response pathway: Ste5p interacts with all members of the MAP kinase cascade. Genetics. 1994 Nov;138(3):609–619. doi: 10.1093/genetics/138.3.609. [DOI] [PMC free article] [PubMed] [Google Scholar]
- Qui M. S., Green S. H. PC12 cell neuronal differentiation is associated with prolonged p21ras activity and consequent prolonged ERK activity. Neuron. 1992 Oct;9(4):705–717. doi: 10.1016/0896-6273(92)90033-a. [DOI] [PubMed] [Google Scholar]
- Ramer S. W., Davis R. W. A dominant truncation allele identifies a gene, STE20, that encodes a putative protein kinase necessary for mating in Saccharomyces cerevisiae. Proc Natl Acad Sci U S A. 1993 Jan 15;90(2):452–456. doi: 10.1073/pnas.90.2.452. [DOI] [PMC free article] [PubMed] [Google Scholar]
- Ramer S. W., Elledge S. J., Davis R. W. Dominant genetics using a yeast genomic library under the control of a strong inducible promoter. Proc Natl Acad Sci U S A. 1992 Dec 1;89(23):11589–11593. doi: 10.1073/pnas.89.23.11589. [DOI] [PMC free article] [PubMed] [Google Scholar]
- Ray L. B., Sturgill T. W. Insulin-stimulated microtubule-associated protein kinase is phosphorylated on tyrosine and threonine in vivo. Proc Natl Acad Sci U S A. 1988 Jun;85(11):3753–3757. doi: 10.1073/pnas.85.11.3753. [DOI] [PMC free article] [PubMed] [Google Scholar]
- Reneke J. E., Blumer K. J., Courchesne W. E., Thorner J. The carboxy-terminal segment of the yeast alpha-factor receptor is a regulatory domain. Cell. 1988 Oct 21;55(2):221–234. doi: 10.1016/0092-8674(88)90045-1. [DOI] [PubMed] [Google Scholar]
- Rhodes N., Connell L., Errede B. STE11 is a protein kinase required for cell-type-specific transcription and signal transduction in yeast. Genes Dev. 1990 Nov;4(11):1862–1874. doi: 10.1101/gad.4.11.1862. [DOI] [PubMed] [Google Scholar]
- Robbins D. J., Cobb M. H. Extracellular signal-regulated kinases 2 autophosphorylates on a subset of peptides phosphorylated in intact cells in response to insulin and nerve growth factor: analysis by peptide mapping. Mol Biol Cell. 1992 Mar;3(3):299–308. doi: 10.1091/mbc.3.3.299. [DOI] [PMC free article] [PubMed] [Google Scholar]
- Robbins D. J., Zhen E., Owaki H., Vanderbilt C. A., Ebert D., Geppert T. D., Cobb M. H. Regulation and properties of extracellular signal-regulated protein kinases 1 and 2 in vitro. J Biol Chem. 1993 Mar 5;268(7):5097–5106. [PubMed] [Google Scholar]
- Roberts R. L., Fink G. R. Elements of a single MAP kinase cascade in Saccharomyces cerevisiae mediate two developmental programs in the same cell type: mating and invasive growth. Genes Dev. 1994 Dec 15;8(24):2974–2985. doi: 10.1101/gad.8.24.2974. [DOI] [PubMed] [Google Scholar]
- Rossomando A. J., Sanghera J. S., Marsden L. A., Weber M. J., Pelech S. L., Sturgill T. W. Biochemical characterization of a family of serine/threonine protein kinases regulated by tyrosine and serine/threonine phosphorylations. J Biol Chem. 1991 Oct 25;266(30):20270–20275. [PubMed] [Google Scholar]
- Scherer S., Davis R. W. Replacement of chromosome segments with altered DNA sequences constructed in vitro. Proc Natl Acad Sci U S A. 1979 Oct;76(10):4951–4955. doi: 10.1073/pnas.76.10.4951. [DOI] [PMC free article] [PubMed] [Google Scholar]
- Seger R., Ahn N. G., Boulton T. G., Yancopoulos G. D., Panayotatos N., Radziejewska E., Ericsson L., Bratlien R. L., Cobb M. H., Krebs E. G. Microtubule-associated protein 2 kinases, ERK1 and ERK2, undergo autophosphorylation on both tyrosine and threonine residues: implications for their mechanism of activation. Proc Natl Acad Sci U S A. 1991 Jul 15;88(14):6142–6146. doi: 10.1073/pnas.88.14.6142. [DOI] [PMC free article] [PubMed] [Google Scholar]
- Sikorski R. S., Hieter P. A system of shuttle vectors and yeast host strains designed for efficient manipulation of DNA in Saccharomyces cerevisiae. Genetics. 1989 May;122(1):19–27. doi: 10.1093/genetics/122.1.19. [DOI] [PMC free article] [PubMed] [Google Scholar]
- Song D., Dolan J. W., Yuan Y. L., Fields S. Pheromone-dependent phosphorylation of the yeast STE12 protein correlates with transcriptional activation. Genes Dev. 1991 May;5(5):741–750. doi: 10.1101/gad.5.5.741. [DOI] [PubMed] [Google Scholar]
- Southern E. M. Detection of specific sequences among DNA fragments separated by gel electrophoresis. J Mol Biol. 1975 Nov 5;98(3):503–517. doi: 10.1016/s0022-2836(75)80083-0. [DOI] [PubMed] [Google Scholar]
- Sprague G. F., Jr Assay of yeast mating reaction. Methods Enzymol. 1991;194:77–93. doi: 10.1016/0076-6879(91)94008-z. [DOI] [PubMed] [Google Scholar]
- Stevenson B. J., Rhodes N., Errede B., Sprague G. F., Jr Constitutive mutants of the protein kinase STE11 activate the yeast pheromone response pathway in the absence of the G protein. Genes Dev. 1992 Jul;6(7):1293–1304. doi: 10.1101/gad.6.7.1293. [DOI] [PubMed] [Google Scholar]
- Struhl K., Stinchcomb D. T., Scherer S., Davis R. W. High-frequency transformation of yeast: autonomous replication of hybrid DNA molecules. Proc Natl Acad Sci U S A. 1979 Mar;76(3):1035–1039. doi: 10.1073/pnas.76.3.1035. [DOI] [PMC free article] [PubMed] [Google Scholar]
- Teague M. A., Chaleff D. T., Errede B. Nucleotide sequence of the yeast regulatory gene STE7 predicts a protein homologous to protein kinases. Proc Natl Acad Sci U S A. 1986 Oct;83(19):7371–7375. doi: 10.1073/pnas.83.19.7371. [DOI] [PMC free article] [PubMed] [Google Scholar]
- Tobe K., Kadowaki T., Tamemoto H., Ueki K., Hara K., Koshio O., Momomura K., Gotoh Y., Nishida E., Akanuma Y. Insulin and 12-O-tetradecanoylphorbol-13-acetate activation of two immunologically distinct myelin basic protein/microtubule-associated protein 2 (MBP/MAP2) kinases via de novo phosphorylation of threonine and tyrosine residues. J Biol Chem. 1991 Dec 25;266(36):24793–24803. [PubMed] [Google Scholar]
- Towbin H., Staehelin T., Gordon J. Electrophoretic transfer of proteins from polyacrylamide gels to nitrocellulose sheets: procedure and some applications. Proc Natl Acad Sci U S A. 1979 Sep;76(9):4350–4354. doi: 10.1073/pnas.76.9.4350. [DOI] [PMC free article] [PubMed] [Google Scholar]
- Traverse S., Gomez N., Paterson H., Marshall C., Cohen P. Sustained activation of the mitogen-activated protein (MAP) kinase cascade may be required for differentiation of PC12 cells. Comparison of the effects of nerve growth factor and epidermal growth factor. Biochem J. 1992 Dec 1;288(Pt 2):351–355. doi: 10.1042/bj2880351. [DOI] [PMC free article] [PubMed] [Google Scholar]
- Tyers M., Futcher B. Far1 and Fus3 link the mating pheromone signal transduction pathway to three G1-phase Cdc28 kinase complexes. Mol Cell Biol. 1993 Sep;13(9):5659–5669. doi: 10.1128/mcb.13.9.5659. [DOI] [PMC free article] [PubMed] [Google Scholar]
- Waskiewicz A. J., Cooper J. A. Evolutionary conservation of Xenopus laevis mitogen-activated protein kinase activation and function. Cell Growth Differ. 1993 Dec;4(12):965–973. [PubMed] [Google Scholar]
- Wu J., Rossomando A. J., Her J. H., Del Vecchio R., Weber M. J., Sturgill T. W. Autophosphorylation in vitro of recombinant 42-kilodalton mitogen-activated protein kinase on tyrosine. Proc Natl Acad Sci U S A. 1991 Nov 1;88(21):9508–9512. doi: 10.1073/pnas.88.21.9508. [DOI] [PMC free article] [PubMed] [Google Scholar]
- Zhang F., Strand A., Robbins D., Cobb M. H., Goldsmith E. J. Atomic structure of the MAP kinase ERK2 at 2.3 A resolution. Nature. 1994 Feb 24;367(6465):704–711. doi: 10.1038/367704a0. [DOI] [PubMed] [Google Scholar]
- Zhou Z., Gartner A., Cade R., Ammerer G., Errede B. Pheromone-induced signal transduction in Saccharomyces cerevisiae requires the sequential function of three protein kinases. Mol Cell Biol. 1993 Apr;13(4):2069–2080. doi: 10.1128/mcb.13.4.2069. [DOI] [PMC free article] [PubMed] [Google Scholar]